Abstract
This study evaluated the effects of plastic mulched ridge-furrow cropping on soil biochemical properties and maize (Zea mays L.) nutrient uptake in a semi-arid environment. Three treatments were evaluated from 2008 to 2010: no mulch (narrow ridges with crop seeded next to ridges), half mulch (as per no mulch, except narrow ridges were mulched), and full mulch (alternate narrow and wide ridges, all mulched with maize seeded in furrows). Compared to the no mulch treatment, full mulch increased maize grain yield by 50% in 2008 and 25% in 2010, but reduced yield by 21% in 2009 after low precipitation in early growth. Half mulch had a similar grain yield to no mulch in the three cropping years, suggesting half mulch is not an effective pattern for maize cropping in the area. Mulch treatments increased aboveground nitrogen (N) uptake by 21−34% and phosphorus (P) uptake by 21−42% in 2008, and by 16−32% and 14−29%, respectively, in 2010; but in 2009 mulching did not affect N uptake and decreased P uptake. Soil microbial biomass and activities of urease, β-glucosidase and phosphatase at the 0−15 cm depth were generally higher during vegetative growth but lower during reproductive growth under mulch treatments than no mulch. Mulching treatments increased carbon (C) loss of buried maize residues (marginally by 5−9%), and decreased light soil organic C (15−27%) and carbohydrate C (12−23%) concentrations and mineralizable C and N (8−36%) at harvest in the 0−20 cm depth compared with no mulch, indicating that mulching promotes mineralization and nutrient release in soil during cropping seasons. As a result of these biological changes, mineral N concentration under mulch was markedly increased after sowing in upper soil layers compared with no mulch. Therefore, our results suggest that mulched cropping stimulated soil microbial activity and N availability, and thus contributed to increasing maize grain yield and nutrient uptake compared with no mulch.
INTRODUCTION
By 2050, the global population is projected to increase by 50% compared to the beginning of this century and global grain demand is projected to double (Tilman et al. Citation2002). Accordingly, if land and water productivity do not improve, crop water consumption will increase by 70−90% (de Fraiture et al. Citation2007), an unaffordable position relative to future world resources (Postel Citation2000). It is not realistic to meet increasing food demands by further extending irrigation areas but it is optimistic to improve productivity in rainfed areas (de Fraiture et al. Citation2007).
Inadequate and unpredictable precipitation and low water availability are major factors limiting agricultural productivity in semi-arid areas (Siddique et al. Citation2001). Plastic-film mulching reduces evaporation and helps use rainwater efficiently, and therefore has been introduced into the production of field crops in those areas (Cook et al. Citation2006; Anikwe et al. Citation2007; Chakraborty et al. Citation2008; Araya and Stroosnijder Citation2010; Kasirajan and Ngouajio Citation2012; Gan et al. Citation2013). Recently, film fully-mulched ridge-furrow cropping, modified from partly-mulched flat land surface, has been widely adopted to facilitate maize (Zea mays L.) production in semi-arid areas of China (Gan et al. Citation2013). Under film fully-mulched ridge-furrow cropping, precipitation on covered ridges is efficiently channeled to furrows where crops are sown and infiltrated into the root zone soil via perforations. In addition, effects of reducing evaporation and soil warming are maximized due to the whole land surface being mulched. As a result, agricultural production has significantly increased in areas where crops with low yielding potential such as wheat (Triticum aestivum L.), potato (Solanum tuberosum L.), pea (Pisum sativum L.), oat (Avena sativa L.), buckwheat (Fagopyrum esculentum Moench.) and millet (Pennisetum glaucum L. R. Br.) traditionally dominated. For example, at a site [altitude 2400 m above sea level (asl), mean annual rainfall 320 mm, temperature 6.5°C] on the Loess Plateau, Zhou et al. (Citation2009) and Liu et al. (Citation2009) reported that by using this cropping pattern, maize grain yield reached 4−5 times that of the unmulched treatment. The increased moisture and temperature in topsoil mainly during early growth supported better grain yields in mulched ridge-furrow systems than in an unmulched system (Liu et al. Citation2009; Zhou et al. Citation2009).
Despite its marked capacity to upgrade cropland productivity, the potential effects of film fully-mulched ridge-furrow cropping on the sustainability of agro-ecosystems are unclear. Soils in semi-arid areas are among those most susceptible to erosion and lowest in soil organic matter content and productivity in China (Catt Citation2001; Chen et al. Citation2003). Soil organic matter contains nutrients, fuels microbiological activities and nutrient cycles, maintains chemical and physical properties, and reduces erosion (Gregorich et al. Citation1994; de la Paz Jimenez et al. Citation2002). Such soil organic matter levels largely determine fertility and productivity of arable soils and the dynamics are associated with the sustainability of agro-ecosystems. The improved soil hydrothermal conditions under film fully-mulched cropping would impact soil microbiological properties. Film mulch has increased soil microbial biomass and activity in wheat (Li et al. Citation2004) and flooded rice (Oryza sativa L.) fields (Li et al. Citation2006). Increased soil mineral nitrogen (N) has been observed in film-mulched wheat cropping (Gao et al. Citation2009). However, despite being widely practiced, there is a lack of knowledge on changes in soil biological properties and nutrient status associated with improved soil temperatures and reduced evaporation under film mulching (Gan et al. Citation2013).
Soil microbes drive the cycling of carbon (C) and nutrients through the regulation of enzyme systems that respond to changes in soil management more quickly than other soil variables (Bandick and Dick Citation1999). β-glucosidase is involved in the last limiting step of cellulose degradation (Acosta-Martínez et al. Citation2007). Urease activity is considered an indicator of N mineralization in soil (Nannipieri and Eldor Citation2009), and phosphatase catalyzes the hydrolysis of a variety of organic and inorganic phosphomonoesters (Acosta-Martínez et al. Citation2007). Therefore, soil microbial biomass and those enzyme activities could be useful measurements for indicating changes in nutrient mineralization and cycling, and decomposition and formation of soil organic matter as affected by film mulching. Crop residue incorporated into soil is more available to microorganisms and thus its decomposition would be more sensitive to changes in soil hydrothermal conditions than native soil organic matter. The light soil organic matter fraction, carbohydrates and mineralizable C are readily affected by soil management practices due to their quick turnover rates (Gregorich et al. Citation1994). Therefore, decomposition of crop residue and changes in those labile soil organic C pools as affected by film mulch would be meaningful for understanding changes in mineralization and nutrient release under film-mulch cropping. In the present study, we determined soil microbial biomass and enzymatic activities, C mineralization, labile organic C pools and N availability in soils under mulch and no mulch treatments. We hypothesized that soil microbial activity would be stimulated and thus soil N availability and nutrient uptake by maize may be enhanced in mulched fields, due to changed hydrothermal conditions compared with no mulch. Our major objective was to evaluate effects of film fully-mulched ridge-furrow cropping on soil microbial activity and mineralizable C and N, which are closely associated with nutrient release and uptake by plants.
MATERIALS AND METHODS
Description of research site
The research site (35°29'N, 107°45'E, 1264 m asl) is situated at Ningxian in Gansu Province on the southern part of the Loess Plateau. The site has a mean annual temperature of 8.7°C, with a maximum monthly temperature of 22.9°C (July) and a minimum of −5.9°C (January). The mean annual precipitation is 565 mm. In the area, three rainfed crops are planted every two years by conventional tillage, irregularly rotated mainly with wheat, maize and soybean (Glycine max L.) or rape (Brassica napus L.). Soils developed from loess sediments are classified as Heilu soils according to the Chinese soil classification system, similar to Mollisols (Soil Survey Staff Citation1975). Soils of the three maize-cultivated years (one field per year) had similar particle-size composition (4% sand, 74% silt, 22% clay) and pH value of 8.1 (soil: water = 1:2.5), with bulk density ranging from 1.14 to 1.16 g cm−3 in the top 20 cm. At sowing, soils in three fields at 0−20 cm depth contained 8.8−8.9 g total organic C, 0.96−1.00 g total N and 0.67−0.79 g total phosphorus (P) per kg soil, with an Olsen P range of 27−35 mg kg−1. The Second National Soil Survey in the 1980s showed that Heilu soils in the area contain 78−84 g carbonates [expressed as calcium carbonate (CaCO3)] per kg soil, with a cation exchange capacity value of 9.8 cmol (+) kg−1. We changed the experimental field in three cultivation years due to farmers’ intentions. However, the three fields were comparable due to their similar soil properties and management practices.
Experimental design and management
Three treatments—no mulch, half mulch and full mulch (A–C)—were arranged in a randomized, complete block design with three replicates. Each of the nine plots was 28.6 m2 (4.4 m × 6.5 m). On the no mulch and half mulch plots, narrow ridges (15 cm high × 40 cm wide) were prepared with an interval space of 70 cm. Maize was sown next to the ridges. The narrow ridges on the half mulch plots were mulched with film (colorless and transparent, 0.008 mm thick). On full mulch plots, narrow ridges (15 cm high × 40 cm wide) were alternated with wide ridges (10 cm high × 70 cm wide), with all the ridges covered by film; crops were seeded in furrows between the narrow and wide ridges. After the soil was covered with film, holes (about 20 cm apart) were made using a handheld device through the film in furrows on the full mulch plots. These holes helped collect and channel water from ridges to the rooting zone when raining. The full mulch is a recent modification (fully-mulched ridge-furrow) from the half mulch pattern and is believed to be more efficient at harvesting rainwater and preventing evaporation than no mulch or half mulch patterns (Liu et al. Citation2009; Zhou et al. Citation2009).
Figure 1 Maize (Zea mays L.) planting patterns for three treatments: (A) no mulch (narrow ridges with flat land between ridges; no mulch); (B) half mulch (narrow ridges covered with mulch but flat land between ridges not covered); (C) full mulch (alternate narrow and wide ridges; all covered with mulch). Maize was seeded next to ridges in no mulch and half mulch treatments and in furrows in full mulch treatment. Within each treatment, soil was sampled along length on narrow ridges.
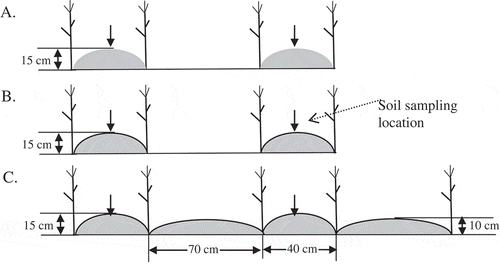
Soil preparation and mulching occurred about 4 weeks before sowing. Prior to setting up ridges, 750 kg superphosphate [containing 12% citric acid soluble phosphorus pentoxide (P2O5)] and 200 kg urea (46% N) per ha were broadcast as basal fertilizer on each plot. Maize (cv. Yuyu in 2008 and 2010 and Funong in 2009, all well adapted to local conditions) was seeded using a handheld device in early March at a density of 52,500 plants per ha (about 30 cm between two plants in rows). At the shooting stage (45−55 d after sowing), 400 kg urea (46% N) per ha was applied in rows to 10 cm deep, between plants using a manually operated device each year. Fertilizer dosages were recommended for maize crops in the area by the Gansu Province Agricultural Technique Extension Station. Maize was harvested in mid-September.
At sowing in 2008 and 2009 growing seasons, two nylon mesh (aperture 1.5 mm) bags each containing 60 g of air-dried maize stalks (length < 20 mm) were separately buried underneath the top 10-cm soil layer of two randomly selected narrow ridges on each plot for assessing soil organic C decomposition. Soybean was cropped prior to each of three maize cropping seasons, with most of the aboveground biomass removed from fields at harvest. Growing season precipitation was 351, 451 and 515 mm in 2008, 2009 and 2010, respectively.
Sampling and measurements
During the 2009 and 2010 growing seasons, soil temperature, soil water, mineral N, microbial biomass and enzymatic activity were measured monthly on narrow ridges in each plot (A–C). Soil temperature was recorded at 5, 10, 15 and 20 cm depths, at 08:00, 14:00 and 20:00 h on three continuous sunny days, for one of the three replicates. Daily soil temperature at 0−20 cm was calculated as the average of three daily readings and four depths. Soils were sampled from the 0−15, 15−30, 30−50, 50−70 and 70−90 cm depths using an auger, randomly on five narrow ridges within a plot. Subsamples at the same depth were pooled to form one composite sample. Soil water content was determined gravimetrically at 105°C. Soil mineral N [nitrate (NO3−) and ammonium (NH4+)] in the five soil layers was extracted with 2 M potassium chloride (KCl) (soil:solution = 1:5, shaken for 30 min on a horizontal shaker) from fresh samples (< 5 mm, equivalent to about 20 g oven-dried weight) and analyzed using a San++ Automated Wet Chemistry Analyzer (Skalar, Breda, Netherlands).
Soil microbial biomass was conducted on fresh soil samples (< 5 mm, equivalent to about 20 g oven-dried weight) from the top 0−15-cm layer. Soil microbial biomass was extracted by a chloroform fumigation-extraction method (Vance et al. Citation1987). Organic C content in filtered extracts was analyzed with a Multi C/N 3100 (Analytik Jena, Jena, Germany). Microbial biomass C was estimated from the difference between organic C concentration in fumigated and non-fumigated extracts and divided by 0.45 (Beck et al. Citation1997).
The activities of β-glucosidase and alkaline phosphatase were assayed by colorimetric determination of p-nitrophenol (PNP) released after soil was incubated with p-nitrophonyl-β-D-glucosidase in pH 6.5 buffer and p-nitrophenyl phosphate in pH 11 buffer for 1 h (37°C), respectively (Tabatabai Citation1982). Fresh soil (< 2 mm) equivalent to 1 g oven-dried weight was weighed into 30-mL glass vials containing 4 mL of buffer and 1 mL of substrate solution, and incubated. After incubation, 1 mL of 0.5-M calcium chloride (CaCl2) and 4 mL of tris buffer pH 12 were added to the β-glucosidase assay, and 1 mL of 0.5-M CaCl2 and 4 mL of 0.5-M sodium hydroxide (NaOH) were added to the alkaline phosphatase assay. Immediately after filtration, the absorbance of enzyme extracts was measured at 400 nm. Activities of β–glucosidase and phosphatase were expressed as mg PNP kg−1 soil h−1. Urease activity was determined as described by Klose and Tabatabai (Citation2000), expressed as mg ammonium nitrogen (NH4+-N) kg−1 soil h−1. Fresh soil (< 2 mm) equivalent to 5 g oven-dried weight was incubated with 9 mL 0.05 M tris buffer (pH 9.0) and 1 mL 0.2 M urea, as the substrate, at 37°C for 2 h, and then 35 mL of KCl and silver sulfate (Ag2SO4) solution was added to stop the reaction. The NH4+ liberated was determined by steam distillation of 20 mL of the soil suspension with magnesium oxide (MgO) for 4 min. All enzyme assays for each sample were duplicated and controls were included.
At harvest, soil C and N mineralization was performed on fresh soil samples (< 2 mm) randomly taken from five narrow ridges in the top 20 cm layer at 25°C in the dark (Li et al. Citation2007b). After adjusting the moisture to 60% water-holding capacity, soil (equivalent to 50 g oven-dried weight) was filled into a small cup. The soil-filled cup was then placed into an enclosed 500-mL jar containing a vial with 10 mL 1-M NaOH for trapping respired carbon dioxide (CO2) and incubated for 10 d. The CO2-trapped vial was replaced with a new vial on the third day of incubation. The amount of carbon dioxide carbon (CO2-C) evolved from soil was determined by titrating the residual NaOH solution with 0.3 M hydro chloric acid (HCl) in the presence of excess barium chloride (BaCl2) to a phenolphthalein end point. Total CO2-C evolved over the 10-d incubation period was defined as mineralizable C (Franzluebbers et al. Citation1994). Before and after incubation, soils were extracted with 2 M KCl (1:5 soil:solution ratio) for analysis of NH4+-N and nitrate nitrogen (NO3–-N). The difference in mineral N (NH4+-N + NO3–-N) concentrations between incubated and non-incubated soils was considered mineralizable N (Franzluebbers et al. Citation1994).
At harvest, air-dried soil samples taken from the top 20 cm on narrow ridges were used to determine light (density < 1.8 g cm−3) soil organic C and carbohydrate C concentrations. The light organic C fraction was separated from 20-g air-dried samples (< 2 mm) according to Marriott and Wander (Citation2006) using sodium iodide (NaI) solution as a density agent, and the C content in the fraction was determined by the Walkley and Black dichromate oxidation method (Nelson and Sommers Citation1982). Carbohydrate C was extracted from 1-g air-dried samples (< 2 mm) using diluted-acid [0.5 M sulphuric acid (H2SO4)], as described by Puget et al. (Citation1999). Carbohydrate concentrations of the filtered extracts were determined by the colorimetric anthrone method at a wavelength of 625 nm (Brink et al. Citation1960), with glucose solutions used as standards.
Buried nylon mesh bags containing maize stalks were taken out from soils and air-dried in the laboratory at room temperature after passing through a 2-mm sieve. The C content of initial and buried maize stalks (ground to fine powders) was analyzed using the Multi C/N 3100 (Analytik Jena, Jena, Germany). C loss of buried maize residue was calculated as the difference in C content within the nylon mesh bags at sowing and harvest.
At maturity, two representative rows of crops per plot were harvested to determine maize grain yield, aboveground biomass and root biomass (constant weight at 60°C). All roots in the two rows were dug using a spade to 30 cm depth with soil washed off in the field. Spikes, leaves, stems and roots were weighed in the field and samples from different maize parts were immediately transported to the laboratory for water content determination. Dried samples from different plant parts were ground to powders and digested in H2SO4 and hydrogen peroxide (H2O2) solution; N and P were determined by the Kjeldahl digestion method and molybdenum-blue colorimetry, respectively (Lu Citation2000). Aboveground N or P uptake is the total nutrient amount in grains, cob cores, and stalks and leaves, and belowground N or P uptake is the total nutrient amount in roots.
Statistics
One-way analysis of variance (ANOVA) was conducted to assess the effects of film-mulch cropping patterns on measured parameters after checking for normality (if not normally distributed then log-transformed). Significant differences between means were detected using Tukey’s honestly significant difference (HSD) test at P ≤ 0.05. The significance of a linear relationship between parameters was expressed by the Pearson’s product moment correlation coefficient. All statistics procedures were performed by SPSS 15.0.
RESULTS
Grain yield, biomass and nutrient uptake
Mulching treatments significantly affected grain yield, aboveground biomass and nutrient (N and P) uptake in each year (, P < 0.001). Compared to the no mulch treatment, full mulch increased maize grain yield by 50% in 2008 and 25% in 2010, but reduced grain yield by 21% in 2009. The half mulch and no mulch treatments had similar grain yields in all three cropping years. Mulch treatments generally increased aboveground biomass in 2009 and 2010 but had no effect in 2008. In contrast, half mulch and full mulch treatments affected root biomass consistently in each of the three experimental years, with increases of 24−39% and 30−58%, respectively, at 0–30 cm depth compared to the no mulch treatment ().
Table 1 Maize (Zea mays L.) grain yield, biomass, and nutrient uptake under different treatments in 2008, 2009 and 2010
N and P concentrations of various plant components at harvest were not affected by mulch (P > 0.05, data not shown); thus, N and P uptake in plant tissues responded to changes in aboveground biomass and roots relating to treatments (). Mulch treatments increased N and P uptake amounts in aboveground biomass by 21−34 and 21−42% in 2008 and by 16−32 and 14−29% in 2010, respectively, but had no effect in 2009. N and P uptake in roots was generally enhanced by mulch treatments, regardless of experimental year ().
Soil temperature and moisture
Half and full mulch treatments increased daily temperature by 1.4−4.6°C at 0−20 cm soil depth during vegetative growth but not during reproductive growth, compared with no mulch (A and B). Soil water content did not increase in mulched treatments in various layers during 2009 and 2010 growth seasons compared with the no mulch treatment; in fact, soil water content even decreased at some stages (A−F).
Soil microbial properties
Soil microbial biomass and activities of β-glucosidase, urease and phosphatase at 0−15 cm depth after sowing were generally higher during vegetative growth but lower at harvest or during reproductive growth under mulch compared with no mulch (A–H). Correlation analysis showed that soil microbial biomass C concentration over one growing season was closely related to the activities of β-glucosidase (r = 0.73 in 2009 and 0.88 in 2010, P < 0.001) and urease (r = 0.73 and 0.91, P < 0.001) or phosphatase (r = 0.76 and 0.86, P < 0.001).
Figure 4 Dynamics of (A, E) soil microbial biomass carbon (MBC), (B, F) ß-glucosidase activity, (C, G) urea activity and (D, H) alkaline phosphatase activity in the 0–15 cm layer under three mulch treatments in 2009 and 2010. Significant differences in means (n = 3) are indicated by different letters at P ≤ 0.05 between treatments within each determination. C, carbon; PNP, p-nitrophenol.ß; NH4+, ammonium.
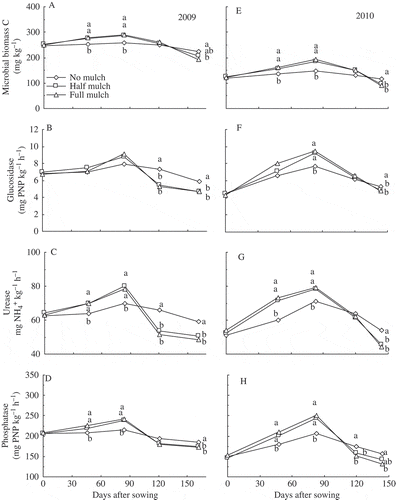
By harvest in 2008 and 2009, C loss of maize residues buried beneath the top 10-cm soil layer marginally increased by 5–9% under mulch compared with no mulch (). At harvest, light organic C concentration in the top 20-cm soil layer was not affected by mulching in 2008 but decreased by 15–27% in 2009 and 2010 (A). Carbohydrate concentration decreased by 12–23% (B) and mineralizable C by 8–21% (C) over three experimental years under mulch compared to no mulch.
Figure 5 Percent carbon loss of maize (Zea mays L.) residues in bags buried beneath the top 10-cm soil layer for different mulch treatments during 2008 and 2009 cropping seasons. Different letters indicate means differ at P ≤ 0.05 between treatments. Bars on columns are standard errors (n = 3). C, carbon.
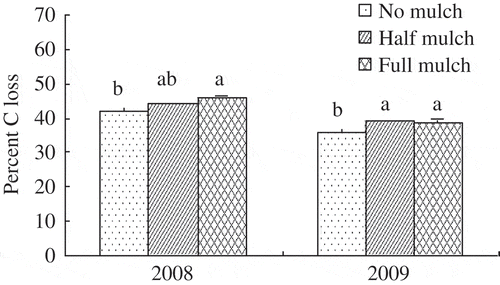
Figure 6 Concentrations of (A) light organic carbon (C), (B) carbohydrate C, (C) mineralizable C and (D) mineralizable nitrogen (N) in the top 20-cm soil layer at harvest in 2008, 2009 and 2010. Different letters indicate means differ at P ≤ 0.05 between treatments. Bars on columns are standard errors (n = 3).
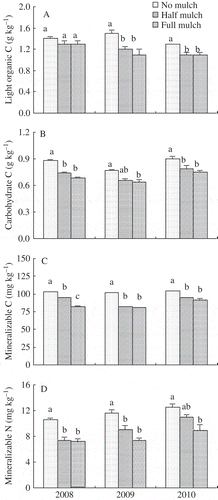
Soil mineral nitrogen
Mulching treatments significantly increased soil mineral N (NO3− − N + NH4+ − N) content after sowing, and mineral N residue at harvest was significantly higher at 0−15 cm depth under mulch compared with no mulch (A–C). In particular, mineral N content in mulched soils was 5−11 times that in unmulched soils at harvest. However, mulching significantly lowered mineralizable N by 13−36% at harvest in three experimental years at 0−20 cm soil depth compared with no mulch at harvest (D).
Figure 7 Dynamics of soil mineral nitrogen (N) [nitrate nitrogen + ammonium nitrogen (NO3−-N + NH4+-N)] contents at 0–15 cm depth under three treatments in (A) 2009 and (B) 2010 cropping seasons. Significant differences in means (n = 3) are indicated by different letters at P ≤ 0.05 between treatments within each determination.
![Figure 7 Dynamics of soil mineral nitrogen (N) [nitrate nitrogen + ammonium nitrogen (NO3−-N + NH4+-N)] contents at 0–15 cm depth under three treatments in (A) 2009 and (B) 2010 cropping seasons. Significant differences in means (n = 3) are indicated by different letters at P ≤ 0.05 between treatments within each determination.](/cms/asset/620f7b42-7523-4a08-9b41-9a9adaaa4435/tssp_a_909709_f0007_b.gif)
DISCUSSION
Soil temperature and moisture
Film mulch increases soil temperature by reducing heat exchange between the soil and air (Liu et al. Citation2010). In the present study, higher soil temperatures in mulched soils than unmulched soil during early but not later growth was possibly because the soil surface was exposed to direct solar radiation during early growth while it was mostly shadowed by a well-established canopy in later growth. Increased soil temperatures from mulching presuppose the acceptance of solar wave radiation (Tarara Citation2000). Film mulch prevents evaporation of water from the soil surface (Lament Citation1993; Li et al. Citation1999; Tian et al. Citation2003; Liu et al. Citation2009). Therefore, film-mulch cropping is usually reported to increase soil water content during the growing season (e.g., Li et al. Citation2001, Citation2007a; Gan et al. Citation2013). However, in the present study, we did not detect any significant increases in soil water content in mulched treatments over three experimental years compared with the unmulched treatment. It is possible that our limited measurements may have missed temporary increases in soil moisture in mulched soils after rain; that is, mulch-conserved rainwater in soil had already been allocated for transpiration by the time of measurement. This suggests that maize crops transpire more water under mulch than no mulch. In fact, soil water content even decreased 60−90 d after sowing in mulched treatments compared with the unmulched treatment, as also reported by Zhou et al. (Citation2009) at another site in China. This indicates that maize has a greater capacity to use moisture in deeper layers under mulch than under no mulch. The increased transpiration and water use capacity of maize crops under mulch could be supported by their faster growth (data not shown) and greater aboveground and root biomasses compared with no mulch. Water uptake and transpiration have been linked to root biomass and canopy size (Poorter and Nagel Citation2000).
Soil microbial activity and mineralization
The present study demonstrated that film mulch accelerated soil microbial activity and C mineralization in maize fields, as evidenced by increased microbial biomass, enzyme activities and loss of buried maize residues and the reduction in various labile soil organic C pools during the cropping season in mulched soils compared to unmulched soil. The increased C mineralization associated with stimulated enzyme activities in mulched soils suggests a stimulatory nutrient release from soil organic matter (Koerselman et al. Citation1993). On the other hand, it indicates enhanced C output through mineralization in mulched soils relative to unmulched soil.
The increased temperature promoted growth and proliferation of soil microorganisms, which led to increased soil microbial biomass and thus assimilative and respiratory utilization of labile soil organic matter pools (i.e., light, carbohydrate and mineralizable C pools) during vegetative growth under mulch compared with no mulch. Plastic mulching increased microbial biomass in a film-mulched wheat field (Li et al. Citation2004) and soil warming increased labile organic C mineralization in a natural ecosystem (Frey et al. Citation2008). The promoted mineralization led to more readily respirable substrates being decomposed during vegetative growth and thus less being left in soil at harvest under mulch treatments than under no mulch. Therefore, due to insufficient feeding of available substrates, soil microbial biomass was suppressed during the late maize growth period in mulched soils compared with unmulched soil. Growth of soil microorganisms relies on substrate abundance and availability (Fontaine et al. Citation2003; Blagodatskaya et al. Citation2007). Prior to being catabolized and anabolized by soil micoorganisms, enzymes catalyze polymers of soil organic matter to extracellularly break down to smaller compounds (Burns and Wallenstein Citation2010). Accordingly, bound to the promoted mineralization, activities of urease, β-glucosidase and phosphatase generally increased under mulch cropping in the present study. The stimulated microbial community size enhanced enzyme production in soil during vegetative growth, but the supressed microbial community size reduced enzyme secretion in late maize growth under mulching. This conjecture was confirmed by similar trends in dynamics and close correlations between soil enzyme activities and microbial biomass under different treatments.
Soil nitrogen availability
The significantly higher mineral N concentration in mulched soils throughout cropping seasons suggests increased N availability compared to unmulched soil. Firstly, N mineralization in mulched soils was stimulated due to increased soil temperature during vegetative growth. This was evidenced by increased urease activity during vegetative growth and decreased mineralizable N at harvest in the mulched soils relative to unmulched soil. Urease activity is considered an indicator of N mineralization in soil (Nannipieri and Eldor Citation2009); more organic N was transformed into mineral N during vegetative growth, with less left to be mineralized at harvest in mulched soils than unmulched soil. N mineralization has been reported to be stimulated by increasing soil temperature (Piatek and Allen Citation1999; Pregitzer et al. Citation2000; Guntiñas et al. Citation2012). Secondly, ammonia volatilization was presumably prohibited by the physical film barrier. N volatilization is a major pathway for N loss in the agroecosystem on the Loess Plateau of China (Roelcke et al. Citation2002; Ju et al. Citation2009). However, further research on the mechanisms for increased mineral N concentrations under mulch compared with no mulch during growth is needed for film-mulched maize cropping.
Yield, biomass and nutrient uptake
Mulch increased soil temperatures and reduced evaporation, and thus improved the N availability associated with higher soil microbial activity. Such improvement under mulch may contribute to increasing maize growth, biomass production and nutrient uptake compared with no mulch. Increasing soil temperature effects on growth and nutrient uptake by plants have been reported by others (Mackay and Barber Citation1984; BassiriRad et al. Citation1993; BassiriRad Citation2000). The increased magnitude of grain yield under full mulch in 2008 and 2010 in the present study was comparable to that reported by Liu et al. (Citation2010) and Zhang et al. (Citation2011) at a similar site, but much lower than that reported by Zhou et al. (Citation2009) and Liu et al. (Citation2009) at another drier and cooler site. This might suggest that the less limited the heat and rainfall, the less significant the full mulch effect on maize grain yield. Increased nutrient uptakes under full mulch indicate enhanced nutrient use efficiency of maize. The similar grain yields in the half mulch and no mulch treatments over the 3 years suggest that half mulch is not an effective pattern for maize cropping in the area, possibly due to a lower efficiency in reducing soil evaporation. Increased maize root production with mulching suggests that film-mulch cropping increases organic C input to the soil, offering a component to help offset the negative effect of stimulated C mineralization on the total soil organic C pool.
We exclude the possibility that different cultivars caused the reduction in grain yield under mulch compared with no mulch in 2009, because the cultivars Yuyu (in 2008 and 2010) and Funong (in 2009) are all well adapted for a normal climate and both cultivars followed a similar yield trend in a given year in neighboring fields. We also exclude another possibility that different mineral N status in soil resulted in the reduction in grain yield in the mulched treatments compared with the unmulched treatment in 2009; in 2009 soil mineral N at 0−15 cm was 47−50 kg N ha−1 for all treatments at sowing, which was comparable with that (52−57 kg N ha−1) in 2008. The reduction in grain yield in 2009 under mulches relative to no mulch was possibly due to less precipitation than other years during early growth, causing more severe water stress on spike development and flowering in mulched crops than unmulched crops. We calculated rainfall distribution over maize growth periods for the three experimental years (A–C). Rainfall was unusually low in late spring and early summer 2009. From day 61−90 after sowing, rainfall was 107 mm in 2008 and 38 mm in 2010, but only 17 mm in 2009; while from day 0−90 after sowing, rainfall was 138 mm in 2008 and 145 mm in 2010, and only 86 mm in 2009. Accordingly, soil water content at 0−90 cm depth was lower throughout the 2009 growing season than in 2010. In particular, the measured soil water content (around 5−6%) at 0−15 and 15−30 cm depth at day 84 after sowing in 2009 was equivalent to permanent wilting point. On the other hand, spike development and flowering stages in maize under mulch largely occurred within this dry soil period due to faster growth. In contrast, maize growth was slower under no mulch, with spike development and flowering stages partly occurring outside this dry period. Reproductive growth in maize is more sensitive to soil moisture than vegetative growth (Igbadun et al. Citation2007). Therefore, considerably less rainfall during early growth would have failed film-mulched furrow-ridge cropping in 2009. Based on our results, film-mulch cropping may decrease maize production when rainfall is too low during early growth. If long-term weather forecasts were available, mulching before the previous winter to save more water in soil (Liu et al. Citation2009) or delaying sowing would avoid such risks with maize production under film mulch in this area.
CONCLUSIONS
The present study suggested that in addition to increased soil temperature and reduced evaporation, stimulated soil microbial activity and the resultant increased soil N availability under mulch contributed to increased maize grain yield, biomass production and nutrient uptake compared with no mulch. When water availability is low during flowering and spike development, film-mulch cropping may decrease maize grain yield due to more severe water stress on developing reproductive organs compared with no mulch.
ACKNOWLEDGMENTS
The study was financed by The State Technology Support Program (2011BAD29B04).
REFERENCES
- Acosta-Martínez V, Cruz L, Sotomayor-Ramírez D, Pérez-Alegría L 2007: Enzyme activities as affected by soil properties and land use in a tropical watershed. Appl. Soil Ecol., 35, 35–45. doi:10.1016/j.apsoil.2006.05.012
- Anikwe MAN, Mbah CN, Ezeaku PI, Onyia VN 2007: Tillage and plastic mulch effects on soil properties and growth and yield of cocoyam (Colocasia esculenta) on an ultisol in southeastern Nigeria. Soil Till. Res., 93, 264–272. doi:10.1016/j.still.2006.04.007
- Araya A, Stroosnijder L 2010: Effects of tied ridges and mulch on barley (Hordeum vulgare) rainwater use efficiency and production in Northern Ethiopia. Agric. Water Manag., 97, 841–847. doi:10.1016/j.agwat.2010.01.012
- Bandick AK, Dick RP 1999: Field management effects on soil enzyme activities. Soil Biol. Biochem., 31, 1471–1479. doi:10.1016/S0038-0717(99)00051-6
- BassiriRad H 2000: Kinetics of nutrient uptake by roots: Responses to global change. New Phytol., 147, 155–169. doi:10.1046/j.1469-8137.2000.00682.x
- BassiriRad H, Caldwell MM, Bilbrough C 1993: Effects of soil temperature and nitrogen status on kinetics of 15NO3– uptake by roots of field-grown Agropyron desertorum (Fisch. Ex Link) Schult. New Phytol., 123, 485–489. doi:10.1111/j.1469-8137.1993.tb03760.x
- Beck T, Joergensen RG, Kandeler E, Makeschin F, Nuss E, Oberholzer HR, Scheu S 1997: An inter-laboratory comparison of ten different ways of measuring soil microbial biomass C. Soil Biol. Biochem., 29, 1023–1032. doi:10.1016/S0038-0717(97)00030-8
- Blagodatskaya EV, Blagodatsky SA, Anderson T-H, Kuzyakov Y 2007: Priming effects in Chernozem induced by glucose and N in relation to microbial growth strategies. Appl. Soil Ecol., 37, 95–105. doi:10.1016/j.apsoil.2007.05.002
- Brink RH, DUBACH P, Lynch DL 1960: Measurement of carbohydrates in soil hydrolyzates with anthrone. Soil Sci. , 89, 157–166. doi:10.1097/00010694-196003000-00006
- Burns RG, Wallenstein MD 2010: Microbial extracellular enzymes and natural and synthetic polymer degradation in soil: Current research and future prospects. In Proceedings of the 19th World Congress of Soil Science: Soil Solutions for a Changing World, Eds. Gilkes R, Prakongkep N, pp. 67–69. Brisbane, Australia.
- Catt JA 2001: The agricultural importance of loess. Earth Sci. Rev., 54, 213–229. doi:10.1016/S0012-8252(01)00049-6
- Chakraborty D, Nagarajan S, Aggarwal P, Gupta VK, Tomar RK, Garg RN, Sahoo RN, Sarkar A, Chopra UK, Sarma KS, Kalra N 2008: Effect of mulching on soil and plant water status, and the growth and yield of wheat (Triticum aestivum L.) in a semi-arid environment. Agric. Water Manag., 95, 1323–1334. doi:10.1016/j.agwat.2008.06.001
- Chen L, Messing I, Zhang S, Fu B, Ledin S 2003: Land use evaluation and scenario analysis towards sustainable planning on the Loess Plateau in China—case study in a small catchment. Catena, 54, 303–316. doi:10.1016/S0341-8162(03)00071-7
- Cook HF, Valdes GSB, Lee HC 2006: Mulch effects on rainfall interception, soil physical characteristics and temperature under Zea mays L. Soil Tillage Res., 91, 227–235. doi:10.1016/j.still.2005.12.007
- de Fraiture C, Wichelns D, Rockstrm J, Kemp-Benedict E, Eriyagama N, Gordon LJ, Hanjra MA, Hoogeveen J, Huber-Lee A, Karlberg L 2007: Looking ahead to 2050: Scenarios of alternative investment approaches. In Water for Food, Water for Life: A Comprehensive Assessment of Water Management in Agriculture, Ed. Molden D, pp. 91–145. International Water Management Institute, London.
- de la Paz Jimenez M, de la Horra AM, Pruzzo L, Palma RM 2002: Soil quality: A new index based on microbiological and biochemical parameters. Biol. Fertil. Soils, 35, 302–306. doi:10.1007/s00374-002-0450-z
- Fontaine S, Mariotti A, Abbadie L 2003: The priming effect of organic matter: a question of microbial competition? Soil Biol. Biochem., 35, 837–843. doi:10.1016/S0038-0717(03)00123-8
- Franzluebbers AJ, Hons FM, Zuberer DA 1994: Seasonal changes in soil microbial biomass and mineralizable C and N in wheat management systems. Soil Biol. Biochem., 26, 1469–1475. doi:10.1016/0038-0717(94)90086-8
- Frey SD, Drijber R, Smith H, Melilloet J 2008: Microbial biomass, functional capacity, and community structure after 12 years of soil warming. Soil Biol. Biochem., 40, 2904–2907. doi:10.1016/j.soilbio.2008.07.020
- Gan Y, Siddique KHM, Turner NC, Li X-G, Niu J-Y, Yang C, Liu L, Chai, Q. 2013: Ridge-furrow mulching systems—An innovative technique for boosting crop productivity in semiarid rain-fed environments. Adv. Agron, 118, 429–476. doi:10.1016/B978-0-12-405942-9.00007-4
- Gao Y, Li Y, Zhang J, Liu W, Dang Z, Cao W, Qiang Q 2009: Effects of mulch, N fertilizer, and plant density on wheat yield, wheat nitrogen uptake, and residual soil nitrate in a dryland area of China. Nutr. Cycl. Agroecosys., 85, 109–121. doi:10.1007/s10705-009-9252-0
- Gregorich EG, Monreal CM, Carter MR, Angers DA, Ellert BH 1994: Towards a minimum data set to assess soil organic matter quality in agricultural soils. Can. J. Soil Sci., 74, 367–385. doi:10.4141/cjss94-051
- Guntiñas ME, Leirós MC, Trasar-Ceped C, Gil-Sotres F 2012: Effects of moisture and temperature on net soil nitrogen mineralization: A laboratory study. Eur. J. Soil Biol., 48, 73–80. doi:10.1016/j.ejsobi.2011.07.015
- Igbadun HE, Tarimo AKPR, Salim BA, Mahoo HF 2007: Evaluation of selected crop water production functions for an irrigated maize crop. Agric. Water Manage., 94, 1–10. doi:10.1016/j.agwat.2007.07.006
- Ju XT, Xing GX, Chen XP, Zhang SL, Zhang LJ, Liu XJ, Cui ZL, Yin B, Christie P, Zhu ZL, Zhang FS 2009: Reducing environmental risk by improving N management in intensive Chinese agricultural systems. Proc. Natl. Acad. Sci., 106, 3041–3046. doi:10.1073/pnas.0813417106
- Kasirajan S, Ngouajio M 2012: Polyethylene and biodegradable mulches for agricultural applications: A review. Agron. Sustain. Dev., 32, 501–529. doi:10.1007/s13593-011-0068-3
- Klose S, Tabatabai MA 2000: Urease activity of microbial biomass in soils as affected by cropping systems. Biol. Fertil. Soils, 31, 191–199. doi:10.1007/s003740050645
- Koerselman W, van Kerkhoven MB, Verhoeven JTA 1993: Release of inorganic N, P and K in peat soils; effect of temperature, water chemistry and water level. Biogeochemistry, 20, 63–81. doi:10.1007/BF00004135
- Lament WJ 1993: Plastic mulches for the production of vegetable crops. HortTechnology, 3, 35–39.
- Li FM, Guo AH, Wei H 1999: Effects of clear plastic film mulch on yield of spring wheat. Field Crops Res., 63, 79–86. doi:10.1016/S0378-4290(99)00027-1
- Li FM, Song QH, Jjemba PK, Shi Y, Shi YC 2004: Dynamics of soil microbial biomass C and soil fertility in cropland mulched with plastic film in a semiarid agro-ecosystem. Soil Biol. Biochem., 36, 1893–1902. doi:10.1016/j.soilbio.2004.04.040
- Li X, Su D, Yuan Q 2007a: Ridge-furrow planting of alfalfa (Medicago sativa L.) for improved rainwater harvest in rainfed semiarid areas in Northwest China. Soil Till. Res., 93, 117–125. doi:10.1016/j.still.2006.03.022
- Li XG, Rengel Z, Mapfumo E, Singh B 2007b: Increase in pH stimulates mineralization of “native” organic carbon and nitrogen in naturally salt-affected sandy soils. Plant Soil, 290, 269–282. doi:10.1007/s11104-006-9158-4
- Li XY, Gong JD, Gao QZ, Li FR 2001: Incorporation of ridge and furrow method of rainfall harvesting with mulching for crop production under semiarid conditions. Agric. Water Manag., 50, 173–183. doi:10.1016/S0378-3774(01)00105-6
- Li YS, Wu LH, Lu XH, Zhao LM, Fan QL, Zhang FS 2006: Soil microbial biomass as affected by non-flooded plastic mulching cultivation in rice. Biol. Fertil. Soils, 43, 107–111. doi:10.1007/s00374-005-0069-y
- Liu CA, Jin SL, Zhou LM, Jia Y, Li FM, Xiong YC, Li XG 2009: Effects of plastic film mulch and tillage on maize productivity and soil parameters. Eur. J. Agron. , 31, 241–249. doi:10.1016/j.eja.2009.08.004
- Liu Y, Yang S, Li S, Chen X, Chen F 2010: Growth and development of maize (Zea mays L.) in response to different field water management practices: Resource capture and use efficiency. Agr. Forest Meteorol., 150, 606–613. doi:10.1016/j.agrformet.2010.02.003
- Lu RK 2000: Soil and Agrochemistry Analysis. China Agricultural Socio-technological Press, Beijing.
- Mackay AD, Barber SA 1984: Soil temperature effects on root growth and phosphorus uptake by corn. Soil Sci. Soc. Am. J., 48(4), 818–823. doi:10.2136/sssaj1984.03615995004800040024x
- Marriott EE, Wander M 2006: Qualitative and quantitative differences in particulate organic matter fractions in organic and conventional farming systems. Soil Biol. Biochem., 38, 1527–1536. doi:10.1016/j.soilbio.2005.11.009
- Nannipieri P, Eldor P 2009: The chemical and functional characterization of soil N and its biotic components. Soil Biol. Biochem., 41, 2357–2369. doi:10.1016/j.soilbio.2009.07.013
- Nelson DW, Sommers LE 1982: Total carbon, organic carbon and organic matter. In Methods of Soil Analysis, Part 2, Chemical and Microbiological Properties. Agronomy Monograph No. 9, 2nd edition, Eds. Page AL, Miller RH, Keeney DR, pp. 539–577. American Society of Agronomy Inc, Madison, WI.
- Piatek KB, Allen HL 1999: Nitrogen mineralization in a pine plantation fifteen years after harvesting and site preparation. Soil Sci. Soc. Am. J., 63, 990–998. doi:10.2136/sssaj1999.634990x
- Poorter H, Nagel O 2000: The role of biomass allocation in the growth response of plants to different levels of light, CO2, nutrients and water: A quantitative review. Aust. J. Plant Physiol., 27, 1191–1191. doi:10.1071/PP99173_CO
- Postel SL 2000: Entering an era of water scarcity: The challenges ahead. Ecological Appl., 10, 941–948. doi:10.1890/1051-0761(2000)010[0941:EAEOWS]2.0.CO;2
- Pregitzer KS, King JS, Burton AJ, Brown SE 2000: Responses of tree fine roots to temperature. New Phytol., 147, 105–115. doi:10.1046/j.1469-8137.2000.00689.x
- Puget P, Angers DA, Chenu C 1999: Nature of carbohydrates associated with water-stable aggregates of two cultivated soils. Soil Biol. Biochem., 31, 55–63. doi:10.1016/S0038-0717(98)00103-5
- Roelcke M, Li SX, Tian XH, Gao YJ, Richter J 2002: In situ comparisons of ammonia volatilization from N fertilizers in Chinese loess soils. Nutr. Cycl. Agroecosys., 62, 73–88. doi:10.1023/A:1015186605419
- Siddique KHM, Regan KL, Tennant D, Thomson BD 2001: Water use and water use efficiency of cool season grain legumes in low rainfall Mediterranean – Type environments. Eur. J. Agron., 15, 267–280. doi:10.1016/S1161-0301(01)00106-X
- Soil Survey Staff 1975: Soil Taxonomy, A Basic System of Soil Classification for Making and Interpreting Soil Surveys. US Government Printing Office, Washington, DC.
- Tabatabai MA 1982: Soil enzymes. In Methods of Soil Analysis, Part 2 Chemical and microbiological properties, 2nd edition, Eds. Page AL, Miller RH, Keeney DR, pp. 903–943. American Society of Agronomy, Madison.
- Tarara JM 2000: Microclimate modification with plastic mulch. HortScience, 35, 169–180.
- Tian Y, Su DR, Li FM, Li XL 2003: Effect of rainwater harvesting with ridge and furrow on yield of potato in semiarid areas. Field Crops Res., 84, 385–391. doi:10.1016/S0378-4290(03)00118-7
- Tilman D, Cassman KG, Matson PA, Naylor R, Polasky S 2002: Agricultural sustainability and intensive production practices. Nature, 418, 671–677. doi:10.1038/nature01014
- Vance ED, Brookes PC, Jenkinson DS 1987: An extraction method for measuring soil microbial biomass-C. Soil Biol. Biochem., 19, 703–707. doi:10.1016/0038-0717(87)90052-6
- Zhang F, Cui Z, Fan M, Zhang W, Chen X, Jiang R. 2011: Integrated soil-crop system management: Reducing environmental risk while increasing crop productivity and improving nutrient use efficiency in China. J. Environ. Qual., 40, 1051–1057. doi:10.2134/jeq2010.0292
- Zhou LM, Li FM, Jin SL, Song Y 2009: How two ridges and the furrow mulched with plastic film affect soil water, soil temperature and yield of maize on the semiarid Loess Plateau of China. Field Crops Res., 113, 41–47. doi:10.1016/j.fcr.2009.04.005