Abstract
Aluminum (Al) and nutrients are key factors to influence tea (Camellia sinensis L.) productivity and quality, while how they interplay in tea plantations under the pressure of global change and increasing fertilization is little studied. In this study, we selected the tea plantations along an age-chronosequence to study Al fractions using a sequential extraction procedure, and nutrient concentrations in topsoil and subsoil and various plant organs. Our results indicated that Al levels and nutrient concentrations in soils and plants generally increased with planting year (P < 0.05), and soil Al bioavailability was positively correlated with Al concentrations in most plant organs. Significant negative relations among pH and most extractable Al fractions in both soil layers suggested that decreased pH would directly alter soil-plant Al cycling due to exogenous nitrogen (N) fertilizer and atmospheric acid deposition. Topsoil total phosphorus (P) was positively correlated with most Al fractions, and root P was positively correlated with root Al concentration, both of which indicate that P and Al were synchronously absorbed by roots in acid tea soils. In addition, topsoil organic carbon was positively correlated with both active and inert Al fractions, indicating that above-ground organic litters would be the main source of elevated Al levels in older tea plantations. Clearly, Al enrichment in tea leaves with increasing planting year needs to be considered under management practices with heavy N and P fertilizers and increasing atmospheric acid deposition in subtropical China.
1. INTRODUCTION
Human activity has altered many element cycles, and their individual biogeochemical cycles are in general well understood (Vitousek et al. Citation1997; Falkowski et al. Citation2000; Hayashi and Yan Citation2010). However, little quantitative information exists on the interactions among various elemental cycles in terrestrial ecosystems (Marklein and Houlton Citation2012). The interplay between aluminum (Al) and nutrients in tea (Camellia sinensis L.) plantations is of scientific and social interest (Ruan et al. Citation2006) since (1) tea plants are an Al-accumulating shrub (Dong et al. Citation2001; Tolrà et al. Citation2011) and commercially available tea contains large amounts of Al. A further 30–40% of Al could be absorbed into infusions and thus absorbed by tea drinkers (Flaten Citation2002); (2) a potential link between high Al contents in tissues and various neuro-degenerative disorders such as Alzheimer’s disease has been reported (Nayak Citation2002); (3) soil pH and nutrients have been altered by atmospheric deposition with global environmental changes and fertilization due to productivity maintenance (Ruan et al. Citation2000; Chen et al. Citation2006; Wang et al. Citation2010), this has affected the Al cycling in tea plantations (Fung and Wong Citation2002; Ruan et al. Citation2004; Fung et al. Citation2008; Duan et al. Citation2012) and vice versa; for example, soil available Al promotes plants to absorb phosphate (Konishi et al. Citation1985; Dong et al. Citation2001; Debnath et al. Citation2011).
Tea plantations are important cash plantations in the world, for tea is one of the three most commonly consumed beverages (coffee, tea and cocoa) worldwide. Currently, there are 3 million ha of tea plantations, globally (Xue et al. Citation2013). In subtropical China, most of the tea-growing areas are acidic-leached red soil (Ultisols) with poor amounts of available nutrients (Han et al. Citation2007; Debnath et al. Citation2011; Han et al. Citation2012). And soil Al availability, in forms such as soluble Al, exchangeable Al and organic Al complexes, has increased with an increased acidic environment in this area. This comes from fertilization practices and environmental changes (Xie et al. Citation2001; Ruan et al. Citation2004; Duan et al. Citation2012). The growth of tea plants as one of the most Al-tolerant species can be markedly stimulated by relatively Al-rich supply (Ghanati et al. Citation2005; Fung et al. Citation2008). Fung et al. (Citation2008) reported that the biomass of tea seedlings increased by 44% and 35%, when grown in solutions of 0.25 and 0.5 mM Al concentrations, respectively, compared with the absence of Al. On the other hand, it is a common and effective fertilization approach to include nitrogen (N) and phosphorus (P) additions to increase the yield and quality of tea (Bajaj et al. Citation1984; Ruan et al. Citation2000). It is also reported that soil Al levels and nutrient status generally increased, but soil quality and biological activity decreased with increasing age of tea plantations, due to unreasonable management practices (Dang Citation2007; Han et al. Citation2007).
If true, the fate and consequence of Al and nutrient accumulations in tea plantations needs to be thoroughly investigated. Al and nutrients can be cycled from soil to plants and back to soil (Rengel Citation2004; Ruan et al. Citation2006), e.g. taken up by roots/mycorrhizae from the soil, internally cycled in the tea plant and then eventually deposited back to the soil via organic litter production (Ruan et al. Citation2004). Al and nutrients can also be internally cycled within the soil in many different ways, e.g. from litter decay to soil solution or from soil solution into the microbial community (Fung and Wong Citation2002). Finally, Al and nutrients can be cycled within tea plants through remobilization from one plant organ to another, for example, in the re-translocation of Al and nutrients from senescing leaves to storage in woody tissues in autumn and the redeployment of the stored Al and nutrients to developing shoots in spring (Ruan et al. Citation2006). Al that is not stored or cycled within the plant-soil system must ultimately be transferred to some other ecosystem through harvest, leaching, erosion, herbivory and other processes (Rengel Citation2004). Therefore, understanding the dynamic of Al and nutrient pools in the plant-soil system with increasing plantation age is necessary to understand the biogeochemical cycling in tea plantations.
In this study, we selected seven different age grades (non-tea plantation, below 10 years old, 10–20 years old, 20–30 years old, 30–40 years old, 40–50 years old and above 50 years old) of tea plantations within a hilly red soil farm, in subtropical China. Soils at the 0–20 cm (topsoil) and 20–40 cm layers (subsoil) and plant organs (roots, stems, twigs, mature leaves and new leaves) were sampled in each of the 19 sites. Our objectives were to identify the Al levels (total content and the fractions), chemical properties (pH and cation exchange capacity) and nutrients [organic carbon (C), total N and P] across an age-chronosequence of traditional tea plantations, and to analyze the linkages between Al fractions and nutrients in soils and plants. Finally, we explored management strategies to decrease tea Al contents and to protect tea from potential Al risks in the study region.
2. MATERIALS AND METHODS
2.1. Study area
The study area is located in Nanchang County, Jiangxi Province, China, and belongs to a typical middle subtropical climatic zone. It has an average annual precipitation of 1600–1800 mm, with a mean annual relative humidity of about 78.5% and an average annual temperature of 17.5°C, with a mean annual frost-free period of 291 d yr−1. The soil type in the study region is hilly red soil (which belongs to the Ultisol soil order) which is a typical soil type in the central subtropical region of China. The soil of the area was derived from Quaternary red clay, formed from arenaceous shale, and is approximately 1 m in depth. Due to rapid industrialization and urbanization during the past 50 years, Nanchang has been considered one of the most serious regions for acid rain pollution. The percentage of acid rain events was 91.3%, with mean pH of 4.42 (Hu et al. Citation2002).
2.2. Study plots
An age-chronosequence of tea plantations was randomly distributed within the Huangma hilly red soil tea farm (28°20’N, 116°01’E, 800 ha, 30–70 m above sea level). The tea farm was established by the Institute of Tea Research, Jiangxi Province Department of Agriculture, in the early 1950s. In the spring of 2007, we selected three replicated plots (20 m × 20 m) for each planting age class including plots without tea planting (control, CK), below 10 years, 10–20 years, 20–30 years, 30–40 years, 40–50 years and over 50 years (only one replicate due to the very limited area of these old plantations). The three plots for the same age class are if possible located in different sites to the east, north and west, in order to analyze the potential spatial variability of soil properties (). All tea plantations have been managed by similar traditional practices with adequate fertilization, timely irrigation, comprehensive pest control, seasonal pruning of branches and harvesting of tea leaves. Generally, three episodes of fertilization including urea and compound fertilizer were used in February, May and October every year. The average fertilizer rate was 635.2, 154.1 and 136.8 kg ha−1 yr−1 for N, phosphorus pentoxide (P2O5) and potassium oxide (K2O), respectively. Three plots of typical wild shrubs as control treatments (CK) were selected in the east, north and west sites, respectively. The dominant species in CK plots included Adinandra millettii (Hook. et Arn.) Benth. et Hook. f. ex Hance, Dicranopteris dichotoma (Thunb.) Berhn., Lindera aggregata (Sims) Kosterm, Loropetalum chinense (R. Br.) Oliver and Quercus serrata var. brevipetiolata (A. DC.) Nakai. Plots selected for the study were on well-drained, flat sites.
Table 1 General properties of the 19 research plots in this study
2.3. Soil sample collection and chemical analysis
2.3.1. Sampling of the 0–40 cm soil layer
In each plot, we randomly selected nine points under the tea plant crown, and used a soil core sampler (10 cm in diameter) to separately collect samples from two layers at depths of 0–20 cm and 20–40 cm as topsoil and subsoil, respectively. For each layer, the samples were mixed completely to form a composite in each plot. All soil samples were brought to the laboratory for pretreatment. After roots and organic debris were removed by hand, the soil samples were air-dried, passed through a 2-mm sieve, ground to pass through a 0.149-mm sieve and then stored in a ground glass bottle before analyzing Al fractions and other properties. A total of 38 samples of air-dried soil were used to analyze soil chemical properties and Al fractions. Meanwhile, samples were digested by heating with perchloric acid- nitric acid (HClO4-HNO3) and analyzed for total Al by flame atomic absorption spectrometry (FAAS) (Lu Citation1999).
2.3.2. Soil Al fractionation with sequential extraction procedure
Soil Al fractionation was obtained by a sequential extraction procedure (Soon Citation1993; Walna et al. Citation2005; Duan et al. Citation2012). Air-dried soils were shaken in centrifuge tubes with 1 M potassium chloride (KCl), 0.1 M copper chloride (CuCl2), 1 M ammonium acetate (NH4OAc), 1 M hydrochloric acid (HCl) and 0.5 M sodium hydroxide (NaOH) solution, respectively. The Al concentrations in supernates extracted by the five chemical solutions mentioned above were determined by FAAS and respectively referred to as the concentrations of exchangeable aluminum (Ec-Al), organic aluminum complexes (Or-Al), sorbed inorganic aluminum (So-Al), hydrous oxide and hydroxide aluminum (Hy-Al) and humic acid aluminum (Hu-Al) (for details see ).
Figure 1 Soil Al fractionation sequential extraction procedure and its corresponding fractions (Revision according to Duan et al. Citation2012). KCl = potassium chloride, CuCl2 = copper chloride, NH4OAc = ammonium acetate, HCl = hydrochloric acid, NaOH = sodium hydroxide, Al = aluminium, Al3+ = trivalent aluminum ion, AlOM = organic aluminum, Al(OH)2+ = bivalent aluminium hydroxide ions, Al(OH)2+ = monovalent aluminium hydroxide ion, Al(OH)3 = aluminium hydroxide, AlHC = humus aluminum.
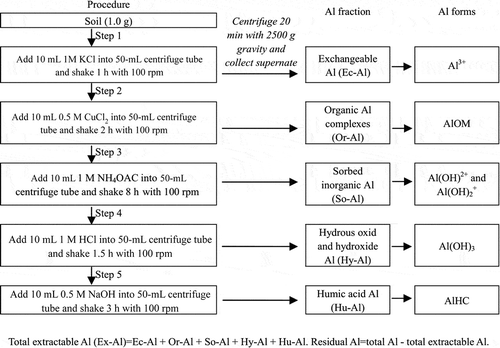
2.3.3. Soil chemical properties
Soil pH (soil: water = 1:2.5) was determined with a PHS-3C pH meter (Shanghai Lida Instrument Factory, China). Soil cation exchange capacity (CEC) was measured by an ammonium acetate exchange method (Lu Citation1999). Organic C was determined by the Walkley-Black wet oxidation method following the removal of carbonates by acid pretreatment (Allen Citation1989). Total N and total P were determined by the Kjeldahl method and by the molybdenum-stibium colorimetry method after the samples were digested with sulfuric acid (H2SO4) (Allen Citation1989). Additionally, soil C, N and P stoichiometric ratios were calculated based on their concentration in dry soils.
2.4. Plant sample collection and analysis
2.4.1. Sampling for fresh plant organs
Three individual tea plants were selected in each plot except CK plots. We used a hoe and a shovel to dig up the plant and the harvested roots (< 5 mm), stems (trunk), twigs (the top branches, outgrowth this year), and mature leaves. Meanwhile, new leaves (one bud with two leaves) were randomly collected within the plot. The samples of each plant organ were mixed to form a composite in each plot and brought to the lab in plastic bags.
2.4.2. Measurement of total Al and nutrient contents in each plant organs
All tissues were first washed in tap water, rinsed with deionized water and dried at 80°C for 48 h. After being ground and passed through a sieve (< 2 mm), the samples were stored in a valve bag at room temperature. For the determination of Al in plant tissues, the samples were digested with an acid mixture of HClO4-HNO3, and their Al concentrations were determined by FAAS (Lu Citation1999). Organic C was determined by the Walkley-Black wet oxidation method (Allen Citation1989). Total N and total P were determined by the Kjeldahl method and by the molybdenum-stibium colorimetry method after the samples were digested with H2SO4 (Allen Citation1989).
2.5. Data treatments
All data were tested for the normal distribution using the Kolmogorov-Smirnov Test. Two-way analysis of variance (ANOVA) was used to examine the effects of planting year, soil layer and their interactions on soil Al fractions. Meanwhile, three-way ANOVA was used to examine the effects of planting year, soil layer, location and their interactions on nutrient properties. Tukey’s multiple comparisons method was used to identify significant differences of Al and nutrient variables in soils and plants among different planting years after ANOVA tests for significance. SPSS software (SPSS Citation2007) was used in order to perform these analyses.
Finally, redundancy analysis (RDA) was performed to examine relationships between concentration of each Al fraction and nutrient properties in soils and plants using CANOCO 4.5 software (Monte-Carlo permutation test, 499 permutations and reduced model). RDA is an asymmetrical analysis that examines how a set of dependent variables Y relates to a set of independent variables X. “Redundancy” expresses how much of the variance in one set of variables can be explained by the other, and it is comparable to the squared multiple correlation in multiple linear regression (Afifi et al. Citation2003). Size and orientation of the arrow represents correlation among Al fraction and nutrient properties in soils and plants and with the axes. The smaller the angle between the arrows (or an arrow and an axis) and the longer the arrows, the more correlated are the variables represented by the arrows. The relationship was positive when the angle was less than 90° between two arrows and vice versa. And the correlation coefficients and P value can be obtained from linear regression using SPSS software. The standard 0.05 level of significance was used to designate a statistically significant effect.
3. Results
3.1. Al fractions and total Al in soils
The effect of planting year was significant on all soil Al fractions and total Al concentration. Except for the So-Al at both soil layers, Hy-Al in topsoil slightly decreased in older tea plantations, compared to the middle-age plantations; other soil fractions and total Al concentrations generally showed an increasing tendency with increasing planting year in topsoil and subsoil, respectively ().
Figure 2 Soil Al fractions varied with planting year and soil layer within a hilly red soil farm, southern China. PY = planting year, SL = soil layer, CK, control treatment. NS not significant, * P < 0.05, ** P < 0.01, ***P < 0.001. NS, *, **, and *** show the results of analysis of variance (ANOVA).
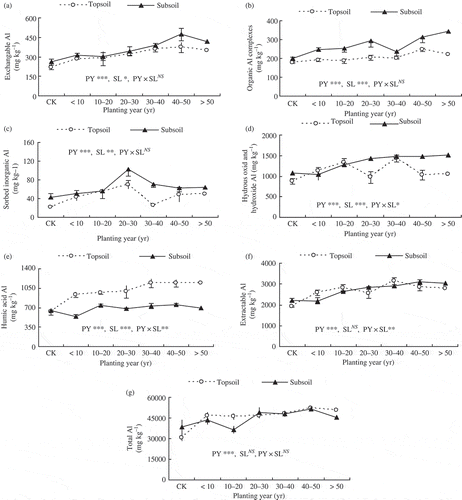
Significant differences in Al fractions between the two soil layers were found, with lower average concentrations of Ec-Al, Or-Al, So-Al and Hy-Al in topsoil than in subsoil, and an opposite tendency for Hu-Al (). By contrast, the average concentration of total Al was not significantly different between soil layers as well as the extractable Al (Ex-Al) (t = −0.24, P = 0.81) and residual Al (Re-Al) (t = 0.36, P = 0.72). Additionally, the interactions of planting year and soil layer were significant on Hy-Al and Hu-Al ().
Among the five extractable Al fractions, the percentage of Hy-Al was highest, occupying 36.09–47.01% and 46.94–51.02% in topsoil and subsoil, respectively, followed by Hu-Al (32.55–40.83% and 22.97–28.97% in topsoil and subsoil, respectively), and lowest for Ec-Al, Or-Al and So-Al, with all percentages being less than 15% at both soil layers (see Table S1).
3.2. Soil chemical properties
The separating effects of planting year and soil layer were significant on soil pH, CEC, organic C, total N and total P, but a location effect was not found (). Soil CEC, organic C, total N and total P showed an increasing tendency with increasing planting year, while soil pH showed an opposite tendency (). Soil C, N and P stoichiometric ratios were relatively stable across the age-chronosequence of tea plantations in our study (). Topsoil CEC, organic C, total N and total P were generally higher than those of subsoil, and pH, C/N and N/P showed the opposite tendency. Most interactions among planting year, soil layer and site location on soil properties were not found except with organic C () .
Table 2 Average values of soil chemical properties across an age-chronosequence of tea (Camellia sinensis L.) plantations within a hilly red soil farm, southern China
3.3. The concentrations of Al and nutrients in tea plants
The effects of planting year and plant organ were significant on total Al, total N and total P (). The concentrations of total Al and total N in different plant organs generally increased with planting year, except for a slight decrease in the plantation of > 50 years ( and ). In contrast, total P concentration in roots, stems and twigs generally increased with planting year, while total P in mature leaves and new leaves did not show this increasing tendency, although the lowest concentration was found in the youngest plantation ().
Figure 3 The concentrations of total Al and nutrients varied with planting year and plant organs within a hilly red soil farm, southern China. Note: The different letters represent significant differences in five plant organs among the plantations of different planting year (P < 0.05).
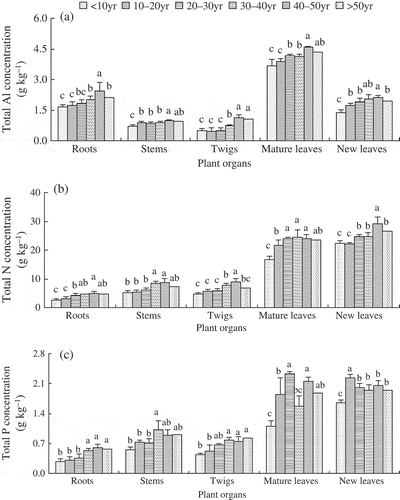
Clearly, total Al concentration in mature leaves was the highest, followed by roots and new leaves, and lowest for stems and twigs (). Both total N and P concentrations in mature and new leaves were higher than those in roots, stems and twigs ( and ). The ratio of N/P ranged from 7.5 to 16.4 (see Table S2), but was significantly influenced by planting year (F5,50 = 20.79, P < 0.001), plant organs (F4,50 = 111.42, P < 0.001) and their interactions (F20,50 = 18.77, P < 0.001).
3.4. Relations among Al level and nutrient properties in soils and plant organs
Soil Al fractions vs. soil nutrient properties relations varied with soil layer (). Topsoil pH was negatively correlated with Ec-Al (r = −0.69, P < 0.01), Or-Al (r = −0.69, P < 0.01), Hu-Al (r = −0.67, P < 0.01), Ex-Al (r = −0.65, P < 0.01) and total Al (r = −0.68, P < 0.01). Topsoil CEC was positively correlated with Ec-Al (r = 0.65, P < 0.01), Hu-Al (r = 0.72, P < 0.01), Ex-Al (r = 0.59, P < 0.01) and total Al (r = 0.65, P < 0.01). Both organic C and total N were positively correlated with Or-Al (r = 0.71, P < 0.01; r = 0.47, P < 0.05). Topsoil total P was positively correlated with Ec-Al (r = 0.61, P < 0.01), Hu-Al (r = 0.58, P < 0.05), Ex-Al (r = 0.62, P < 0.01) and total Al (r = 0.81, P < 0.001) ().
Figure 4 Biplot diagram for redundancy analysis (RDA) among the extractable aluminum (Al), total Al and chemical properties in (a) topsoil and (b) subsoil. The X and Y axes represent the percentages of total variations in extractable Al fractions that are explained by soil chemical properties. The plots represent 19 sampling sites in the distribution of the four quadrants. The projection location of plots to soil chemical properties arrows can express the value of soil chemical properties in these plots. OC = organic carbon, TN = total nitrogen, TP = total phosphorus, Ec-Al = exchangeable aluminum, Or-Al = organic aluminum complexes, So-Al = sorbed inorganic aluminum, Hy-Al = hydrous oxide and hydroxide aluminum, Hu-Al = humic acid aluminum, Ex-Al = extractable aluminum, CEC, soil cation-exchange capacity.
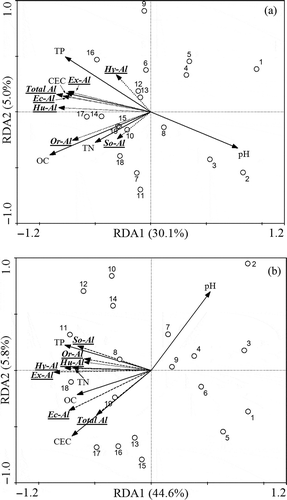
In contrast, subsoil pH did not correlate with Or-Al (r = −0.38, P > 0.05) and Hu-Al (r = −0.42, P > 0.05), CEC did not correlate with Ex-Al (r = 0.38, P > 0.05) and Hu-Al (r = 0.37, P > 0.05). Organic C was positively correlated with Ec-Al (r = 0.48, P < 0.05), Hy-Al (r = 0.48, P < 0.05) and Ex-Al (r = 0.72, P < 0.001). Total N was positively correlated with Or-Al (r = 0.52, P < 0.05), Hy-Al (r = 0.64, P < 0.01), Hu-Al (r = 0.55, P < 0.05), Ex-Al (r = 0.67, P < 0.01) and total Al (r = 0.48, P < 0.05). Subsoil total P was positively correlated with So-Al (r = 0.71, P < 0.01) ().
Meanwhile, the below-ground vs. above-ground relation in Al level varied with Al fraction and soil layer (). In general, topsoil Ec-Al, Hu-Al and Ex-Al were positively correlated with total Al concentrations in plant organs (). In contrast, subsoil Ex-Al, Or-Al and Hy-Al were positively correlated with total Al in most plant organs (). Clearly, the correlation of Al concentrations among different plant organs was much stronger than that of soil Al fractions in our selected sites ( and ).
Figure 5 Biplot diagram for redundancy analysis (RDA) among total aluminum (Al) in plant organs and extractable Al fractions in (a) topsoil and (b) subsoil. The X and Y axes represent the percentages of variations in total Al in plant organs that are explained by soil extractable Al fractions. The plots represent 19 sampling sites in the distribution of the four quadrants. The projection location of plots to soil extractable Al fractions arrows can express the value of soil extractable Al fractions in these plots. Ec-Al = exchangeable aluminum, Or-Al = organic aluminum complexes, So-Al = sorbed inorganic aluminum, Hy-Al = hydrous oxide and hydroxide aluminum, Hu-Al = humic acid aluminum, Ex-Al = extractable aluminum. Total Al = total aluminum concentration in soils, R-Al = total aluminum concentration in roots, S-Al = total aluminum concentration in stems, T-Al = total aluminum concentration in twigs, M-Al = total aluminum concentration in mature leaves, N-Al = total aluminum concentration in new leaves.
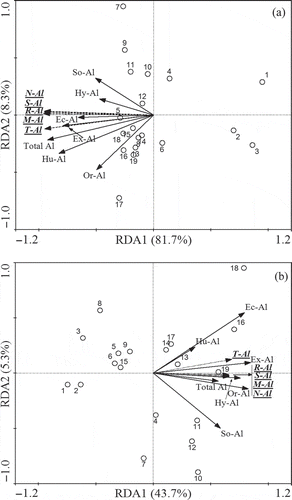
In addition, some relationships among total Al concentration and nutrient properties in plant organs were found (see Table S3). The relations of total Al vs. total N in twigs (r = 0.57, P < 0.05) and mature leaves (r = 0.57, P < 0.05), total Al vs. total P in roots (r = 0.57, P < 0.05) were significantly positive, and those of total Al vs. N/P ratio in twigs (r = −0.51, P < 0.05), mature leaves (r = −0.74, P < 0.01) and new leaves (r = −0.52, P < 0.05) were significantly negative, while other relations among total Al level vs. nutrients properties in plant organs were not significant (see Table S3).
4. Discussion
Al levels in both soils and plants generally increased with increasing planting years; this is supported by many similar studies in tea plantations (Flaten Citation2002; Carr et al. Citation2003; Chen et al. Citation2006). Since tea plants are an Al-accumulator shrub, Al can accumulate in the soil and plant of tea plantations as a result of Al biogeochemical cycling (Dong et al. Citation2001; Flaten Citation2002). As we know, Al is a strongly hydrolyzing metal and is relatively insoluble with pH ranging from 6.0–8.0 (Sposito Citation1996); however, the solubility of Al rapidly increases in acidic situations (pH < 6.0). In the tea plantations on acid soils, the mobility of Al increases sharply and it actively competes with other cations for exchangeable situation, because soluble Al, such as most Ex-Al fractions, is the most chemically and biologically available form for plants (Wong et al. Citation2003). Generally, the Al fractions derived from mineral Al in soil solution can transform to Al3+ and form corresponding complexes with phosphate or sulphate in acidic soils (Ruan et al. Citation2000; Fung and Wong Citation2002; Wong et al. Citation2003). Meanwhile, pH and CEC were correlated with Ec-Al, Or-Al, Hu-Al, Ex-Al and total Al in topsoil and subsoil, and all these results were observed by previous researchers (Dong et al. Citation2001; Xie et al. Citation2001). Clearly, soil pH would be the key factor to determine the extractable Al fractions and their availability to tea plants. Therefore, the decreasing pH with increasing planting year in our study could explain our results that soil available Al levels increased with planting year ( and ).
On the other hand, the Al levels in tea plant organs would be positively related to soil Al bio-availability. In this study, soil pH decreased along the age-chronosequence, which resulted in more available Al fractions in the older than in the younger tea plantations (). In acid tea soils with pH < 5.5, complex Al3+ forms are absorbed or transported passively into the root surface of the tea plant (Wong et al. Citation2003). After Al3+ was transported from soil to tea plants, part of Al3+ would stay in the roots, while excess Al3+ in the new leaves might form strong complexes with organic acid or polyphenol, and could transfer to the old leaves (Jayman and Sivasubramaniam Citation1980; Wong et al. Citation2003). Therefore, it is not surprising that the Al level was higher in old leaves than in young leaves, and Al levels in all plant organs generally increased with increasing planting year, as per our expectations. In addition, as an important Al source back into the soil, litters besides the pruning would be another reason to increase soil and plant Al levels along age-chronosequences in tea plantations (Shu et al. Citation2003). This deduction was tested by Ding and Huang (Citation1991), who found that the annual litter production of tea plantations was 3661 and 4572 kg ha−1 and the Al contents in litters were 21.64 and 28.77 kg ha−1 in 20- and 80-year-old tea plantations, respectively.
In the acidic soil, the Ec-Al is mainly present in five fractions of the extractable Al (Duan et al. Citation2012). Interestingly, the total Ex-Al and total Al contents were not significantly different between topsoil and subsoil in our tea plantation ( and ). However, the relative active Al fractions including Ec-Al, Or-Al, So-Al and Hy-Al in the tea plantations were generally lower in topsoil than in subsoil, while the relative inert Al fraction (Hu-Al) showed the opposite trend (–). The result was consistent with Satoh et al. (Citation1990), who reported that the mobility of Al was closely linked with organic acids, e.g. oxalic acid and citric acid, which were found more in the topsoil than in the subsoil. However, the Al complexes with low molecular organic acids easily and freely moved along the profile in acid soil (James and Riha Citation1989). Since the Al complex arrived in the subsoil, Al was re-released due to the increase of pH and microbial consumption. Therefore, the differences with lower active Al fractions in topsoil and higher inert Al fractions in subsoil were found in the tea plantations. Additionally, the abundant precipitation, often as acid rain (Hu et al. Citation2002), which leads to the leaching of ionic Al (Wang et al. Citation2009), would be another reason for the vertical distributions of lower active Al fractions in topsoil than in subsoil. According to a survey in the north Jiangxi province of China, the mean pH of precipitation during 1992–2006 was 4.76, and the rain pH decreased slowly in the 1990s, but a rapid decrease after 2002 was observed with pH below 4.5 (Zhu et al. Citation2010). In topsoil, an exchange reaction was easily executed between hydrogen ions (H+) in acid rain and Al on the surface of soil minerals, which led to an increase in production of Ec-Al. Afterwards, the Ec-Al was transported into the subsoil through leaching (Maitat et al. Citation2000).
In contrast, humic acids are complex compounds that exist in soils with high levels of organic matter formed by the microbial decay of plant litters (Ruan et al. Citation2004). In our study, the differences of Hu-Al between topsoil and subsoil increased with increasing planting year (). Among the five plant organs, the Al concentration was the highest in mature leaves, followed by roots and new leaves, and lowest in stems and twigs (). Clearly, the decomposition of foliar litters could be the major source of the soil Al level, especially the Hu-Al. The positive correlations among total Al in plant organs and topsoil but not subsoil Hu-Al would also be important evidence.
Previous studies have well reported the cycle of individual elements, such as Al (Flaten Citation2002), N (Han et al. Citation2012) and P (Debnath et al. Citation2011) in tea plantations, which were generally tested by our study. However, we know little about at what points the major biogeochemical cycles interact and how these linkages are affected by environmental change and fertilization management and the development of tea plantations (Marklein and Houlton Citation2012). In our study, as an intensively managed plantation, exogenous fertilizers, such as urea and compound fertilizer, were used three times within a year. Soil pH could decrease with the slathering of nitrogenous fertilizer due to the microbial oxidation of ammonium (Barak et al. Citation1997). Cregan and Scott (Citation1998) reported the pH decreased with the usage of ammonium fertilizer as 80 kg ha−1 in Tarlee, Australia. The acid-forming process was performed by the following three reactions (Barak et al. Citation1997):
Hydrolysis of urea and nitrification of products:
Nitrification of ammonium:
Nitrification of ammonia:
Where CO(NH2)2, O2, H+, NO3−, H2O, CO2, NH4+ and NH3 represent carbamide, oxygen, hydrogen ion nitrate, water, carbon dioxide, ammonium ion and ammonia, respectively. Clearly, these processes with the addition of N fertilizer would lead to decrease of pH, and thus increase Al availability. Unfortunately, we cannot quantitatively provide the relative contributions of N fertilization and acid deposition to increase soil Al availability because both can alter soil pH in our tea plantations.
Meanwhile, soil total P was strongly positively correlated with most Ex-Al fractions in both soil layers in our study (). Generally speaking, soil Al availability helps tea plants absorb P (Konishi et al. Citation1985). In acid tea soil, Al3+often forms a complex with P in soil solution, and the AlPx complexes have less charge, or are even neutral molecules (with or without organic acids), which are easily absorbed by tea plant (Wong et al. Citation2003). Thereafter, malic acid can chelate Al3+ at the roots and transport it to the young leaves while releasing phosphate for plant uptake (Wong et al. Citation2003). In this study, root total P waspositively correlated with root Al concentration (Table S3), which indicates that both P and Al were synchronously absorbed by roots. Meanwhile, the total N in twigs and mature leaves was positively correlated with the Al concentration in both plant organs, respectively, while their N/P were negatively correlated with the Al concentrations in twigs and mature leaves (Table S3). Clearly, N affected P and Al levels in new and mature organs. Meanwhile, N/P in new leaves were negatively correlated with their Al concentration (Table S3), respectively, which also indicates that P and Al were synchronously absorbed by new tissues as well as roots. Overall, decreased soil pH driven by N fertilizer and acid deposition was the key factor to explain the interactions among Al and nutrient cycling across the age-chronosequence of tea plantations.
In summary, both the Al biogeochemical cycle with increasing acid deposition and agronomic practices such as fertilization in tea plantation accelerated plant-soil Al cycling, and soil available Al concentrations and plant total Al levels increased with increasing planting year. Fertilization improved soil nutrient status, but the use of nitrogenous fertilizer would be an important reason to decrease soil pH. With the increasing soil acidity along the age-chronosequrence, elevated Al availability was helpful to increase P uptake by tea plant. In order to protect tea from potential Al risk, moderately reducing N fertilizer and removing pruning litters would be effective management practices especially in older tea plantations. Of course, using lime to amend strong acidification of soil shall be recommended as a direct pathway to ensure Al safety in tea plantations.
Supplementary Material
The supplementary material for this article is available online from: http://dx.doi.org/10.1080/00380768.2014.906295
Supplementary Tables S1-S3
Download MS Word (72 KB)ACKNOWLEDGMENTS
This work was supported by the National Natural Science Foundation of China (No. 31060081) and Jiangxi Provincial Department of Science and Technology (No. 20122BCB23005).
REFERENCES
- Afifi A, May S, Clark VA 2003: Computer-Aided Multivariate Analysis, The Chemical Rubber Company Press, Florida.
- Allen SE 1989: Chemical Analysis of Ecological Materials, 2nd edn. Blackwell Scientific Publications, Oxford.
- Bajaj K, Devchoudhury MN, Sukhija PS 1984: Effect of phosphorus on fatty acids of tea leaves and on the quality of teas. J. Food Sci. Technol., 21, 392–394.
- Barak P, Jobe BO, Krueger AR, Peterson LA, Laird DA 1997: Effects of long-term soil acidification due to nitrogen fertilizer inputs in Wisconsin. Plant Soil, 197, 61–69. doi:10.1023/A:1004297607070
- Carr H, Lombi E, Küpper H, McGrath SP, Wong MH 2003: Accumulation and distribution of aluminium and other elements in tea(Camellia sinensis) leaves. Agronomie, 23, 705–710. doi:10.1051/agro:2003045
- Chen YM, Wang MK, Zhuang SY, Chiang PN 2006: Chemical and physical properties of rhizosphere and bulk soils of three tea plants cultivated in Ultisols. Geoderma, 136, 378–387. doi:10.1016/j.geoderma.2006.04.003
- Cregan P, Scott B 1998: Soil Acidification-An Agricultural and Environmental Problem, pp. 98–128. CSIRO Publishing, Melbourne.
- Dang MV 2007: Quantitative and qualitative soil quality assessments of tea enterprises in Northern Vietnam. Afr. J. Agr. Res., 2, 455–462.
- Debnath A, Barrow NJ, Ghosh D, Malakar H 2011: Diagnosing P status and P requirement of tea (Camellia sinensis L.) by leaf and soil analysis. Plant Soil, 341, 309–319. doi:10.1007/s11104-010-0645-2
- Ding R, Huang X 1991: Biogeochemical cycle of aluminum and fluorine in tea garden soil system and its relationship to soil acidification. Acta Pedo. Sin., 28, 229–236. (in Chinese)
- Dong D, Xie Z, Du Y 2001: The bioavailability of Al in soils to tea plants. Appl. Geochem., 16, 1413–1418. doi:10.1016/S0883-2927(01)00052-X
- Duan XH, Hu X, Chen F, Deng Z 2012: Bioactive ingredient levels of tea leaves are associated with leaf Al level interactively influenced by acid rain intensity and soil Al supply. J. Food Agric. Environ., 10, 1197–1204.
- Falkowski P, Scholes R, Boyle E, Canadell J, Canfield D, Elser J, Gruber N, Hibbard K, Högberg P, Linder S 2000: The global carbon cycle: A test of our knowledge of earth as a system. Science, 290, 291–296. doi:10.1126/science.290.5490.291
- Flaten TP 2002: Aluminium in tea-concentrations, speciation and bioavailability. Coordination Chem. Rev., 228, 385–395. doi:10.1016/S0010-8545(02)00036-X
- Fung K, Carr HP, Zhang J, Wong MH 2008: Growth and nutrient uptake of tea under different aluminium concentrations. J. Sci. Food Agric., 88, 1582–1591. doi:10.1002/jsfa.3254
- Fung K, Wong MH 2002: Effects of soil pH on the uptake of Al, F and other elements by tea plants. J. Sci. Food Agric., 82, 146–152. doi:10.1002/jsfa.1007
- Ghanati F, Morita A, Yokota H 2005: Effects of aluminum on the growth of tea plant and activation of antioxidant system. Plant Soil, 276, 133–141. doi:10.1007/s11104-005-3697-y
- Han W, Kemmitt SJ, Brookes PC 2007: Soil microbial biomass and activity in Chinese tea gardens of varying stand age and productivity. Soil Biol. Biochem., 39, 1468–1478. doi:10.1016/j.soilbio.2006.12.029
- Han WY, Xu JM, Yi XY, Lin YD 2012: Net and gross nitrification in tea soils of varying productivity and their adjacent forest and vegetable soils. Soil Sci. Plant Nutr., 58, 173–182. doi:10.1080/00380768.2012.664783
- Hayashi K, Yan X 2010: Airborne nitrogen load in Japanese and Chinese agroecosystems. Soil Sci. Plant Nutr., 56, 2–18. doi:10.1111/j.1747-0765.2009.00423.x
- Hu C, Huang X, Li C 2002: Pollution features and changing regularity of acid rain in Nanchang city. Acta Agr. Univ. Jiangxiensis, 24, 689–691. (in Chinese)
- James BR, Riha SJ 1989: Aluminum leaching by mineral acids in forest soils: II. Role of the forest floor. Soil Sci. Soc. Am. J., 53, 264–296. doi:10.2136/sssaj1989.03615995005300010048x
- Jayman T, Sivasubramaniam S 1980: The use of fertilizers for tea in Sri Lanka. 3. Foliar and soil analysis with particular reference to P, Ca and Mn. Tea Q, 49, 137–141.
- Konishi S, Miyamoto S, Taki T 1985: Stimulatory effects of aluminum on tea plants grown under low and high phosphorus supply. Soil Sci. Plant Nutr., 31, 361–368. doi:10.1080/00380768.1985.10557443
- Lu RK 1999: Analysis Methods of Soil Agricultural Chemistry, China Agricultural Science and Technology Press, Beijing. (in Chinese)
- Maitat O, Boudot JP, Merlet D, Rouiller J 2000: Aluminum chemistry in two contrasted acid forest soils and headwater streams impacted by acid deposition, Vosges mountains, NE France. Water Air Soil Pollut., 117, 217–243. doi:10.1023/A:1005132321147
- Marklein AR, Houlton BZ 2012: Nitrogen inputs accelerate phosphorus cycling rates across a wide variety of terrestrial ecosystems. New Phytol., 193, 696–704. doi:10.1111/j.1469-8137.2011.03967.x
- Nayak P 2002: Aluminum: Impacts and disease. Environ. Res., 89, 101–115. doi:10.1006/enrs.2002.4352
- Rengel Z 2004: Aluminium cycling in the soil-plant-animal-human continuum. Biometals, 17, 669–689. doi:10.1007/s10534-004-1201-4
- Ruan J, Ma L, Shi Y 2006: Aluminium in tea plantations: Mobility in soils and plants, and the influence of nitrogen fertilization. Environ. Geochem. Health, 28, 519–528. doi:10.1007/s10653-006-9047-z
- Ruan J, Ma L, Shi Y, Zhang F 2004: Effects of litter incorporation and nitrogen fertilization on the contents of extractable aluminium in the rhizosphere soil of tea plant (Camallia sinensis (L.) O. Kuntze). Plant Soil, 263, 283–296. doi:10.1023/B:PLSO.0000047744.44940.96
- Ruan J, Zhang F, Wong MH 2000: Effect of nitrogen form and phosphorus source on the growth, nutrient uptake and rhizosphere soil property of Camellia sinensis L. Plant Soil, 223, 65–73. doi:10.1023/A:1004882001803
- Satoh F, Sakuma T, Okajima H 1990: A toposequence of fine-textured soils in the hilly area of the northernmost part of Hokkaido: III. Importance of chelating low molecular organic acids in the migration process of aluminum and iron 1 Cited from the Ph. D. Thesis of F. Satoh, Hokkaido University, 1985. Soil Sci. Plant Nutr., 36, 251–260.
- Shu W, Zhang Z, Lan C, Wong M 2003: Fluoride and aluminium concentrations of tea plants and tea products from Sichuan Province, PR China. Chemosphere, 52, 1475–1482. doi:10.1016/S0045-6535(03)00485-5
- Soon Y 1993: Fractionation of extractable aluminum in acid soils: A review and a proposed procedure. Commun. Soil Sci. Plant Anal., 24, 1683–1708. doi:10.1080/00103629309368908
- Sposito G 1996: The Environmental Chemistry of Aluminum, CRC Press, Boca Raton.
- SPSS, Inc. (2007). SPSS for Windows (16.0). Chicago IL: SPSS Inc.
- Tolrà R, Vogel-Mikuš K, Hajiboland R, Kump P, Pongrac P, Kaulich B, Gianoncelli A, Babin V, Barceló J, Regvar M, Poschenrieder, C. 2011: Localization of aluminium in tea (Camellia sinensis) leaves using low energy X-ray fluorescence spectro-microscopy. J. Plant Res., 124, 165–172. doi:10.1007/s10265-010-0344-3
- Vitousek PM, Aber JD, Howarth RW, Likens GE, Matson PA, Schindler DW, Schlesinger WH, Tilman DG 1997: Human alteration of the global nitrogen cycle: Sources and consequences. Ecol. Appl., 7, 737–750.
- Walna B, Siepak J, Drzymała S, Sobczyński T 2005: Research on aluminium speciation in poor forest soils using the sequential extraction method. Pol. J. Environ. Stud., 14, 243–250.
- Wang N, Li JY, Xu RK 2009: Use of agricultural by-products to study the pH effects in an acid tea garden soil. Soil Use Manag., 25, 128–132. doi:10.1111/j.1475-2743.2009.00203.x
- Wang H, Xu RK, Wang N, Li XH 2010: Soil acidification of alfisols as influenced by tea cultivation in eastern China. Pedosphere, 20, 799–806. doi:10.1016/S1002-0160(10)60070-7
- Wong M, Fung K, Carr H 2003: Aluminium and fluoride contents of tea, with emphasis on brick tea and their health implications. Toxicol Lett., 137, 111–120. doi:10.1016/S0378-4274(02)00385-5
- Xie Z, Ye Z, Wong M 2001: Distribution characteristics of fluoride and aluminum in soil profiles of an abandoned tea plantation and their uptake by six woody species. Environ. Int., 26, 341–346. doi:10.1016/S0160-4120(01)00010-1
- Xue H, Ren X, Li S, Wu X, Cheng H, Xu B, Gu B, Yang G, Peng C, Ge Y, Chang, J. 2013: Assessment of private economic benefits and positive environmental externalities of tea plantation in China. Environ. Monit. Assess., 185, 8501–8516. doi:10.1007/s10661-013-3191-6
- Zhu L, Hu Z, Hu R, Wang L, Xie Y 2010: Characteristic analysis of acid rain in recent 15 years of Ganzhou city, south Jiangxi. Acta Agr. Jiangxi, 22, 118–120. (in Chinese)