Abstract
Potassium (K) in microbial cells, microbial biomass K, in soil has been recently recognized as a K pool for plant growth. We determined soil microbial biomass K in paddy fields to reveal its importance as a K pool in paddy field soil for the first time. Microbial biomass K ranged from 5 to 21 mg K kg−1 in the soil samples periodically collected from a paddy field and the value corresponded to 41% of the exchangeable K on average. Both microbial biomass K and exchangeable K increased conspicuously due to the long-term application of livestock manure compost or rice straw compost. Biomass K was higher than exchangeable K under K-deficient conditions in the long-term experimental plots without K application. The present study revealed that the microbial biomass contained considerable amounts of K in paddy field soil, indicating the need for evaluation of the microbial biomass K as a source and a stock of K in soil that has been overlooked.
INTRODUCTION
Soil microbial biomass has important roles in supply of nutrients such as nitrogen (N), phosphorus (P) and sulfur (S) for plants as decomposers of organic materials and transformers of various elements. The microbial biomass also serves a reservoir for the nutrients though the proportion of the biomass is only 2–3% of the soil organic carbon (C) in general (Jenkinson and Ladd Citation1981), indicating that the amount of microbial biomass is a useful index of available nutrients for plants.
Potassium (K) is one of the three major essential nutrients and the amount of K uptake by crops is equivalent to or larger than that of the N uptake (Owa Citation2006) due to the high demand of K for plant growth. There are four forms of K in soil: water-soluble, exchangeable, non-exchangeable or fixed, and structural or mineral. Among them, the water-soluble and exchangeable forms are most readily available to plants (Sparks Citation2011), which usually account for less than 5% of total K in arable soil (Kirkman et al. Citation1994; Moritsuka et al. Citation2003). In addition, uptake of non-exchangeable K has been reported (Moritsuka Citation2009) in the rhizosphere (e.g., Okajima and Matsunaka Citation1973; Moritsuka et al. Citation2003). These inorganic forms of K in soil had been solely considered for K uptake by plants, and K in microbial cells in soil (microbial biomass K) has been ignored.
K is the major intracellular cation in all kinds of organisms and the concentration inside cells is generally maintained higher than that outside (Uozumi Citation2011). Bacteria and fungi also accumulate K inside the cells with a concentration greater than 0.18–0.2 M (Brown Citation1964; Slayman and Tatum Citation1964). Thus, it can be expected that soil microbial biomass is a significant K pool. However, only a few studies on biomass K in soil have been reported.
Anderson and Domsch (Citation1980) showed that the average ratio of K to C in microbial biomass was 1 to 0.098 by determining the C and K contents in microbial cells, and that the biomass in soil contained substantial quantities of K. Roberts (Citation1968) and Perrott et al. (Citation1990) found significant releases of K from microbial biomass K in grassland soils by measuring seasonal changes in the amounts of microbial biomass K. Tateishi (Citation1998) showed that soil microbial biomass could be a significant K pool for plants in the secondary succession after fires in a Japanese red pine (Pinus densiflora Sieb. & Zucc.) forest. Khan et al. (Citation2009) estimated the biomass K contents in the soils contaminated with heavy metals by the chloroform-fumigation extraction method. In these studies, however, the amount of biomass K had not been surveyed in a wide range of soils, particularly agricultural soils, and the conversion factor (k factor) for calculating the biomass K from the flush K by the chloroform-fumigation had not been determined.
Lorenz et al. (Citation2010) recently established a rapid assay for the determination of biomass K in soil by the chloroform-fumigation extraction method using a range of agricultural soils, and showed the k factor for the biomass K for the first time. They showed that the ratio of the biomass K to the exchangeable K was 0.37 on average in cornfield soils and indicated the importance of the biomass K as a reservoir of K in soil.
Although the K uptake surpasses the N uptake and is important for growth of rice (Oryza sativa L.) plants, there is still no study on the biomass K in paddy field soil so far. Therefore, the aims of the present study are to evaluate the amount of biomass K in paddy field soil and to show the importance of biomass K as a possible source of available K for rice plants. We estimated the factor kK for paddy field soil, determined the amount and the seasonal fluctuation of biomass K and examined effects of organic fertilizer application on the amounts in paddy field soil.
Table 1 Soil properties and fertilization rate (kg ha−1) in Omagari field
Table 2 Contents of biomass carbon (C) and nitrogen (N) in Omagari field (mg C or N kg−1 soil)
MATERIALS AND METHODS
Studied field
Two experimental paddy fields located at the Aichi-ken Anjo Research and Extension Center, Anjo, Aichi, Japan (Anjo; 34°58'N, 137°4'E) and the NARO Tohoku Agricultural Research Center, Daisen (formerly Omagari), Akita, Japan (Omagari; 39°29'N, 140°30'E) were used.
The paddy field plot in Anjo was cropped with rice (Oryza sativa L. cv. Aichinokaori SBL) and wheat (Triticum aestivum L. cv. Kinuakari) in summer and winter, respectively. The soil was Oxyaquic Dystrudept with a Light Clay texture (Soil Survey Staff Citation1999); total C content of 12.6 g kg−1; total N content of 1.1 g kg−1 and pH (H2O), 5.8. The area of the plot was 0.5 ha. Puddling was conducted and rice seedlings were transplanted in late May. Rice plants were harvested in mid-October. A compound chemical fertilizer (BB464, JA Group Aichi, Aichi, Japan; 56 kg ha−1 of N, 80 kg ha−1 of phosphorus pentoxide [P2O5] and 56 kg ha−1 of potassium oxide [K2O]) was applied as a basal fertilizer for rice in late May. For top dressing, a compound chemical fertilizer (BBNK2, JA Zen-Noh, Tokyo, Japan) was applied with 19 kg ha−1 of N and 19 kg ha−1 of K2O in early August and 24 kg ha−1 of N and 24 kg ha−1 of K2O in mid-August. The plot was flooded in late May and drained for about a week in mid-July (mid-season drainage) and in early October before harvesting rice. Wheat plants were grown in the plot from mid-December under upland conditions. Details of the field managements including cultivation, water regime and fertilizer application in 2011 are shown in .
Figure 1 Management of the paddy field plot in Anjo field in 2011: (a) puddling and basal fertilizer (on May 26); (b) transplanting for rice (Oryza sativa L.) seedlings (on May 30); (c) mid-season drainage (from July 8 to 15); (d) top dressing (on August 3); (e) top dressing (on August 12); (f) drainage (on October 3); (g) harvesting (on October 13); (h) cultivation of wheat (Triticum aestivum L.) (from December 12).
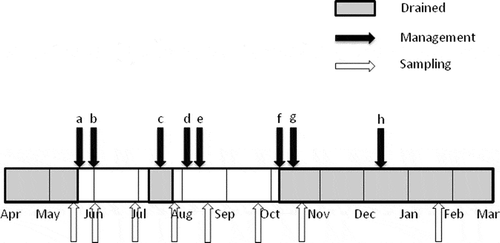
The paddy field in Omagari consisted of two experimental fields with long-term application of livestock manure compost (field A) and rice straw compost and chemical fertilizers (field B). Outlines of the experiments were reported by Kanamori (Citation2000). The soil was Typic Fluvaquents with the texture Clay Loam. Soil properties and details about fertilizer application to fields A and B were summarized in .
The experiment in field A was established in 1973 and livestock manure compost was annually applied with 3.6 × 104 kg ha−1 (fresh weight) except for in 2006 and 2007. Four plots, livestock manure compost plot (MC), livestock manure compost plus chemical fertilizer plot (MCCF), chemical fertilizer plot without application of livestock manure compost (CF) and no fertilizer plot (NF), were used for the soil sampling. The plots were cropped with rice (Oryza sativa L. cv. Bekoaoba) except that soybean (Glycine max [L.] Merr.) was grown in 2006 and 2007 under upland conditions. The area of each plot was 100 m2. Puddling was conducted and rice seedlings were transplanted in mid-May. Rice plants were harvested in late September. The plot was flooded in early May and drained for about a week between late June and early July (mid-season drainage) and it was drained in early September before harvesting rice.
The experiment in field B was established in 1968. The rice cultivar used was Kiyonishiki. Three plots, rice straw compost plus chemical fertilizer plot (RSC), chemical fertilizer plot (NPK) and no potassium fertilizer plot (NP), with two replicates, were used for the soil sampling. The area of each plot was 40 m2. Puddling was conducted and rice seedlings were transplanted in mid-May. Rice plants were harvested in late September.
Soil sample
Soil samples were periodically collected from the plow layer of the Anjo field during the rice cultivation period in 2011. Four soil samples (about 500 g and located > 2 m apart) were collected from each plot at a depth of 0–10 cm on May 19 (before flooding), June 1 (after rice transplanting), June 29 (before mid-season drainage), July 26 (after mid-season drainage), August 19 (after the second top dressing), September 20 (before rice harvest) and October 17 (after drainage and rice harvest) (). A soil sample was collected from the Anjo field for determination of the kK factor on January 20, 2012 (under wheat cultivation). Soil samples were taken from the Omagari fields on August 2 (after mid-season drainage) in 2011 by the same method as described above. The four soil samples from each plot were combined into a polyethylene bag and transported to the laboratory. The soil samples were then passed through a 2-mm mesh sieve and stored at 4°C until use.
Determination of microbial biomass K, C and N
Microbial biomass was determined by the chloroform-fumigation extraction method (Vance et al. Citation1987). The submerged soil sample was spread in a Petri dish to form a thin soil layer, according to Inubushi et al. (Citation1984). The 10 g portion of the fumigated or non-fumigated soil was extracted with 50 mL of 1 M ammonium acetate for determination of the biomass K and exchangeable K (Lorenz et al. Citation2010), where the ratio of soil to extractant was changed from 1:10 to 1:5. Fifty milliliters of 0.5 M potassium sulfate was used for extracting biomass C and N (Vance et al. Citation1987) according to the protocols described by Sakamoto (Citation1997, Citation2003). Inorganic K was determined by inductively coupled plasma atomic emission spectrometry (ICP-AES) (IRIS model, Nippon Jarrell-Ash Co., Ltd., Kyoto, Japan). Total organic C and N in the extracts were quantified with a TOC analyzer (TOC-VCPH, Shimadzu, Kyoto, Japan). All the measurements were performed in triplicates. Values for the conversion factors, kC and kN, were 0.45 and 0.45 (Joergensen Citation1996; Urashima Citation2013) for the calculation of biomass C and N, respectively. The factor kk for biomass K was estimated as described below and used for the calculation.
Determination of kK factor for paddy field soil
The soil sample taken from the Anjo field on January 20, 2012, was used for determination of the kK factor according to the method described by Castellano and Dick (Citation1991) and Lorenz et al. (Citation2010). In brief, 10 g of the soil sample was diluted with 95 mL of 0.15 M sodium chloride (NaCl) solution supplemented with two drops of Tween 20. One milliliter of the 10−4 diluted suspension was inoculated to 750 mL nutrient broth (8 g L−1) with 100 mg L−1 cycloheximide for growing bacteria or 1 mL of the 10−1 diluted suspension to 750 mL potato dextrose broth (24 g L−1) with 100 mg L−1 streptomycin chloride and 5 mg L−1 tetracycline hydrochloride for growing fungi. The preparations were incubated at 25°C for 4 d. One milliliter of the culture solution was spread onto the respective solid medium supplemented with 15 g L−1 agar and incubated at 25°C for 4 d. The lawn of microorganisms grown on the plate was harvested and suspended in 1 mL of 0.15 M NaCl solution by homogenization with a vortex mixer (VORTEX-GENE 2, M&S Instruments Inc., Osaka, Japan). The suspension was washed with 1 mL of 0.15 M NaCl solution three times by centrifugation and decantation to remove free K outside the cells. One milliliter of the mixed bacterial or fungal suspension was inoculated to 10 g fresh soil sample and mixed. K was extracted and determined after chloroform-fumigation of the inoculated and uninoculated soil samples as described above. Non-fumigated soil was used as a control. Total K in the bacterial and fungal suspensions was determined by the boiling nitric acid (HNO3) method according to Helmke and Sparks (Citation1996). The bacterial (kKb) or fungal (kKf) factor was estimated as:
The kK factor was calculated as
based on the ratio of bacterial and fungal biomass in soil by Anderson and Domsch (Citation1980). The determination was performed in triplicate.
Statistical anaylsis
One-way analysis of variance (ANOVA), Tukey’s significant test, t-test and correlation analysis were performed with Microsoft Excel 2013 for Windows (Microsoft Corp., Tokyo, Japan) and Ekuseru-Toukei 2012 (Social Survey Research Information Co., Ltd., Tokyo, Japan).
RESULTS
kK factor for paddy field soil
The flush, (K in inoculated soil) – (K in uninoculated soil), was 8.32 ± 1.27 mg K kg−1 soil and 10.5 ± 2.1 mg K kg−1 soil (average ± SE, n = 3) for bacterial and fungal inoculation, respectively; K in inoculated bacterial and fungal biomass was 35.5 ± 9.1 mg K kg−1 soil and 71.6 ± 1.7 mg K kg−1 soil, respectively. Then, kKb and kKf factors were 0.234 ± 0.053 and 0.146 ± 0.027, respectively. The kK factor was calculated to be 0.168 ± 0.033.
Soil biomass K, C and N in Anjo paddy field under rice cultivation
Amount of K from fumigated soil was significantly greater than that from unfumigated soil for each sample (P < 0.05). Biomass K and exchangeable K in the Anjo paddy field soil ranged from 5 to 21 mg K kg−1 soil and 21 to 90 mg K kg−1 soil, respectively (). The ratio of biomass K to exchangeable K was 0.09–0.99. The content of biomass K tended to increase slightly during the sampling period, while it decreased and increased after mid-season drainage and the top-dressing, respectively. The content of exchangeable K decreased during the period except for after top-dressing in August and after drainage in October. Contents of biomass C and N ranged from 121 to 273 mg C kg−1 soil and from 13 to 28 mg N kg−1 soil, respectively ().
Effect of organic fertilizer application on soil biomass K, C and N
A significantly greater amount of K was extracted from fumigated soil than from unfumigated soil for each sample in Omagari paddy field (P < 0.05). shows biomass K and exchangeable K in Omagari paddy field plots. In field A, contents of biomass K and exchangeable K were 3.3 and 11.4 times higher in the MC plot than the NF plot, and 2.4 and 6.2 times higher in the MCCF plot than the CF plot, by the application of livestock manure compost. The content of exchangeable K was similar between MC and MCCF, but that of biomass K was different between the plots. On the other hand, the content of exchangeable K was different between CF and NF, but that of biomass K was similar between the plots. In field B, biomass K and exchangeable K showed significantly larger values in the RSC plot than the NPK and NP plots by the ANOVA and Tukey tests. The ratio of biomass K to exchangeable K was between 0.46 and 0.73 for the soil in the plots (MC, MCCF and RSC) containing more than 25 mg K kg−1 of exchangeable K. The ratio showed a higher value, between 0.93 and 2.37, for the soil in the plots (NF, CF, NPK and NP) containing exchangeable K of less than 25 mg K kg−1, where the contents of exchangeable K in these four plots were extremely low, less than 10 mg K kg−1. Contents of biomass C and N ranged from 43 to 64 mg C kg−1 soil and from 3.6 to 8.8 mg N kg−1 soil in the NF, CF, NPK and NP plots and from 89 to 119 mg C kg−1 soil and from 19 to 22 mg N kg−1 soil in the MC, MCCF and RSC plots, respectively ().
Figure 3 Contents of biomass potassium (K) and exchangeable K in Omagari field. MC, livestock manure compost plot; MCCF, livestock manure compost plus chemical fertilizer plot; CF, chemical fertilizer plot without application of livestock manure compost; NF, no fertilizer plot; RSC, rice straw compost plus chemical fertilizer plot; NPK, chemical fertilizer plot; NP, no potassium fertilizer plot. Error bars indicate standard errors of triplicate measurements for MC, MCCF, CF and NF, and ranges of mean values of triplicate measurements from two replicate plots for RSC, NPK and NP.
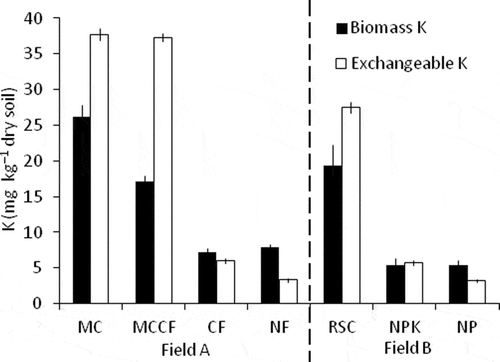
DISCUSSION
Lorenz et al. (Citation2010) reported that the kK factor ranged from 0.18 to 0.24 for three kinds of cornfield soils with various textures – fine sand, silt loam and clay. The value of the kK factor for paddy field soil in the present study was 0.168 and the sole and first estimation of the kK factor for paddy field soil. The value was a little smaller than the values, 0.18 and 0.20, for the silt loam and clay soils, respectively, by Lorenz et al. (Citation2010). The ratio of bacterial to fungal biomass in paddy field soil is possibly different from the value found by Anderson and Domsch (Citation1980) and this may affect the kK factor. Hasebe et al. (Citation1984) showed that the proportion of bacterial biomass in total microbial biomass was 0.82–0.99 in paddy field soil by the direct counting method, indicating the predominance of bacterial biomass over fungal biomass. In contrast, the proportion of fungal biomass was inferred to be about 0.67 in paddy field soil based on the estimation of rDNA abundance in paddy field soil (Sakai et al. Citation2012). We tentatively used the ratio of 1:3 reported by Anderson and Domsch (Citation1980) for the calculation of the kK factor in the present study due to lack of confirmed information about the ratio of bacterial to fungal biomass in paddy field soil. Further estimations of the kK factor are still necessary for various paddy soils under different conditions, as repeatedly mentioned also in the study by Lorenz et al. (Citation2010), for example under flooded conditions, because the factor in the present study was from the paddy soil under drained conditions cropped with wheat.
The contents of biomass K in the present study ranged from 4.8 to 26 mg K kg−1 and were smaller than the values, from 68 to 179 mg K kg−1, in Ohio soils reported by Lorenz et al. (Citation2010). The ratio of biomass K to exchangeable K in Anjo field was 0.41 on average, indicating that considerable amounts of K were present in the microbial biomass, also in paddy field soil. By using the data of biomass K and N in Anjo field, and assuming that the bulk density of the soil was 1.0 and the depth of the plow layer was 10 cm, average amounts of biomass K and N per area were estimated to be 13 kg K ha−1 and 19 kg N ha−1, respectively. The application doses of K and N fertilizers for rice per year in the Anjo field were 82 kg K ha−1 and 99 kg N ha−1 and average amounts of K and N uptake by rice plants were 130 kg K ha−1 and 111 kg N ha−1 (Owa Citation2006), though the K and N uptake by rice plants in the Anjo field was not measured in the present study. The amounts of biomass K and N corresponded to 16 and 19% of the annual application dose of K and N fertilizers, and 10 and 17% of the K and N uptake by rice plants, respectively, based on these estimations. This suggests that biomass K could be an important source of K supply for rice plants in paddy field soil, as biomass N is well recognized as an important N source, though turnover rates of the biomass K and N are not considered in the estimations.
The contents of biomass K showed a seasonal fluctuation in the present study (). A peak was found before harvesting of rice in September and the content was about four times higher than the minimum value recorded before flooding in May. Roberts (Citation1968) and Perrott et al. (Citation1990) indicated the accumulation of K in microbial biomass in pasture soils during winter and release of K from the biomass during spring and afterward, with about four times difference between the maximum and minimum values. In the present study, the content of biomass K seemed to decrease with mid-season drainage of the field and increase with K application as the top-dressing (). However, contents of the exchangeable K, biomass C and biomass N did not fluctuate correspondingly (). Therefore, we should be cautious to conclude that the drainage and fertilizer application caused the fluctuation of biomass K. Further investigation will be needed to understand dynamics of biomass K in paddy field soil and effects of various factors such as fertilizer application, water regime and K uptake by rice plants on it.
Application of organic fertilizers, livestock manure compost and rice straw compost increased amounts of biomass K as well as exchangeable K in Omagari field (). Crop residues such as rice straw and animal wastes are effective K source for plants, and most of the K in these materials is soluble (Xie and Hasegawa Citation1985). Long-term application of livestock manure compost or rice straw compost increased exchangeable K. Those also increased microbial biomass in soil, as evidenced by the contents of biomass C and N in , and resulted in an increase of the K contents in microbial biomass. It is interesting that the ratio of biomass K to exchangeable K was higher in the soils without application of organic fertilizers (0.93–2.37) than in the soils with organic fertilizers (0.46–0.73). In particular, the contents of biomass K were higher than those of exchangeable K in the soils without K application (NF and NP plots) though the ratios in Omagari field were higher than those in Anjo field, which may be attributed to differences in the soil properties of the two fields such as soil texture or clay mineralogy. These findings indicate that the pool size of K in microbial biomass may have a limit and excess K exists as water-soluble or exchangeable forms in soil, and that microbial biomass predominantly may take up and retain K under K-deficient conditions. Both the size of the microbial biomass and the K content in soil may influence amount of biomass K.
Khan et al. (Citation2009) reported a positive correlation between biomass K and biomass C. Lorenz et al. (Citation2010) showed that there was a positive correlation between biomass K and total C. In the present study, no correlation was found between biomass K and biomass C, N, and exchangeable K either when the data were compared within Anjo field (data not shown) or when all the data in the both fields were used except between biomass K and biomass N (P < 0.05) (). However, significant correlations were found between biomass K and biomass C, N and exchangeable K using only the data in Omagari field (). Because the application of compost increased the contents of exchangeable K and biomass K, C and N in Omagari fields as described above, a clear correlation was observed between biomass K and other parameters. In contrast, some factors other than organic matter application could have influenced the relationships between biomass K and the parameters since only chemical fertilizer was applied in the Anjo field. However, this remains to be elucidated in the present study. Further investigation of soil samples from various paddy fields will reveal the relationship between biomass K and the other parameters mentioned above.
Figure 4 Relationships between (a) biomass potassium (K) and biomass carbon (C), (b) biomass K and biomass nitrogen (N) and (c) biomass K and exchangeable K, in Anjo and Omagari fields. The dotted line represents the correlation for all data (n = 17) and the solid line represents the correlation for the data in Omagari field (n = 10).
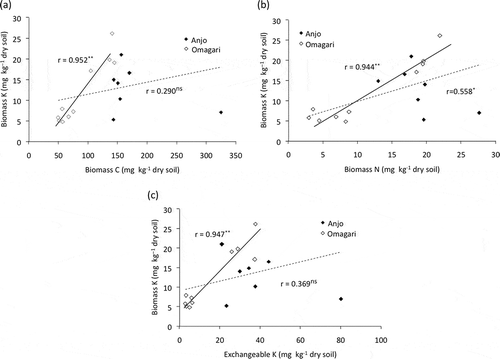
The present study showed for the first time that the microbial biomass contained considerable amounts of K and could be an important K source for rice plants in paddy field soil, indicating the need for evaluation of the microbial biomass K as a source and a stock of K in soil that has been overlooked. Evaluation of the turnover rate of biomass K is requisite to estimate the contribution of biomass K to uptake of K by rice plants. In addition, further investigations are necessary for estimation of the kk factor in various soils and measurement of biomass K in different types of soils under conditions with varied field managements. Biomass K will be taken into account to consider K fertilization and availability of K to crops in soil by using the information mentioned above.
ACKNOWLEDGMENTS
We thank staff members of the Aichi-ken Anjo Research and Extension Center and the NARO Tohoku Agricultural Research Center for their help in the soil sampling.
REFERENCES
- Anderson JPE, Domsch KH 1980: Quantities of plant nutrients in the microbial biomass of selected soils. Soil Sci., 130, 211–216. doi:10.1097/00010694-198010000-00008
- Brown AD 1964: Aspects of bacterial response to the ionic environment. Bacteriol. Rev., 28, 296–329.
- Castellano SD, Dick RP 1991: Modified calibration procedure for the measurement of microbial sulfur in soil. Soil Sci. Soc. Am. J., 55, 283–285. doi:10.2136/sssaj1991.03615995005500010049x
- Hasebe A, Kanazawa S, Takai Y 1984: Microbial biomass in paddy soil. I. Microbial biomass calculated from direct count using fluorescence microscope. Soil Sci. Plant Nutr., 30, 175–187. doi:10.1080/00380768.1984.10434681
- Helmke PA, Sparks DL 1996: Lithium, sodium, potassium, rubidium, and cesium. In Methods of Soil Analysis, Part 3. Chemical properties, Ed. Sparks DL, pp. 561–562. SSSA, Madison.
- Inubushi K, Wada H, Takai Y 1984: Determination of microbial biomass-nitrogen in submerged soil. Soil Sci. Plant Nutr., 30, 455–459. doi:10.1080/00380768.1984.10434710
- Jenkinson DS, Ladd JN 1981: Microbial biomass in soil: measurement and turnover. In Soil Biochemistry, Vol. 5, Ed. Paul EA, Ladd JN, pp. 415–471. Marcel Dekker, New York and Basel.
- Joergensen RG 1996: The fumigation-extraction method to estimate soil microbial biomass: calibration of the kEC value. Soil Biol. Biochem., 28, 25–31. doi:10.1016/0038-0717(95)00102-6
- Kanamori T 2000: Long-term field experiments on successive application of chemical fertilizers and composts as organic matters in national and prefectural agricultural research stations of Japan. Misc. Pub. Natl. Agric. Res. Cent., 42, 1–303. (in Japanese).
- Khan KS, Heinze S, Joergensen RG 2009: Simultaneous measurement of S, macronutrients, and heavy metals in the soil microbial biomass with CHCl3 fumigation and NH4NO3 extraction. Soil Biol. Biochem., 41, 309–314. doi:10.1016/j.soilbio.2008.11.001
- Kirkman JH, Basker A, Surapaneni A, MacGregor AN 1994: Potassium in the soils of New Zealand—a review. New Zealand J. Agric. Res., 37, 207–227. doi:10.1080/00288233.1994.9513059
- Lorenz N, Verdell K, Ramsier C, Dick RP 2010: A rapid assay to estimate soil microbial biomass potassium in agricultural soils. Soil Sci. Soc. Am. J., 74, 512–516. doi:10.2136/sssaj2008.0391
- Moritsuka N 2009: Forms of potassium in agricultural soils in Japan: evaluation at regional, field and root-zone scales. Jpn. J. Soil Sci. Plant Nutr., 80, 80–89. (in Japanese).
- Moritsuka N, Yanai J, Fujii A, Sano S, Kosaki T 2003: Evaluation of readily available nonexchangeable potassium in soil by sequential extractions with 0.01 molar hydrochloric acid. Soil Sci. Plant Nutr., 49, 631–639. doi:10.1080/00380768.2003.10410053
- Okajima H, Matsunaka T 1973: Studies on the rhizosphere soil (part 1): on the trends of mineral ions in the rhizosphere soil solution of maize and alfalfa. J. Sci. Soil Manure Jpn., 44, 413–420. (in Japanese with English summary).
- Owa N 2006: The fundamentals of fertilization. In Encyclopedia of Fertilizers, Ed. Owa N, Kimura M, Koshino M, Saigusa M, Tadano T, Hasegawa I, Yoshiba M, pp. 207–212. Asakura Publishing, Tokyo. (in Japanese).
- Perrott KW, Sarathchandra SU, Waller JE 1990: Seasonal storage and release of phosphorus and potassium by organic matter and the microbial biomass in a high-producing pastoral soil. Aust. J. Soil Res., 28, 593–608. doi:10.1071/SR9900593
- Roberts FJ 1968: The annual pattern of potassium release from organic matter in sandy soils of south-west Australia. Aust. J. Soil Res., 6, 193–202. doi:10.1071/SR9680193
- Sakai Y, Hayatsu M, Fujii T 2012: Estimation of rDNA abundance of Bacteria, Eukarya and Archaea in agricultural soils: soil factors affecting the abundance of archaeal rDNA. Soil Microorganisms, 66, 63–69. (in Japanese with English summary).
- Sakamoto K 1997: Soil Biomass. In Analytical Methods for Soil and Environments, Ed. Konno T, pp. 146–156. Hakuyusha, Tokyo. (in Japanese).
- Sakamoto K 2003: Measurement of soil microbial biomass. In Manual of Microbiological Experiments for Beginners, 2nd ed, Ed. Ando A, pp. 58–62. Gihodo, Tokyo. (in Japanese).
- Slayman CW, Tatum EL 1964: Potassium transport in Neurospora. 1. Intracellular sodium and potassium concentrations, and cation requirements for growth. Biochim. Biophys. Acta., 88, 578–592.
- Soil Survey Staff 1999: Chapter 15 Inceptisols. In Soil Taxonomy, A Basic System of Soil Classification for Making and Interpreting Soil Surveys, 2nd ed, pp. 518–524. United States Department of Agriculture Natural Resources Conservation Service, Washington DC.
- Sparks DL 2011: Bioavailability of soil potassium. In Handbook of Soil Science: Resource Management and Environmental Impacts, 2nd ed, Ed. Huang PM, Li Y, Sumner ME, pp 11-37–11-47. CRC press, Boca Raton, London, New York.
- Tateishi T 1998: The role of soil microbial biomass in burned Japanese red pine forest. Environ. For. Sci., 8, 261–268.
- Uozumi N 2011: Research on transporters and its impact on the fields of soil science, fertilizers and plant nutrition. 5. Na tolerance and Na circulation in plants. Jpn. J. Soil Sci. Plant Nutr., 82, 65–69. (in Japanese).
- Urashima Y 2013: Chloroform-fumigation method. In Experimental Methods for Soil Microorganisms, 3rd ed, Ed. Japanese Society of Soil Microbiology, pp. 87–90. Yokendo, Tokyo. (in Japanese).
- Vance ED, Brookes PC, Jenkinson DS 1987: An extraction method for measuring soil microbial biomass C. Soil Biol. Biochem., 19, 703–707. doi:10.1016/0038-0717(87)90052-6
- Xie J-C, Hasegawa M 1985: Organic and inorganic sources of potassium in intensive cropping systems: experiences in the People’s Republic of China and Japan. In Potassium in Agriculture, Ed. Munson RD, pp. 1177–1199. American Society of Agronomy; Crop Science Society of America; Soil Science Society of America, Madison.