Abstract
Radioactivity levels of cesium (Cs)-134 and 137Cs in bamboo [Phyllostachys reticulata (Rupr) K. Koch] sprouts grown from April to June 2011 over a wide area (including Fukushima Prefecture) were elevated (max. 3100 Bq kg−1 fresh weight) after the Tokyo Electric Power Company, Inc. (TEPCO) Fukushima Daiichi Nuclear Power Plant disaster in March 2011. Bamboo sprouts in 2012 also contained high radioactivity levels. Radioactivity imaging analysis of bamboo sprouts harvested in 2012 showed increasing concentration gradients of radioactivity from the lower parts to the top of the sprouts. The peels were individually separated from the sprouts, and the inner edible part (trunk) was cross-sectioned at the internodal sections from the top to the lower parts. Each segmented trunk and its corresponding peel were analyzed for radioactive cesium (134Cs and 137Cs) and stable cesium (133Cs). The concentrations of 134Cs and 137Cs showed significant increases from the lower part to the top, whereas 133Cs showed an almost constant value in the trunk and peel except in the peel of the top node. We speculated that 134Cs and 137Cs in newly emerging bamboo sprouts in 2012 were translocated mainly from various plant tissues (where the fallout was layered on the bamboo tissues) in older bamboo, while 133Cs was translocated from the soil through the roots of the new bamboo sprouts and was present in the roots and stems.
Key words:
INTRODUCTION
After Tokyo Electric Power Company, Inc. (TEPCO) Fukushima Daiichi Nuclear Power Plant disaster on March 11–20, 2011 (Brumfiel Citation2011; Nuclear Regulation Authority Citationn.d.a), high levels of radioactive cesium (134Cs and/or 137Cs) were detected in many foods harvested in 2011 from a wide area, including Fukushima Prefecture, which was contaminated with fallout from TEPCO’s Nuclear Power Station. However, the concentrations of radioactive cesium in the majority of crops and vegetables in 2012 decreased to less than 1/10th of those in 2011. In contrast, the concentrations of 134Cs and 137Cs in wild plants did not decrease as significantly as in cultivated plants (Fukushima Prefecture Citationn.d.). For example, radioactive cesium in the edible part (trunk) of bamboo (Phyllostachys reticulata (Rupr) K. Koch) sprouts harvested from April to June 2011 showed levels [max. 3100 Bq kg−1 fresh weight (FW)] exceeding the limit of 500 Bq kg−1 FW set by the Food Safety Commission of the Japanese government, which is a temporary restriction level for foods sold by farmers. The bamboo trunks harvested from March to June in 2012 and 2013 also contained radioactive cesium (max. 1272 and 469 Bq kg−1 FW, respectively), which exceeded the value of 100 Bq kg−1 FW set as the revised restriction level by the Japanese government in 2012. This report explores differences in the distribution between radioactive cesium and the stable isotope of cesium (133Cs) in bamboo sprouts harvested from a highly contaminated bamboo forest in Iitate village in 2012.
MATERIALS AND METHODS
Sampling of bamboo shoots
We harvested bamboo sprouts [Phyllostachys reticulata (Rupr.) K. Koch: “Madake” in Japanese] 40–50 cm length on August 3, 2011 and July 12, 2012 () from a bamboo forest in Iitate village located 40 km from TEPCO’s Fukushima Daiichi Nuclear Power Plant. The bamboo bush faced the road and many bamboo sprouts were seen several meters from the bush (). The radioactivity at the sampling bamboo forest was measured using a radiation scanner (model 900+; Coliy Technology GmbH) and/or Aloka γ-survey meter (TCS-172). The air-dose rates at a height of 1 m inside the bamboo forest were 6.5–8.0 µSv h−1 in 2011 and 4.5–6.8 µSv h−1 in 2012. The air dose rates 1 cm above the ground inside the bamboo forest were 8.0–8.7 µSv h−1 and 6.7–8.0 µSv h−1 in 2011 and 2012, respectively.
Qualitative autoradiographic studies
The longitudinally or cross-sectioned shoots and branched leaves were exposed to a Fujifilm imaging plate (IP-plate) for about 1 month. Radioactivity was then imaged using an image analyzer (Fujifilm FLA-5000) (, ). A young bamboo sprout about 100 cm tall was isolated, and the radioactivity of the top peel () and the next-to-top peel () of the sprout was imaged. Eight bamboo sprouts were sliced longitudinally or cross-sectioned in the area between 50 and 70 cm from the ground, and these segments were imaged (, ). Radioactivity images of the whole bodies were obtained after exposing tissues to the IP-plate with a plastic film between the tissues and IP-plate to avoid direct contamination from the tissues. Surface skins of 11 bamboo trees randomly selected in the bamboo forest were scraped at 150 cm from the ground using a cutter (NT cutter A300) in 2013, and these skins were exposed as described above.
Figure 2 (a, b) Original material for segmentation and slicing of bamboo shoots harvested in 2011, (c) autoradiograph of (A) longitudinally sliced segments and (B) cross-sectioned segments of bamboo shoots; (C) lateral shoots. White triangles indicate nodal parts.
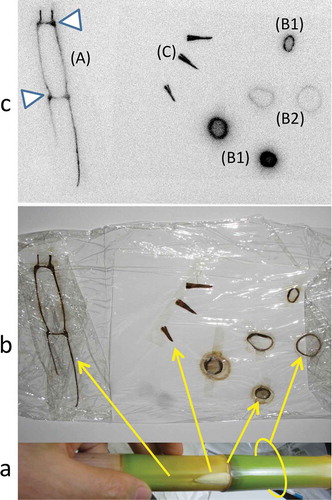
Figure 3 Autoradiograph (b) of newly emerged branched leaves (a) in 2012. Radioactivities of new leaves and new twigs are shown in Table S1.
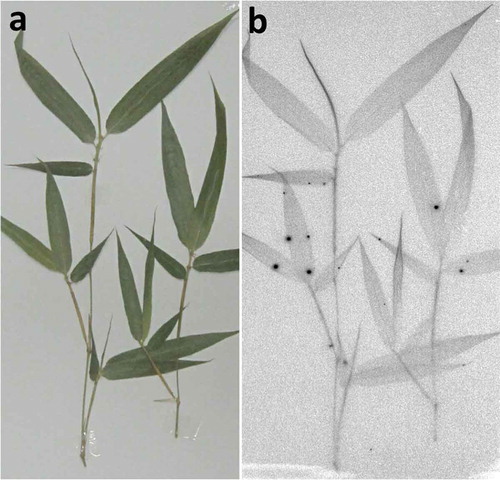
Figure 4 (a) The top leaves (A) and lower peels (B) from a bamboo sprout sampled in 2011. (b) Autoradiographic image of (a). Radioactivities of each part are shown in Table S2.
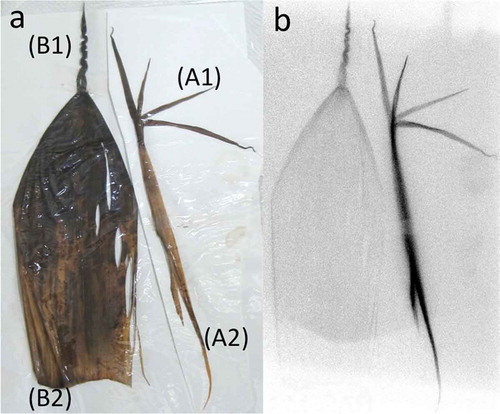
Quantitative radioactivity and mineral element analysis
Each segment of the cross-sectioned bamboo trunk or peel () was dried at 80°C for 2 d, after which the dry weight was measured. The top three peels and nodes were too small to divide, and were therefore treated as the top peel and node, respectively. Each dried segment was then analyzed for 134Cs and 137Cs using a Perkin-Elmer automatic gamma-counter (2480 WIZARD2®). Radioactivity was corrected and shown as the value for the sampling day. Samples of approximately 50 mg dry weight of each bamboo segment were digested with 3 mL of 6 N nitric acid (HNO3) in a Teflon tube at 220°C for 20 min in an ultrahigh throughput microwave wet-type combustion apparatus (CEM MARSXpress), after which the solution was analyzed for potassium (K) and calcium (Ca) using an atomic absorption spectrometer (Shimadzu AA-7000F) and for manganese (Mn), zinc (Zn), copper (Cu) and 133Cs using an inductively coupled plasma - mass spectometry (ICP-MS) spectrometer (Thermo Scientific X Series II ICP-MS).
RESULTS
shows the bamboo bush and many bamboo sprouts (). shows the condensed aerial roots from a bamboo shoot. The longitudinally sliced bamboo shoot contained radioactivity (), lateral buds at the nodal parts accumulated radioactivity (C) and the cross-sectioned nodal parts () showed greater accumulation than the tubular parts (). The newly emerged branches (new twigs and leaves in 2012) from the node of old adult bamboo shoots contained radioactive Cs inside and had several hot spots (, Table S1). These hot spots likely represented fallout scattered from other bamboo shoots by the wind, after which it was redeposited. Bamboo sprouts were harvested and autoradiographic images were obtained. Accumulation of radioactivity in the top peel () and its four small leaf lamina () was stronger than in the lower peel () and its lamina ().
A bamboo sprout was sequentially sectioned into nodes and internodes, and their autoradiographic images were obtained. Nodal parts showed greater accumulation of radioactivity than internodal parts, similar to (B1, B2; ). shows seven longitudinally sectioned bamboo sprouts. The radioactivity formed a concentration gradient from the lower part to the top of the trunk of the sprouts (). Inside the trunk, each node appeared as a ladder. Both trunks and peels contained radioactivity ().
The radioactivity gradient described above based on autoradiographic image analysis was also confirmed by gamma-ray spectrometry. Peels were separated sequentially and the corresponding nodal parts of the trunk were cross-sectioned, as shown in . The radioactivity levels of 134Cs and 137Cs in all separated parts were then measured. The radioactive Cs level in the trunk parts was several times higher than the corresponding peels (). The increasing trend of the radioactivity from the lower parts to the top part was clearly observed in both trunks and peels. The distributions of 133Cs in both trunks and peels were almost constant (100–200 µg kg−1), irrespective of nodal position. Moreover, the concentration of 133Cs in the top peel was very high, and this section may be a strong sink of Cs specific to bamboo species. We compared the distribution pattern in bamboo sprouts between radioactive cesium (134Cs and 137Cs) and stable cesium (133Cs), and the pattern differed between trunks and peels ().
Figure 8 Distributions of radioactive cesium (134Cs + 137Cs, top) and stable cesium (133Cs, bottom) in each node (trunk) and the corresponding peel of a bamboo sprout sampled in 2012.
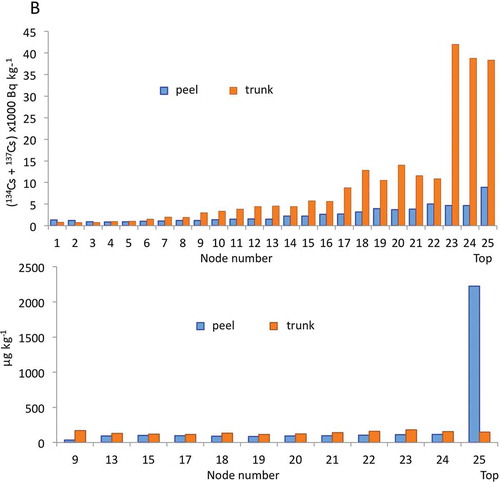
Figure 9 Comparison of the distribution patterns between radioactive cesium (134Cs + 137Cs) and other metals (iron (Fe), zinc (Zn), potassium (K), manganese (Mn), calcium (Ca), cupper (Cu)) in the (a) trunks and (b) peels of a bamboo sprout sampled in 2012. In (b), Zn, Fe, Mn and Cu concentrations are shown on the y-axis on the right side.

We also analyzed the concentrations of other elements, including K, Ca, Mn, iron (Fe), Zn, and Cu, and compared their distribution patterns with the radioactivity (134Cs + 137Cs) in both trunks and peels (). In trunks, the radioactivities of 134Cs and 137Cs increased with trunk number, and the top three trunks contained almost four times higher radioactivity than the others. The concentrations of K, Ca, Mn, Zn, Cu, and 133Cs were stable in all trunks. Only the concentration of Fe showed a gradual increase with trunk number. In peels, the radioactivities of 134Cs and 137Cs increased with peel number. The concentrations of K and Ca reached a plateau in peel number 13. The concentrations of Zn Fe, Mn, and Cu increased gradually. The concentration of 133Cs was almost constant except in the top peel, which showed a markedly higher 133Cs level ().
The skin at 150 cm above the ground of 11 old bamboo shoots in the bush was scraped, autoradiographic images were obtained (, S1) and the amounts of radioactive Cs were measured. Black spots were observed on the outside surface. Radioactivity was also detected on the inside of the skin. Strong 137Cs radioactivity [ranging from 1144 to 28,202 Bq kg−1 dry weight (DW)] was measured 1.5 years after the disaster (Table S3).
Figure 10 (a) Scars after scraping the skin from a bamboo tree in 2013; (b) bamboo skin scraped from bamboo trees; (c) autoradiograph of the bamboo skin. Outside surface (o) and inner side (i) of the skin were exposed to make autoradiographic images. Eleven skin samples from bamboo were used to obtain autoradiographic images and determine the radioactivity levels (Supporting information: Fig. S1, Table S3).
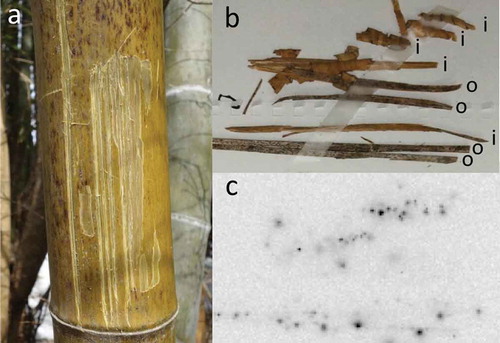
DISCUSSION
After the TEPCO disaster on March 11–20, 2011, bamboo shoots () directly received fallout in the plume through precipitation on the surfaces of their leaves, stalks, branches and shoots (). Water-soluble components of the fallout were absorbed through these tissue surfaces, which may represent the first intrusion into the plant body, such as in vegetables (Mitsui et al. Citation1958), orchard trees (Zehnder et al. Citation1995; Carini et al. Citation1999, Citation2012; Takata et al. Citation2013), and Rhus vernicifera (Stokes) F.A. Barkley (Mori et al. Citation2012). The molecular mechanism of Cs absorption into cells in the top tissues may be similar to the root absorption mechanism, which occurs through K transporters (White and Broadley Citation2000; Broadley et al. Citation2001; Britto and Kronzucker Citation2008; Kanter et al. Citation2010; Kobayashi et al. Citation2010). After the disaster, during the rainy season in Japan, some of the soluble components of the fallout may have moved downward on the surface of bamboo shoots into the litter on the soil surface (Nuclear Regulation Authority Citationn.d.b) or into the surface soils below the litter layer, or could have been absorbed by the condensed aerial roots around the nodes (), which are morphologically specific to bamboo trees (Karizumi Citation2010).
We analyzed the contents of 134Cs + 137Cs and 133Cs to compare their distribution patterns in bamboo sprouts harvested in 2012. We observed clear differences, as shown in and . These results suggested that the “source” of radioactive stable Cs incorporated into the new bamboo sprouts in 2012 was different. We then speculated on the mechanisms for the differences in distribution of radioactive and stable Cs. In general, for a perennial plant such as asparagus (Asparagus officinalis L.), the source of 133Cs in bamboo sprouts may be either the soil or underground sheathes and/or roots. Bamboo roots are tightly entangled under the ground in a bamboo forest (Karizumi Citation2010) and store minerals and organic substrates. When we compared mineral elements in the bamboo trunk, the distribution patterns of K, Ca, Mn, Zn and Cu (but not Fe) were very similar to that of 133Cs, as shown in . The concentrations of these mineral elements were almost constant in all segmented nodal pieces of the trunk, suggesting that a sufficient supply of these minerals in the bamboo trunk was always maintained to meet the rapid growth of bamboo sprouts. However, a slightly different trend was observed for the peels. K and Ca were saturated in the peels at nodal numbers higher than 13, but other heavy metals, Zn, Fe, Mn and Cu, were not yet saturated. Their amounts also showed an increasing trend with increasing nodal number, suggesting that peels at the lower parts were rapidly expanding, which could dilute the concentrations of minerals in peels. Notably, 133Cs in the top peel (probably including the youngest leaf sheath and/or youngest leaf blades) contained high levels of 133Cs (). Radioactive Cs did not show this pattern, although the radioactivity in the top peel was highest (). Movement of 133Cs in a sprout has been suggested to be very rapid, and radioactive Cs can follow the 133Cs trend. The reason why the highest amount of 133Cs is observed in the top of the peel remains unknown. The delayed saturation of Fe in trunks () and the delayed saturation of heavy metals (Fe, Mn, Zn, Cu; ) in peels may be caused by the low velocity of these heavy metals in plants compared with other macroelements, such as K, Ca and nitrogen (N). Transport of heavy metals (such as Fe) in plants is also very low, especially below 20°C (Engels and Marschner Citation1996), which is the temperature just before spring in Japan when bamboo sprouts are rapidly shooting. Mineral transport from the trunks to the peels requires morphologically complicated sieve tubes at the nodes (, white arrows), which may explain why the transport of minerals into peels was low compared to trunks (). This may also explain why higher levels of radioactivity were always detected at the nodes than at the internodes, as seen between B1 and B2 in and .
The main origin of radioactive Cs in the sprouts may differ from that of 133Cs. As discussed above, after the TEPCO disaster in 2011, the radioactive Cs in the fallout may have been absorbed from the tissue surfaces and/or aerial roots and translocated to the various parts of bamboo shoots, where it was stored as a “source” of radioactivity. In spring 2012, the stored radioactivity flowed into the phloem stream in the underground stem and reached the new bamboo sprouts, forming a “sink.”
The particulate deposition on the bamboo shoots may have contained very high levels of radioactive Cs (, S1, Table S3), which was solubilized, absorbed and translocated to the new bamboo sprouts, similar to the situation in 2011. Although fallout adsorbed to the plant tissue surface is difficult to solubilize (Mitsui et al. Citation1958), if only small amounts of the adsorbed radioactive Cs were solubilized with rain, it may be sufficient as a “source” to surpass the restriction level (100 Bq kg−1 FW) in the trunk of a bamboo sprout “sink.” We observed radioactive images with many black dots on the outside surfaces and smear images on the inner side of the scraped bamboo shoots (, S1), which suggested that solubilized radioactivity on the surface of the bamboo shoot may penetrate and/or diffuse into the bamboo tree.
In conclusion, radioactive Cs in bamboo sprouts harvested in 2012 mainly originated from the particulate deposition on various parts of bamboo shoots, while 133Cs originated from the soil and tissues.
Future perspectives
The reason why cultivated plants showed a significant decrease in radioactive Cs levels after 1 year is now partly understood based on many studies of soil science and plant nutrition presented in three successive annual meetings of the Japanese Society of Soil Science and Plant Nutrition. Cultivated plants are typically fertilized with K by farmers, and K+ suppresses radioactive Cs absorption from the roots through competitive inhibition with K+-transporters in the plant root cell membranes. In contrast, wild plants in forests or bushes, which are not supplied with K+-fertilizers, can absorb high levels of radioactive Cs compared to cultivated plants. Therefore, if farmers do not wipe or wash out the old bamboo shoots, as well as sweep out litter and surface soils in the bamboo forests, the production of highly radioactive bamboo sprouts will continue in the future.
SUPPLEMENTARY MATERIAL
Supplementary material for this article is available online from http://dx.doi.org/10.1080/00380768.2014.939936
939936.zip
Download Zip (2.2 MB)ACKNOWLEDGMENTS
We are deeply indebted to Aichi Steel Company for financial funding of this study. We also thank Prof. Naoko K. Nishizawa (Ishikawa Prefectural University) for critical reading of this manuscript, and Prof. Tomoko Nakanishi (The University of Tokyo) for supplying facilities for radioisotope studies to S.M.
REFERENCES
- Britto DT, Kronzucker HJ 2008: Cellular mechanisms of potassium transport in plants. Physiol. Plant., 133, 637–650. doi:10.1111/j.1399-3054.2008.01067.x
- Broadley MR, Escobar-Guetierez AJ, Bowen HC, Willey NJ, White PJ 2001: Influx and accumulation of Cs+ by the akt1 mutant of Arabidopsis thaliana (L.) Heynh. lacking a dominant K+ transport system. J. Exp. Bot., 52, 839–844.
- Brumfiel G 2011: Fallout forensics hike radiation toll. Nature, 478, 435–436. doi:10.1038/478435a
- Carini F, Pellizzoni M, Giosuè S 2012: Radionuclide transfer to fruit in the IAEA TRS No.472. EPJ Web of Conferences, 24, 06002. doi:10.1051/epjconf/20122406002
- Carini F, Scotti IA, DʼAlessandro PG 1999: 134Cs and 85Sr in fruit plants following wet aerial deposition. Health Phys., 77, 520–529. doi:10.1097/00004032-199911000-00005
- Engels C, Marschner H 1996: Effects of suboptimal root zone temperatures and shoot demand on net translocation of micronutrients from the roots to the shoot of maize. Plant Soil, 186, 311–320. doi:10.1007/BF02415526
- Fukushima Prefecture n.d.: Monitoring info. www.new-fukushima.jp/monitoring/en/ (February, 2014).
- Kanter U, Hauser A, Michalke B, Draxl S, Schaffner AR 2010: Caesium and strontium accumulation in shoots of Arabidopsis thaliana: genetic and physiological aspects. J. Exp. Bot., 61, 3995–4009. doi:10.1093/jxb/erq213
- Karizumi N 2010: The Latest Illustrations of Tree Roots (“Saishin jyumoku konkei zusetsu”). Seibundo-shinko-sya, Tokyo. (in Japanese).
- Kobayashi D, Uozumi N, Hisamatsu S, Yamagami M 2010: AtKUP/HAK/KT9, a K+ transporter from Arabidopsis thaliana mediates Cs+ uptake in Escherichia coli. Biosci. Biotechnol. Biochem., 74, 203–205. doi:10.1271/bbb.90638
- Mitsui S, Tensho K, Yen K, Ono K 1958: Investigations on the radioactive contamination of crop plants as a result of nuclear detonation (Part 4): Radioactive contamination of crop plants and soils especially with regard to Sr90 from 1956 to 1957. Jap. J. Soil Sci. Plant Nutri., 29, 109–116. (in Japanese).
- Mori S, Hirato A, Tanoi K, Takeda K, Yamakawa T, Nakanishi H 2012: Radioactive cesium flow in Rhus vernicifera. Soil Sci. Plant Nutri., 58, 611–617. doi:10.1080/00380768.2012.727188
- Nuclear Regulation Authority n.d.a: Preparation of Distribution Map of Radiation Doses, etc. (Map of Radioactive Cesium Concentration in Soil) by MEXT. radioactivity.nsr.go.jp/en/contents/5000/4165/24/1750_083014.pdf (February, 2014).
- Nuclear Regulation Authority n.d.b: Results of the MEXT’s Survey Concerning the Distribution of Radioactive Substances (Survey on the Movement of Radioactive Substances in Forests). radioactivity.nsr.go.jp/en/contents/6000/5668/24/1750_110214e.pdf (February, 2014).
- Takata D, Sato M, Abe K, Yasunaga E, Tanoi K 2013: Radioactivity Distribution of the Fruit Trees Ascribable to Radioactive Fall Out(V)–Transfer of caesium-137 from scion to other organs in ‘Kyoho’ grapes–. Radioisotopes, 62, 455–459. (in Japanese). doi:10.3769/radioisotopes.62.455
- White PJ, Broadley MR 2000: Mechanisms of caesium uptake by plants. Tansley Review No. 113. New Phytol., 147, 241–256. doi:10.1046/j.1469-8137.2000.00704.x
- Zehnder HI, Kopp P, Eikenberg G, Feller JU, Oertli JJ 1995: Uptake and transport of radioactive cesium and strontium into grapevines after leaf contamination. Radiat. Phys. Chem., 46, 61–69. doi:10.1016/0969-806X(94)00115-Z