Abstract
This study investigated the effects of potassium (K) on the behavior of cesium 137 (137Cs) in hydroponically cultured rice plants (Oryza sativa L. “Nipponbare”), revealing that K supply has an influence on reducing 137Cs translocation from leaves to ears as well as decreasing 137Cs uptake by roots. When K was omitted from the culture solution and 137Cs was supplied, the 137Cs content in the ears was increased 6.7-fold compared with the K-sufficient plants, while the total 137Cs content in the shoot part of the K-deficient rice was only 3-fold higher than that in the K-sufficient rice. The fraction of the ears in the total shoot 137Cs content was indeed 2.5 times higher in the K-deficient rice (45%) than that in the K-sufficient rice (18%), demonstrating that K supplementation has an effect on 137Cs translocation to the ears. Irrespective of the K condition, > 95% of the 137Cs that accumulated in the ears was found to be absorbed from the culture solution before ear emergence. K supplementation after ear emergence had an influence on achieving the percentage of 137Cs remobilization in the K-deficient rice close to that in the K-sufficient rice, although the effect on reducing the 137Cs content in the ears could be limited. The results indicate the importance of maintaining an appropriate soil K concentration during the early growth stages to avoid 137Cs contamination of rice grains.
INTRODUCTION
Soil contamination with radionuclides derived from nuclear weapons testing or accidental release from nuclear facilities has a long-term radiological impact on agriculture. After the accident at the Fukushima Daiichi nuclear power plant in 2011, radiocesium (137Cs and 134Cs) was the main artificially produced radionuclide in the east part of Japan, and 137Cs requires special consideration because of its long half-life (30 years). To avoid the contamination of crops, which are the major source of radioactive contamination in the human body (Shaw et al. Citation1994), the mechanisms of 137Cs transportation into rice (Oryza sativa L. “Nipponbare”) is a major concern in Japan.
137Cs distribution in rice plants was studied in Fukushima during the autumn of Citation2011. Then, the rice plants grown in a paddy field where highly contaminated brown rice grains (approximately 500 Bq kg–1) were uncommonly produced were demonstrated to contain higher 137Cs levels in the newer leaves than in the older leaves, which was the opposite of what was found in rice plants grown in a paddy field which produced a grain with very low 137Cs concentration Tanoi et al., Citation2013; Nakanishi and Tanoi Citation2013, and also contrary to a previous report demonstrating that 137Cs concentration was higher in old leaves than new leaves and panicles when grown in an experimental field replicating the typical paddy field (Tsukada et al. Citation2002). Based on these characteristic 137Cs distribution in the rice plant, two hypotheses were conceived that may explain why brown rice containing higher 137Cs concentrations was uncommonly produced in some paddy fields. First, there may have been seasonal variation in the environmental 137Cs concentration, i.e., high 137Cs levels may have been available in the paddy soil during the midsummer when upper leaves grow actively and ears are ready to head up. Indeed, rice plants treated with cesium (Cs) after initial heading had the highest Cs concentration in their ears (Uchida et al. Citation1987). In the summer heat, 137Cs may also have been released during the decomposition of contaminated organic matter (Nemoto and Abe Citation2013). Irrigation water from the mountains may have contained high 137Cs levels, which could have been absorbed by rice plants during summer. The second hypothesis is related to potassium (K) concentration. Cs is a Group I alkali metal with chemical properties similar to those of K. K+ is actively translocated from old tissues to growing tissues if K is deficient (Marschner Citation1995). If Cs+ mimics the movement of K+ inside plants, 137Cs may also accumulate in newer tissues under K-deficient conditions. The paddy soils where the rice had higher 137Cs levels in their newer leaves had extremely low K concentrations because of lack of K fertilization. However, the movements of K and Cs in plants could not be exactly the same. A comparison of Cs and K accumulation in rice, willow (Salix viminalis L.), buckwheat (Fagopyrum esculentum Moench) and millet showed that the Cs:K ratio varied among tissues (Menzel and Heald Citation1955; Gommers et al. Citation2000; Tsukada et al. Citation2002).
We performed a 137Cs tracer experiment using hydroponic rice cultures to analyze the effect of environmental K levels and the 137Cs supply period on 137Cs uptake and transport apart from other environmental causes. It was clearly demonstrated that the K condition before the ear emergence reduced the 137Cs transport to the edible part as well as the uptake of 137Cs. In addition, the effect of the K fertilization after the ear emergence to the 137Cs translocation from leaves to grains was experimentally estimated to consider the cultivation management in the agricultural field.
MATERIALS AND METHODS
Plant materials and growth conditions
Seeds of rice (Oryza sativa L. “Nipponbare”) were germinated in pure water for 4 d and transferred to 0.5 mM calcium chloride (CaCl2) solution. After 2 d, the seedlings were placed in 2-L plastic hydroponic vessels containing modified half-strength Kimura B nutrient solution (pH 5.6) (Tanoi et al. Citation2011) for 2 weeks. Three-week-old rice seedlings were transferred to 300-mL plastic pots with culture solutions that included different K concentrations: 0 mM, 0.27 mM (the K+ concentration in the modified half-strength Kimura B solution) and 3.0 mM (). The 0-mM solution was prepared by replacing KH2PO4, K2SO4, and KNO3 with sodium salts. The 3-mM K solution was prepared by adding KCl to the modified half-strength Kimura B solution. The pots treated with the three K concentrations were designated the experimental plots and three trials were conducted (Experiments A, B and C) (). In Experiment A, non-carrier-added 137Cs was added to the nutrient solution to maintain a final concentration of 9 kBq L–1, which corresponded to 20 pmol L–1 of 137Cs+ ions, throughout the experiment. In Experiment B, rice plants had been grown without 137Cs until the ears started to emerge, when 137Cs (9 kBq L–1) was added. In Experiment C, 137Cs (9 kBq L–1) was added until the heading season, when the solutions in each pot were replaced with 3.0 mM K solution without 137Cs.
Figure 1 Experimental procedures. Plants were grown at 30°C with a 12:12 h light:dark photoperiod. The nutrient solution was changed twice every week. In Experiment A, 3-week-old rice (Oryza sativa L. “Nipponbare”) was transplanted into nutrient solution, which contained Potassium (K) concentrations of either 0 mM, 0.27 mM or 3.0 mM, referred to as LK, MK and HK, respectively, and Cesium 137 (137Cs) was added to maintain the concentration of 9 kBq L–1 until harvesting. In Experiment B, 3-week-old rice was transplanted into solutions with various K concentrations as in Experiment A, while 137Cs (9 kBq L–1) was added only after ear emergence. In Experiment C, rice seedlings were grown in the same way as in Experiment A until ear emergence, when they were transplanted to nutrient solution containing 3.0 mM K, but no 137Cs.
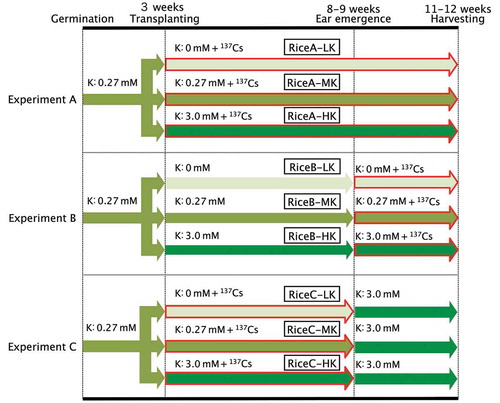
Each rice sample was defined as the “experiment type-K condition”, i.e., pots in Experiment A grown with 0 mM, 0.27 mM and 3.0 mM K were designated “RiceA-LK (low K)”, “RiceA-MK (medium K)” and “RiceA-HK (high K)”, respectively.
The plants were grown at 30°C with a 12:12 h light:dark photoperiod. The nutrient solution was changed twice a week. The rice plants were grown for 12 weeks until the grains matured.
Sampling and 137Cs measurement
The mature rice plants were separated into organs, i.e., leaves, stems and ears. Each rice plant had 10 leaf positions. The leaf blade and sheath were collected separately. The blade/sheath of the nth leaf (“n” indicates an arbitrary ordinal number of leaves counted acropetally from an incomplete leaf on the main stem) was designated L“n”-B/L“n”-S. The newest leaf (10th leaf) was the flag leaf, which was divided into “FL-B” and “FL-S.” If the leaf was a sheath- and blade-integrated organ, the leaf was designated L“n”.
The radioactivity of each leaf, stem and ear was measured using a well-type NaI(Tl) scintillation counter (ARC-300, Aloka, Tokyo, Japan) for 1 minute. All the samples shown in the figures had a sufficient net count, more than 100. The weight of each tissue was measured after drying at 60°C for 1 week.
Ion analysis
For ion analysis, rice plants were grown under the same conditions as those of Experiments A, B and C, except that 137Cs was not added. The sampling procedure was the same as that used for 137Cs, except the ears were divided into brown rice and husks. The tissues were weighed, before 5 mL of nitric acid (6.6 M) was added, followed by heat digestion at 90°C for ≥ 4 h, and low-pressure filtration through 0.2-µm polysulfone membrane filters. Pure water was added to the digest to produce a final volume of 10 mL. The total K, sodium (Na), magnesium (Mg) and calcium (Ca) concentrations in the extracts were determined using an inductively coupled plasma optical emission spectroscopy (ICP-OES; Optima 7300DV, Parkin Elmer), which operated in high-resolution mode.
RESULTS
Plant biomass
In Experiments A and B, 3-week-old rice seedlings were transplanted into culture solutions with varying K concentrations, referred to as LK (low K, 0 mM), MK (medium K, 0.27 mM) and HK (high K, 3.0 mM) (). Leaves older than the seventh leaf (L7), which emerged before transplanting, were designated “old leaves”, whereas the leaves from L7 to flag leaf (FL) were designated “new leaves”. The biomass of LK rice plants at harvesting time was lower than that of MK and HK rice plants, although this difference was not significant (P > 0.05), and the balanced biomass partitioning to the ears, culms, new leaves and old leaves was maintained, regardless of the K concentration supplied ().
Figure 2 Shoot dry weight of each part of the plant grown under three different Potassium (K) conditions in Experiment A and B. Leaves present when Cesium 137 (137Cs) was started to be applied were designated “old leaves” whereas the other leaves were designated “new leaves.” Data are shown as the mean ± SD.
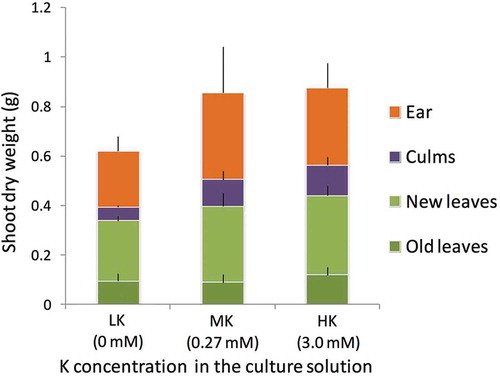
137Cs uptake and distribution in plants
In Experiment A, rice plants were grown with nutrient solution containing 137Cs from vegetative growth until after completely matured (). Then, the total 137Cs accumulation at harvest in RiceA-LK was approximately 3-fold as much 137Cs as RiceA-HK (). This agrees with previous reports on increased Cs absorption under K-deficient conditions (Cline and Hungate Citation1960; Nishita et al. Citation1962; Smolders et al. Citation1996; Zhu et al. Citation2002; Hampton et al. Citation2004). The K level had a significant effect also on the distribution of 137Cs among various plant parts. The amount of 137Cs in RiceA-LK ears made up 45% of the 137Cs amount found in the whole shoot part, whereas 27 and 18% of the total 137Cs were accumulated in RiceA-MK and RiceA-HK ears, respectively (). The synergistic effect of up-regulated 137Cs uptake and the relatively higher percentage of 137Cs translocation toward the newer tissues under K-deficient conditions resulted in a 6.7-fold increase in 137Cs accumulation in the ears of RiceA-LK compared with those of RiceA-HK ().
Figure 3 Cesium 137 (137Cs) accumulation in the various organs of rice plants (Oryza sativa L. “Nipponbare”) grown under three different Potassium (K) conditions from the third week until after harvesting period (Experiments A and B). (a) the amount of 137Cs in each shoot part in Experiment A; (b) the fraction of each shoot part (%) in total shoot 137Cs content in Experiment A; (c) the amount of 137Cs in each shoot part in Experiment B; (d) the fraction of each shoot part (%) in total shoot 137Cs content in Experiment B. The relative contributions of 137Cs absorbed before or after heading season to the total mass of 137Cs accumulated (e) in the above-ground shoots and(f) in the ears and the culms at the time of harvesting were calculated based on (a) and (c). 137Cs absorbed before and after the heading are shown in dark gray and pale gray, respectively. L = leaf, B = blade, S = sheath and FL = flag leaf. The number associated with “L” indicates an arbitrary ordinal number of leaves counted acropetally from an incomplete leaf on the main stem. Data in (a), (b), (c) and (d) are shown as the mean ± SD. For the total 137Cs content in (a) and (c), p-values are shown above the bars. Different letters inside the bars in (a) and (c) indicate that there is a significant difference (p < 0.05, t-test) in the 137Cs content in the ears. In (b) and (d), different letters inside the bars indicate that there is a significant difference (p < 0.05, t-test) in the percentage of the ear fraction.
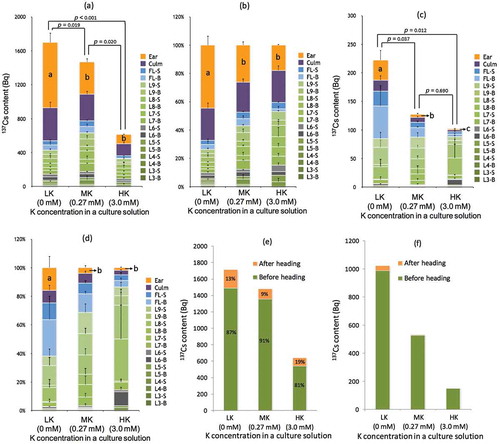
In Experiment B, where 137Cs was applied after the ears emerged (), RiceB-LK contained twice as much 137Cs as RiceB-HK (). The fraction of the ears (%) in the total shoot 137Cs content (the ear fraction) was approximately 10-fold higher in RiceB-LK (16%) than RiceB-HK (1.8%) (). Accordingly, the 137Cs content of RiceB-LK ears increased by 19-fold compared with the amount of 137Cs in the RiceB-HK ears. These results suggested that the low K concentration promoted 137Cs accumulation in the edible parts, regardless of the timing of 137Cs absorption. On the other hand, the fraction of the leaves (%) in the total shoot 137Cs content (the leaf fraction) in K-deficient rice differed depending on the timing of 137Cs absorption. In RiceA-LK, which was exposed to 137Cs from vegetative growth, the 137Cs content was almost the same in FL, L9, L8 and L7. In contrast, in RiceB-LK, which was exposed to 137Cs only after ear emergence, the 137Cs content was higher in the following order: FL > L9 > L8 > L7 ( and ), which was similar to the 137Cs distribution pattern found in the highly contaminated rice plants harvested in Fukushima during Citation2011 (Tanoi et al. Citation2011; Nakanishi and Tanoi Citation2013). These observations suggest that the rice plants having highly contaminated grains were K-deficient, and at the same time they probably received additional 137Cs during the latter half of the growth stages after the summer season.
By subtracting the 137Cs content in RiceB from the 137Cs content in RiceA, we estimated that approximately 80–90% of the total 137Cs accumulated in the mature rice plants had been absorbed before ear emergence (). Mikkelsen and Patrick reported that approximately 75% of the total K absorbed by rice plants throughout the growth period was accumulated before the booting stage and only a small amount was absorbed after panicle initiation (Milkkelsen and Patrick Citation1968). These similar trends in the 137Cs and K absorption periods suggest the high relevance between the uptake systems of 137Cs and K.
Besides, the subtraction of 137Cs content in the ears and culms in RiceB from those in RiceA suggested that 95–98% of the 137Cs contained in the ears and culms (), which grow vigorously after ear emergence, had been absorbed by the roots before ear emergence, and then remobilized to the ears and culms ( and ). In addition, the ear and culm fraction in RiceA () were considerably higher than those in RiceB () despite the fact that RiceB received 137Cs after the flowering season until harvesting, which exactly covered the whole period of brown rice development. Therefore, it could be supposed that suppression of the 137Cs remobilization from the leaves to the grains after panicle initiation may help avoid 137Cs contamination of grains. Because 137Cs uptake by root was shown to be reduced by K fertilization in the present study () as well as in the previous reports (Kaunisto et al. Citation2002; Rosén et al. Citation2011), it was plausible to expect that K fertilization would reduce also 137Cs translocation from leaves to reproductive organs. However, the effect of additional K fertilization in the middle of plant growth stages remains unknown. To investigate the effects of K fertilization on 137Cs translocation from the leaves to the grains, Experiment C was conducted, where plants were grown under three different K conditions with 137Cs until the heading season and then transplanted into K-rich culture solutions without 137Cs (). In this study, RiceC somehow developed tillers with mature ears. Therefore, the results regarding 137Cs amounts in the main stem of RiceC () could not be quantitatively compared with RiceA and RiceB ( and ). The amount of 137Cs found in RiceC-LK ears was 6-fold higher than in RiceC-HK ears (), which is a similar result to RiceA; the amount of 137Cs found in RiceA-LK ears was 6.7-fold higher than RiceA-HK (). The reason for this similarity between RiceA and RiceC can be explained from the data that a large amount of 137Cs was absorbed before the heading period (), while 137Cs absorbed after ear emergence accounted for less than 5% of 137Cs accumulated in the ears and culms at the harvesting period (). These results indicate that K fertilization after heading could not be an effective 137Cs-reduction strategy (). On the other hand, when considering the remobilization from leaves to ears, the similar ear fraction measured in RiceC-MK and RiceC-HK, and even RiceC-LK (), could be supposed to be achieved by the additional K fertilization. On the contrary, the fraction of 137Cs translocation to the ears in 137Cs absorbed until heading would be higher in the K-deficient rice, which was estimated by a simple comparison between RiceA and RiceB ( and ). Thus, additional K fertilization in the heading season was indicated to have some reduction effect on 137Cs remobilization from the leaves to the edible parts, although the effect on reducing the 137Cs content in the grain could be limited.
Figure 4 Cesium 137 (137Cs) accumulation in various parts in the main stem of rice plants (Oryza sativa L. “Nipponbare”) grown under three different Potassium (K) conditions with 137Cs (9 kBq L–1) until ear emergence, followed by 3.0 mM K supplementation (Experiment C) and withdrawal of 137Cs from the culture solution. The amounts of 137Cs accumulated in (a) the ear, culm, new leaves and old leaves of the main stem and (b) the fraction of each shoot part (%) in total 137Cs content in the main stem are shown. Data are shown as the mean ± SD. For the net 137Cs amount in the main stem, p-values are shown above the bars in (a). Different letters inside the bars in (a) indicate that there is a significant difference (p < 0.05, t-test) in the 137Cs content in the ears. In (b), there is no significant difference (p < 0.05, t-test) in the percentage of the ear fraction.
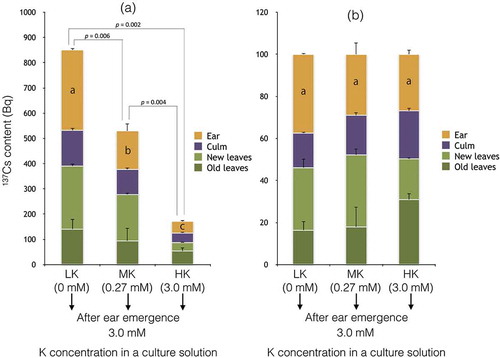
K distribution in plants cultured under various K concentrations
The K concentration in each plant part in RiceA and RiceB was measured to compare the transportation of K and Cs. Then, the K concentrations of leaves and culms were increased with the K concentration in the culture solution. In contrast, the K concentration remained constant in the husks and brown rice at different K concentrations in the culture solution. As a result, K concentration in the LK brown rice (0 mM) increased to 7 to 9 times higher than that in the old and newer leaves, while the K concentration in the brown rice was only 25–50% of that in the leaves of HK plants (3 mM) (). These data suggest that rice plants could up- or down-regulate K transportation into brown rice in response to the external/internal K conditions. The K-deficient rice, i.e., RiceA-LK and RiceB-LK, could not absorb K from the nutrient solution after the third week of germination, so K was probably remobilized from the leaves to brown rice to maintain K homeostasis. Because the Na concentration was increased by omitting K from the culture solution (Fig. S1), it is probable that Na ions play some part of the role of K to keep the rice growth.
Figure 5 The Potassium (K) concentrations in rice plants (Oryza sativa L. “Nipponbare”) grown under three different K conditions (Experiments A and B). The K concentration was maintained in the brown rice and the husks whereas the leaves had significantly different K concentrations, depending on the K concentration in the nutrient solution. Older leaves comprised the leaves present at the time of transplanting. Newer leaves comprised leaves that emerged after transplanting. Data are shown as the mean ± SD. Different letters above the bars indicate that there is a significant difference (p < 0.05, t-test) between the different K conditions.
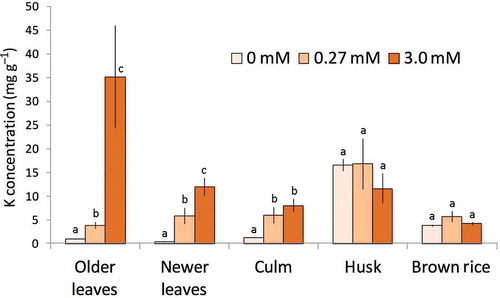
In the LK rice, both K and 137Cs concentrations tended to be higher in the new organs including the flag leaves, culms and ears ( and Fig. S2), suggesting the large contribution of the translocation process to K and 137Cs transportation in K-deficient rice. The 137Cs tracer experiment also showed that the newer leaves contained more 137Cs when plants were grown under K-deficient conditions even in 2-week-old rice (Fig. S3), which suggests that active 137Cs translocation was accompanied by K translocation, regardless of the growth stage.
137Cs:K ratio
The 137Cs:K concentration ratio of each organ was calculated for Experiment A to compare the transport mechanisms of 137Cs and K in the shoots (). The ratio of the Cs to K concentrations (RCsK) was defined as follows.
Figure 6 Cesium (Cs): potassium (K) concentration ratios [RCsK; 137Cs (Bq) K–1 (mg–1)] in rice plants (Oryza sativa L. “Nipponbare”) supplied with (a) LK (0 mM), (b) MK (0.27 mM) and (c) HK (3.0 mM). The RCsK of brown rice was normalized against the RCsK of the total leaves, i.e., the “relative RCsK” (d). L = leaf, B = blade, S = sheath and FL = flag leaf. The number associated with “L” indicates an arbitrary ordinal number of leaves counted acropetally from an incomplete leaf on the main stem. Data are shown as the mean ± SD.
![Figure 6 Cesium (Cs): potassium (K) concentration ratios [RCsK; 137Cs (Bq) K–1 (mg–1)] in rice plants (Oryza sativa L. “Nipponbare”) supplied with (a) LK (0 mM), (b) MK (0.27 mM) and (c) HK (3.0 mM). The RCsK of brown rice was normalized against the RCsK of the total leaves, i.e., the “relative RCsK” (d). L = leaf, B = blade, S = sheath and FL = flag leaf. The number associated with “L” indicates an arbitrary ordinal number of leaves counted acropetally from an incomplete leaf on the main stem. Data are shown as the mean ± SD.](/cms/asset/93a16c06-995e-4d82-aea3-03d7213469fb/tssp_a_947617_f0006_b.gif)
Different RCsK values have been previously reported in rice plant tissues (Tsukada et al. Citation2002), wheat (Triticum aestivum L.) tissues (Smolders and Shaw Citation1995), grass (Menzel and Heald Citation1955) and trees (Gommers et al. Citation2000; Robison et al. Citation2009). Tsukada et al. (Citation2002) also reported low RCsK values in rice grains. On the other hand, RCsK values in tissues were almost uniform in some tropical plants (Staunton et al. Citation2003; Sanches et al. Citation2008), olive (Olea europaea) and citrus trees (Skarlou et al. Citation1999) and other crops (Gerzabek et al. Citation1998).
For each treatment, the RCsK of brown rice was normalized against that of the total leaves, i.e., the “relative RCsK” (). The RCsK of RiceA-HK brown rice was approximately 60% of the RCsK of the leaves. Furthermore, the RCsK of RiceA-LK brown rice was 20% of that of the leaves. These results indicate that K was transported preferentially compared with Cs, especially under K-deficient conditions, via translocation from the leaves to brown rice, which is the essential pathway for 137Cs to reach rice grains.
DISCUSSION
This study demonstrated the influence of K on 137Cs translocation in rice shoots, as well as on 137Cs uptake by roots. Maintaining an adequate external K concentration reduced the total uptake amount of 137Cs and its distribution to the edible parts. These two effects of K are supposed to be the mechanism responsible for the decrease in radiocesium contamination of brown rice grains when an appropriate K fertilization is applied in paddy fields. We tested whether additional K fertilization in the heading season may suppress 137Cs accumulation in brown rice (Experiment C). However, when plants were grown under K-deficient conditions until the heading season, the 137Cs levels in the edible parts was still higher as a consequence of copious accumulations of 137Cs before fertilization (). Thus, K fertilization should be applied especially during the early growth stages, which is actually a guideline for radiocesium-contaminated fields that was recently provided by the Japanese Ministry of Agriculture, Forestry, and Fisheries. This situation contrasts with that observed with cadmium (Cd), another pollutant in the field. In rice plants, approximately 40% of the final Cd accumulation in grains is absorbed after the heading season (Rodda et al. Citation2011), so it is important to take measures during the heading season to reduce Cd contamination. In this regard, the Cd-polluted paddy soil is guided to be flooded around the heading period so that Cd remains in a reduced form, which is insoluble and is barely absorbed by plants.
During uptake via the roots, Cs may be transported via a K-like dual-affinity system, i.e., a low-affinity uptake system that works constitutively and a high-affinity uptake system induced by K deficiency (Bange and Overstreet Citation1960; Shaw and Bell Citation1989; White and Broadley Citation2000). KAT1, a low-affinity K channel, had low Cs selectivity in oocyte experiments (Schachtman et al. Citation1992). TaHKT2;1 and AtHAK5 are high-affinity K transporters in the roots of wheat and Arabidopsis, respectively, which efficiently transport Cs during K deficiency (Schachtman and Schroeder Citation1994; Qi et al. Citation2008). Moreover, Cs+ uptake is strongly suppressed by K+ under K-deficient conditions (Shaw and Bell Citation1991; Shaw et al. Citation1992; Zhu and Shaw Citation2000). Therefore, the increased Cs uptake under K-deficient conditions ( and c) may be partly explained by the function of the high-affinity K transport system.
Understanding the Cs distribution in the upper parts of plants is an important part of a study to cope with radiocesium contamination of grains, but the molecular mechanisms of Cs transportation remain unknown. According to the present study, regardless of K levels in culture solution, 95–98% of the 137Cs in the ears and culms at harvest time had been stored in the leaves before ear emergence and then translocated to the ears and culms ( and ). Thus, understanding the mechanisms of Cs translocation and suppressing 137Cs transportation in rice plants will be of significant value.
When plants were cultured under LK (0 mM), the RCsK values of the newer organs, such as brown rice, were expected to be higher than those of the older leaves, because 137Cs, but not K, could be taken up by the roots and transported to the newer organs. In contrast with our expectations, however, a lower RCsK value was found in brown rice of RiceA-LK (). Thus, under K-deficient conditions, the highly efficient movement of K relative to 137Cs may have occurred during leaf-to-brown rice translocation, which was probably because the K transport system induced under K deficient conditions, if any, had a lower permeability for 137Cs. This result suggests that the mechanism by which K is translocated from the leaves to brown rice differed from that observed in the high-affinity K transport system found in the roots, which had higher permeability for 137Cs (Bange and Overstreet Citation1960; Shaw and Bell Citation1989; White and Broadley Citation2000).
Arabidopsis mutants lacking one of the genes of the AtCNGC family, which encodes the voltage insensitive cation channels (VICCs), had a distinct RCsK in their shoots (Hampton et al. Citation2005). Mutants lacking some AtCNGCs had lower shoot Cs concentrations, whereas mutants lacking other AtCNGCs accumulated more Cs in their shoots. Most of the mutants maintained the same K concentration in their shoots. This indicates that there may be a relationship between the specific properties of the K transport system and the Cs distribution pattern, which could lead to variation in the RCsK values among tissues.
The detailed mechanisms of Cs translocation in the shoots, which could be regulated in response to K, remain unclear. Various K channels have been identified in plant leaf cells but not all of these efficiently transport Cs and some are blocked by Cs (Heidrich and Dietrich Citation1996). A high-affinity K transporter that efficiently transports Cs has been identified in root cells but not in the aboveground plant parts (Zhu and Smolders Citation2000).
We suggest two different ion transport pathways to brown rice: (1) Cs is transported from roots to leaves but can be remobilized via the phloem to the grain and (2) Cs is transported from roots to the aboveground parts via the xylem, and xylem-to-phloem transfer carries Cs into the brown rice without passing through the leaves. The results presented here demonstrate the principal contribution of the first pathway (1), on which the K concentration can have an influence. Xylem-to-phloem transfer in the rachis or head is a major transport mechanism into the grains of cereals for micronutrients such as Cd (cadmium), Zn (zinc) and Mn (manganese) (Wolswinkel Citation1999). In wheat, the xylem plays an important role in transporting Zn and Mn to spikelets, although the phloem is the point of entry into the caryopsis (Pearson et al. Citation1995, Citation2008). The present study was not conducted to evaluate the significance of the second pathway (2) in Cs transport, but the relatively low level in the ear fraction and the culm fraction in RiceB () could give the idea that the xylem-to-phloem transfer pathway has a minor function in Cs transport into the rice grain.
Supplementary Material
Supplemental data (Figure S1 to S3) published online alongside this article at http://dx.doi.org/10.1080/00380768.2014.947617
Supplemental Materials
Download MS Power Point (375.6 KB)REFERENCES
- Bange GG, Overstreet R 1960: Some observations on absorption of cesium by excised barley roots. Plant. Physiol., 35, 605–608. doi:10.1104/pp.35.5.605
- Cline JF, Hungate FP 1960: Accumulation of potassium, cesium and rubidium in bean plants grown in nutrient solutions. Plant Physiol., 35, 826–829. doi:10.1104/pp.35.6.826
- Gerzabek M, Strebl F, Temmel B 1998: Plant uptake of radionuclides in lysimeter experiments. Environ. Pollut., 99, 93–103. doi:10.1016/S0269-7491(97)00167-X
- Gommers A, Thiry Y, van den Hove H, Vandecasteele CM, Smolders E, Merckx R 2000: Radiocesium uptake by one-year-old willows planted as short rotation coppice. J. Environ. Qual., 29, 1384–1390. doi:10.2134/jeq2000.00472425002900050003x
- Hampton CR, Bowen HC, Broadley MR, Hammond JP, Mead A, Payne KA, Pritchard J, White PJ 2004: Cesium toxicity in Arabidopsis. Plant Physiol., 136, 3824–3837. doi:10.1104/pp.104.046672
- Hampton CR, Broadley MR, White PJ 2005: Short review: the mechanisms of radiocaesium uptake by Arabidopsis roots. Nukleonika, 50, S3–S8.
- Hedrich R, Dietrich P 1996: Plant K+ channels: similarity and diversity. Bot. Acta., 109, 94–101. doi:10.1111/j.1438-8677.1996.tb00548.x
- Kaunisto S, Aro L, Rantavaara A 2002: Effect of fertilization on the potassium and radiocaesium distribution in tree stands (Pinus sylvestris L.) and peat on a pine mire. Environ. Pollut., 117, 111–119. doi:10.1016/S0269-7491(01)00153-1
- Marschner H 1995: Mineral Nutrition of Higher Plants. 2nd edn. Academic Press, London.
- Menzel RG, Heald WR 1955: Distribution of potassium, rubidium, cesium, calcium, and strontium within plants grown in nutrient solutions. Soil Sci., 80, 287–294. doi:10.1097/00010694-195510000-00006
- Mikkelsen DS, Patrick WH 1968: Fertilizer use on rice. In Changing Patterns of Fertilizer Use, Ed. Nelson, LB, pp. 403–432. SSSA, Madison.
- Nakanishi TM, Tanoi K, Eds. 2013: Agricultural Implications of the Fukushima Nuclear Accident. Springer. doi:10.1007/978-4-431-54328-2
- Nemoto K, Abe J 2013: Radiocesium absorption by rice in paddy field ecosystems. In Agricultural Implications of the Fukushima Nuclear Accident, Eds. Nakanishi TM. Tanoi K, pp. 19–27. Springer. doi:10.1007/978-4-431-54328-2
- Nishita H, Dixon D, Larson KH 1962: Accumulation of Cs and K and growth of bean plants in nutrient solution and soils. Plant Soil, 17, 221–242. doi:10.1007/BF01376226
- Pearson JN, Rengel Z, Jenner CF, Graham RD 1995: Transport of zinc and manganese to developing wheat grains. Physiol. Plantarum., 95, 449–455. doi:10.1111/j.1399-3054.1995.tb00862.x
- Pearson JN, Rengel Z, Jenner CF, Graham RD 2008: Manipulation of xylem transport affects Zn and Mn transport into developing wheat grains of cultured ears. Physiol. Plantarum., 98, 229–234. doi:10.1034/j.1399-3054.1996.980202.x
- Qi Z, Hampton CR, Shin R, Barkla BJ, White PJ, Schachtman DP 2008: The high af finity K+ transporter AtHAK5 plays a physiological role in planta at very low K+ concentrations and provides a caesium uptake pathway in Arabidopsis. J. Exp. Bot., 59, 595–607. doi:10.1093/jxb/erm330
- Robison WL, Brownb PH, Stonec EL, Hamiltona TF, Conradoa CL, Kehla S 2009: Distribution and ratios of 137Cs and K in control and K-treated coconut trees at Bikini Island where nuclear test fallout occurred: effects and implications. J. Environ. Radioactiv., 100, 76–83. doi:10.1016/j.jenvrad.2008.10.016
- Rodda M, Li G, Reid R 2011: The timing of grain Cd accumulation in rice plants: the relative importance of remobilisation within the plant and root Cd uptake post-flowering. Plant Soil, 347, 105–114. doi:10.1007/s11104-011-0829-4.
- Rosén K, Vinichuk M, Nikolova I, Johanson K 2011: Long-term effects of single potassium fertilization on 137Cs levels in plants and fungi in a boreal forest ecosystem. J. Environ. Radioactiv., 102, 178–184. doi:10.1016/j.jenvrad.2010.11.009
- Sanches N, Anjos RM, Mosquera B 2008: 40K/137Cs discrimination ratios to the aboveground organs of tropical plants. J. Environ. Radioactiv., 99, 1127–1135. doi:10.1016/j.jenvrad.2008.01.003
- Schachtman DP, Schroeder JI 1994: Structure and transport mechanism of a high-affinity potassium uptake transporter from higher plants. Nature, 370, 655–658. doi:10.1038/370655a0
- Schachtman DP, Schroeder JI, Lucas WJ, Anderson JA, Gaber RF 1992: Expression of an inward rectifying potassium channel by the Arabidopsis KAT1 cDNA. Science, 258, 1654–1658. doi:10.1126/science.8966547
- Shaw G, Bell JNB 1989: The kinetics of caesium absorption by roots of winter wheat and the possible consequences for the derivation of soil-to-plant transfer factors for radiocaesium. J. Environ. Radioactiv., 10, 213–231. doi:10.1016/0265-931X(89)90026-X
- Shaw G, Bell JNB 1991: Competitive effects of potassium and ammonium on caesium uptake kinetics in wheat. J. Environ. Radioactiv., 13, 283–296. doi:10.1016/0265-931X(91)90002-W
- Shaw G, Bell JNB 1994: Plants and radionuclides. In Plants and Chemical Elements: Biochemistry, Uptake, Tolerance and Toxicity, Ed. Farago ME, pp. 179–220. VCH, Germany.
- Shaw G, Hewamanna R, Lillywhite J, Bell JNB 1992: Radiocaesium uptake and translocation in wheat with reference to the transfer factor concept and ion competition effects. J. Environ. Radioactiv., 16, 167–180. doi:10.1016/0265-931X(92)90014-K
- Skarlou V, Nobeli C, Anoussis J, Haidouti C, Papanicolaou E 1999: Transfer factors of 134Cs for olive and orange trees grown on different soils. J. Environ. Radioactiv., 45, 139–147. doi:10.1016/S0265-931X(98)00098-8
- Smolders E, Kiebooms L, Buysse J, Merckx R 1996: 137Cs uptake in spring wheat (Triticum aestivum L. cv. Tonic) at varying K supply. I. The effect in solution culture. Plant Soil, 181, 205–209. doi:10.1007/BF00012054
- Smolders E, Shaw G 1995: Changes in radiocaesium uptake and distribution in wheat during plant development: a solution culture study. Plant Soil, 176, 1–6. doi:10.1007/BF00017669
- Staunton S, Hinsinger P, Guivarch A, Brechignac F 2003: Root uptake and translocation of radio- caesium from agricultural soils by various plant species. Plant Soil, 254, 443–455. doi:10.1023/A:1025584016465
- Tanoi K, Kobayashi NI, Ono Y, Fujimura S, Nakanishi TM, Nemoto K 2013: Radiocaesium distribution in rice plants grown in the contaminated soil in Fukushima prefecture in 2011. Radioisotopes, 62, 25–29. doi:10.3769/radioisotopes.62.25
- Tanoi K, Saito T, Iwata N, Kobayashi NI, Nakanishi TM 2011: The analysis of magnesium transport system from external solution to xylem in rice root. Soil Sci. Plant Nutr., 57, 265–271. doi:10.1080/00380768.2011.576397
- Tsukada H, Hasegawa H, Hisamatsu S, Yamasaki S 2002: Rice uptake and distributions of radioactive 137Cs, stable 133Cs and K from soil. Environ. Pollut., 117, 403–409. doi:10.1016/S0269-7491(01)00199-3
- Uchida S, Sumiya, M., Yokosuka, S., Ohmomo, Y. 1987: Transfer of radionuclides to crop plants through roots-radiocesium and strontium. Radioisotopes, 36, 575–580. (in Japanese). doi:10.3769/radioisotopes.36.11_575
- White PJ, Broadley MR 2000: Tansley Review No. 113. Mechanisms of caesium uptake by plants. New Phytol., 147, 241–256. doi:10.1046/j.1469-8137.2000.00704.x
- Wolswinkel P 1999: Long-distance nutrient transport in plants and movement into developing grains. In Mineral Nutrition of Crops: Fundamental Mechanisms and Implications, Ed. Rengel Z, pp. 91–113. Food Products Press, New York.
- Zhu YG, Shaw G 2000: Soil contamination with radionuclides and potential remediation. Chemosphere, 41, 121–128. doi:10.1016/S0045-6535(99)00398-7
- Zhu YG, Shaw G, Nisbet AF, Wilkins BT 2002: Effect of external potassium supply and plant age on the uptake of radiocaesium (137Cs) by broad bean (Vicia faba): interpretation of results from a large-scale hydroponic study. Environ. Exp. Bot., 47, 173–187. doi:10.1016/S0098-8472(01)00124-1
- Zhu YG, Smolders E 2000: Plant uptake of radiocaesium: a review of mechanisms, regulation and application. J. Exp. Bot., 51, 1635–1645. doi:10.1093/jexbot/51.351.1635