Abstract
Pot and field experiments were conducted to clarify the effect of soil exchangeable potassium (K) and cesium-137 (137Cs) on 137Cs accumulation and to establish soil index in rice (Oryza sativa L.). Four paddy soils in Fukushima Prefecture, Japan, showing different transfer factors for radioactive Cs derived from the accident of Fukushima Daiichi Nuclear Power Station in the field were compared in terms of 137Cs accumulation in rice in a pot experiment. 137Cs accumulation in shoots and brown rice widely varied among soils with the transfer factor ranging from 0.018 to 0.068 for shoots and 0.004 to 0.065 for brown rice. 137Cs concentration in brown rice and shoots tended to decrease with higher levels of soil exchangeable K, and they were more closely related to the exchangeable Cs/K ratio. Similar relationships between the Cs/K ratio and Cs accumulation in plants were obtained for the stable isotope cesium-133 (133Cs). The distributions of 137Cs and 133Cs in grains were also similar and variable among soils. The transfer factors obtained in pot experiments mostly agreed with field observations. The results imply that the exchangeable 137Cs/K can be a potential soil index to estimate 137Cs accumulation in rice.
INTRODUCTION
Following the Fukushima Daiichi Nuclear Power Station accident in Fukushima Prefecture, Japan, in March 2011, the prevention of radioactive cesium (Cs) accumulation in crops became a critical issue (Miyashita Citation2012; Mimura et al. Citation2014). A survey of rice in Fukushima Prefecture and surrounding areas showed that the radioactive Cs concentration in rice grain varied greatly among the fields and locations. In some locations in northern Fukushima Prefecture, high cesium-137 (137Cs) and cesium-134 (134Cs) concentrations, that exceeded the provisional guidelines, were observed in brown rice (Saito et al. Citation2012). To establish effective measures for controlling radioactive Cs concentration in rice, it is essential to identify soil properties and other factors affecting the variation in radioactive Cs accumulation.
Several reports have shown that soil chemical and physical properties significantly affect Cs uptake (reviewed by Yamaguchi et al. Citation2012). Notably, potassium (K) is known to be a strong counter-element for Cs uptake by rice and other plants (Tsumura et al. Citation1984; Zhu and Smolders Citation2000) because of the similar chemical and physical behaviors of K and Cs. Cs uptake in plant roots is primarily conveyed by the K uptake system (White and Broadley Citation2000). K application has been effective in lowering Cs concentrations in rice (Tsumura et al. Citation1984). Recent reports on rice in the fields in Fukushima and other affected prefectures clearly showed that the transfer factor (TF) from soil to brown rice decreased with increasing exchangeable K (Kato Citation2012; Saito et al. Citation2012). On the other hand, exchangeable K could not totally explain the variation in TF among soils, such as in a soil with high vermiculite (Kato Citation2012), which signifies the possibility that soil Cs fixation ability affects Cs uptake. It is reported that soil with high exchangeable Cs tended to enhance Cs uptake in rice (Tsumura et al. Citation1984). This indicates that soil Cs availability is also a significant factor to determine Cs uptake. Therefore, it is important to precisely understand the effect of soil K availability in combination with other soil properties such as Cs availability on Cs accumulation in rice under paddy conditions. Under field conditions, factors other than indigenous soil properties such as climatic conditions, cultivation method, irrigation and fallout from the atmosphere may confound Cs accumulation in plants. Therefore, experiments with controlled conditions are required to exclusively examine the effect of indigenous soil factors, particularly with regard to the availability of K and Cs.
The aim of this study is to clarify the effect of soil exchangeable K and 137Cs on the accumulation of 137Cs in brown rice and shoots in rice, and to establish a soil index for the estimation of 137Cs concentrations in the plant using exchangeable K and 137Cs. The accumulation and distribution of the stable isotope cesium-133 (133Cs) in the plant were also determined to examine similarities between 133Cs and 137Cs. The results obtained in pot experiments under controlled conditions were compared with field observations.
MATERIALS AND METHODS
Soils
Paddy soils were collected from four locations, Ohisa in Iwaki City (Typic Fluvaquents), Hiwada in Koriyama City (Typic Fluvaquents), Yanagawa in Date City (Aeric, Typic Epiaquults) and Obama in Nihonmatsu City (Typic Fluvaquents), in Fukushima Prefecture, Japan, for use in pot experiments. Soils were collected at the plow layer depth in November to December 2011 after the harvest of rice in the 2011 cropping season. The soil was air-dried and sieved before being used in the pot experiments. The basic chemical and physical properties of the soils are shown in . The clay content was lowest in the soils from in Nihonmatsu and highest in those from Date.
Table 1 Basic properties of soils used in the pot experiment
Soil analysis
Cs and K in the soils were analyzed before the pot experiment. For the determination of total 137Cs and 134Cs, soil samples were placed in a 2-L Marinelli beaker and analyzed using a germanium semiconductor detector (GX5520, Canberra Co., USA). The counting time was 301–4680 s. Total 133Cs was determined on the basis of the method reported by Hossener (Citation1996), with slight modifications. Soil (0.5 g) was placed in Teflon tubes, to which 7 mL of nitric acid (HNO3), 2.5 mL of hydrofluoric acid (HF) and 0.5 mL of hydrogen peroxide (H2O2) were added. The soil was digested using a microwave concentration rotor (ETHOS PLUS, Milestone Co., Italy) for 20 min at a maximum temperature of 210°C. After cooling, 1 mL of HNO3 and 2 mL of perchloric acid (HClO4) were added and it was again heated using a microwave up to 230°C for 20 min. The 133Cs in the digest was determined by Inductively coupled plasma - mass spectrometry (ICP-MS) (ELAN DRC-E, ParkinElmer Co., USA). Exchangeable K, 133Cs, 137Cs and 134Cs were determined by extracting soil with 1 M CH3COONH4 (soil:solution = 1:10). 137Cs and 134Cs concentrations in the extracted ammonium acetate (CH3COONH4) solution were determined using a germanium semiconductor (GC2020, GC2520, GC4020, Canberra Co., USA) in a 2-L Marinelli beaker. The counting time was 1835–30,000 s, and counting errors for 137Cs and 134Cs measurement were less than 10%.
Clay mineralogy
The soils were oxidized with sodium hypochlorite (pH 8.0, room temperature; Mikutta et al. Citation2005) and subsequently dispersed by ultrasonic vibration and sodium (Na)-saturated resin (Bartoli et al. Citation1991). Clay fractions (< 2 μm) were quantitatively recovered by sedimentation. Clay mineral composition was assessed with an X-ray diffractometer (SmartLab, Rigaku co., Japan). Clay fractions were treated with dithionite–citrate–bicarbonate (Mehra and Jackson Citation1960) and dark oxalate (Higashi and Ikeda Citation1974). Clay fractions were subsequently saturated with magnesium (Mg) or K using a chloride salt. Mg-saturated clay fractions were assessed before and after glycerol solvation. K-saturated clay fractions were assessed before and after heating to 300 and 550°C.
Growing conditions
Rice (O.sativa L, cv. Hitomebore) was grown in a plastic Wagner pot (1/5000 a) containing 2.4 kg of soil. Three 21-d-old seedlings were transplanted and grown under submerged conditions until maturity. Pots were placed in a temperature-controlled chamber with artificial light during 0–45 and 61–120 days after transplanting (DAT) and in a greenhouse with naturally lit conditions during 46–60 DAT. Daily mean temperature was 23.5, 24.6 and 24.1°C during 0–45, 46–60 and 61–120 DAT, respectively. Photosynthetically active radiation (PAR) was adjusted at 300 and 800 μmol m−2 s−1 during 0–45 and 61–120 DAT in the chamber.
Nitrogen (N) ((NH4)2SO4), phosphorus (P) (single super phosphate), and K potassium chloride (KCl) fertilizers were basally applied by mixing with soil before transplanting at 0.5 g N pot−1, 0.11 g P pot−1 and 0.21 g K pot−1, respectively. N was top-dressed at 40 DAT with (NH4)2SO4 at 0.4 g N pot−1. The experiment was conducted with six replications.
Soil solution
Soil solution was sampled using a porous cup (3 mm in diameter and 10 cm in length; Nitto Kagakau Co., Japan; Toriyama and Ishida Citation1987). K concentration in the soil solution was analyzed using ion chromatography (DX-320J, Dionex Co., USA) equipped with separation column for cations (IonPac CS12A, Dionex Co., USA). The elution solution used was 20 mmol l−1 methanesulfonic acid.
Plant analysis after the harvest
The aboveground plant parts were sampled on 75 DAT at around panicle initiation stage (PI) and on 120 DAT at maturity. Samples were separated into shoots (stems and leaves), brown rice, husk and rachis. Samples were dried at 70°C, and the dry weight was determined. Samples were subsequently ground using a grinding mill (WSX-200, Fujiwara Scientific Co. Japan).
To determine 137Cs and 134Cs concentrations in brown rice and shoots, a sample in a styrene U-8 beaker (100 mL) was analyzed using a germanium semiconductor detector (GC2519, Canberra Co., USA). The counting time was 23,212–227,349 s. At the time of plant sampling, soil was also sampled from each pot and analyzed for 137Cs and 134Cs using the method described above.
137Cs TF was calculated as follows:
For elemental analysis of 133Cs in brown rice and shoots, samples were further finely ground using a milling machine (TI-100, Fujiwara Scientific Co. Japan), and 20 mg of each sample were digested with HNO3. The concentration of 133Cs in the digest was determined by ICP-MS (ELAN DRC Plus, Perkin Elmer Co. USA). All elemental concentrations in plants and soil are expressed on a dry weight basis.
Field observation
At the four locations where soil was sampled, 137Cs concentrations in brown rice and the soils’ plow layers were determined after the harvest in the 2011 cropping season. The rice cultivar was ‘Hitomebore’ in Nihonmatsu and ‘Koshihikari’ in the other three sites. Fertilizer application rates (g m−2) of N, P and K were 4.20, 1.83 and 3.49, respectively, in Iwaki; 4.00, 3.49 and 6.64, respectively, in Koriyama; 6.8, 2.09 and 5.65, respectively, in Date; and 5.20, 5.59 and 3.32, respectively, in Nihonmatsu. The brown rice samples were subsampled from the bulk samples harvested by farmers. Brown rice samples in styrene U-8 beakers (100 mL) were analyzed for 137Cs using a germanium semiconductor coupled to a multichannel analyzer (GC3020, Canberra Co., USA) at Fukushima Agricultural Technology Centre. The counting time was approximately 1800 s. Soil samples were collected from five points in one paddy field at a depth of 0–15 cm using a core sampler (HS-30, Fujiwara Scientific Co. Japan) in December 2011. 137Cs in the soil was analyzed using a styrene U-8 beaker (100 mL) and a germanium semiconductor detector (GMX30, Ortec Co., USA) at Kyushu Environmental Evaluation Association. The counting time was approximately 1000 s. TF from soil to brown rice was calculated as mentioned above.
Statistical analysis
All data were subjected to analysis of variance (ANOVA), and the differences among soils were compared using Tukey’s multiple range test (P < 0.05) using the statistical software Statistix (ver 9, Analytical Software Co. USA).
RESULTS
Soil Cs and clay mineralogy
Soil properties for Cs and K are presented in . Total concentrations of 134Cs and 137Cs varied from 379–1555 Bq kg−1 and from 487–2055 Bq kg−1, respectively. Exchangeable K varied with the highest value in Koriyama and the lowest value in Nihonmatsu. The fraction of exchangeable 137Cs in total 137Cs varied from 2.8% in Koriyama to 20.4% in Iwaki. The fraction of exchangeable 133Cs in total 133Cs varied among soils in an order similar to 137Cs and 134Cs.
Table 2 Soil properties of Cs and K
The characteristics of the soil clay mineralogy are shown in . All the soils contained kaoline as a major clay mineral. Soils from Iwaki did not contain vermiculite while those from Koriyama, Date and Nihonmatsu contained vermiculite to some extent, although it was somewhat interlayered with hydroxyl aluminum (Al).
Table 3 Comparison of clay mineralogy of soils
Plant growth and Cs uptake
For radioactive Cs, only the results for 137Cs are presented because those for 134Cs showed trends similar to the results for 137Cs. Plant growth in terms of aboveground dry weight and tiller number were not significantly different among soils at PI (). At maturity, aboveground dry weight was lowest in the soils from Nihonmatsu, with the lowest dry weight of brown rice being associated with a low ripening rate.
Table 4 Plant growth at panicle initiation stage (PI) and maturity in the pot experiment
137Cs concentrations in shoots and brown rice at PI and maturity are shown in . 137Cs concentration in shoots was highest in the soils from Nihonmatsu at both stages. 137Cs concentration in brown rice was also highest in the soils from Nihonmatsu, followed by those from Iwaki and Date. Soils from Koriyama showed the lowest 137Cs in brown rice.
Relationship of 137Cs accumulation in plants with soil K and Cs
The relationship between 137Cs concentration in plants and the exchangeable form and total amount of 137Cs in soil are shown in and , respectively. 137Cs concentration in plants tended to increase with increasing exchangeable 137Cs in soil, but no overall significant correlation was observed (). There was no clear relationship found between 137Cs concentration in plants and total 137Cs in soil (). On the other hand, they exponentially decreased with higher exchangeable K (). 137Cs concentration in plants had a significant positive linear correlation with the exchangeable 137Cs/K ratio (). In a similar way, 133C concentration in plants showed a positive linear correlation with exchangeable 133Cs/K ().
Figure 2 Relationship of cesium-137 (137Cs) concentration in shoots and brown rice with (a) soil exchangeable 137Cs and (b) soil total 137Cs in the pot experiment. Notes: PI - panicle initiation stage.
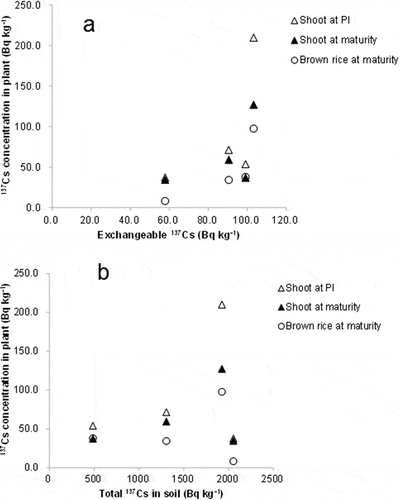
Distribution of Cs in brown rice and shoot
The distribution of dry matter into brown rice, which was calculated as the dry weight of brown rice/(dry weight of brown rice + shoot), showed no significant difference among soils (). In contrast, 137Cs distribution in brown rice, which was calculated as 137Cs accumulated in brown rice/(137Cs accumulated in brown rice + 137Cs accumulated in shoot), significantly varied among soils, ranging from 8.2 to 25.4%. The distribution ratio of 137Cs in brown rice was lowest in the soils from Koriyama and highest in those from Iwaki. 133Cs distributions in brown rice showed variations similar to those in 137Cs. 137Cs and 133Cs distributions in brown rice were considerably lower than those in dry matter in the soils form Koriyama, which indicated that Cs translocation from the shoots to brown rice was limited compared with assimilated dry matter.
Soil solution
The change in K concentrations in soil solution during the growing period is shown in . K concentration was highest during the initial stage and decreased in later growth periods in all soils. The K concentration was lower than 0.1 mmol L−1 in the stages after 55 DAT in all four soils. K concentration tended to be highest in the soils from Koriyama at all stages, followed by those from Iwaki, which was similar to exchangeable K. The average concentration of K in the soil solution during the growing period tended to correlate with exchangeable K among soils (r = 0.906, P < 0.10).
Comparison between pot and field conditions
TFs obtained in the pot experiments and field observations are compared in . The TF for shoots at PI was highest in the soils from Nihonmatsu and Iwaki, followed by Date, and lowest in the soils from Koriyama in the pot experiments. TFs for shoots at maturity showed trends similar to PI. At maturity, TF varied from 0.018 to 0.068 for shoots and 0.004 to 0.065 for brown rice. The TF for brown rice in fields exhibited a similar order among soils, with high values for soils from Nihonmatsu and low values for those from Koriyma. In Nihonmatsu, the TF in the field tended to be higher than the TF obtained in the pot experiment.
DISCUSSION
Relationship between Cs accumulation and exchangeable K and Cs in soil
The results in the pot experiment clearly showed that 137Cs accumulation in rice widely varied among soils (). TF for brown rice varied by 15-fold among soils (). These results confirmed that soil properties are a strong influencing factor in determining 137Cs transfer from soil to rice plants. The relationship between exchangeable K and 137Cs concentration in plants indicated that low exchangeable K enhances 137Cs uptake in rice (). The effect of soil K availability in this study agreed with the results of field surveys in various areas that TF for brown rice tends to increase with low exchangeable K, particularly in the range below 5.3 mmol K kg−1 (Kato Citation2012).
137Cs concentration in plants was most closely related to the exchangeable 137Cs/K ratio (), indicating that these two factors interactively affect 137Cs uptake. A similar relationship found for 133Cs supported the importance of the Cs/K ratio in soils to determine the Cs concentration in plant (). These results probably reflect the competitive uptake of Cs with K in plant root systems and soil–plant systems (White and Broadley Citation2000; Zhu and Smolders Citation2000). An increase in K inhibited Cs absorption in a hydroponic study when K in solution was lower than 1.0 mmol L−1 (Smolders et al. Citation1997). K concentration in the soil solution was below 1.0 mmol L−1 in this study (). Shaw and Bell (Citation1991) suggested that the inhibitory effect of K on Cs uptake in wheat (Triticum aestivum L.) seedlings could be described using the Michaelis–Menten kinetics equation as follows:
where v is the absorption rate of Cs, Vmax is the maximum absorption rate for Cs, [Cs] is the Cs concentration in solution, Km is the Michaelis constant for Cs, [i] is the K concentration in solution and Ki is the inhibition constant.
This relationship implies that when [Cs] is considerably lower than Km and [i] is considerably greater than Ki, [Cs]/[K] becomes the dominant determinant for v. Assuming that Cs and K concentrations in solution are proportional to exchangeable Cs and K in the soil, it is possible that the close relationship between plant Cs concentration and exchangeable Cs/K obtained in this study reflects the competitive inhibitory effect of K in soil solution on Cs absorption. 137Cs uptake was more active before flowering than after flowering (Kodaira et al. Citation1973). In this study, K in soil solution drastically decreased with growing stages (), probably due to the absorption by the root. The changes in K concentrations in soil solution with stages may affect Cs uptake in each growth stages. Precise kinetic studies on root absorption of Cs and K among different stages are needed to fully understand the competition between uptake of Cs and of K.
Factors affecting Cs exchangeability
The close relationship of Cs concentration in rice with exchangeable Cs/K for 137Cs and 133Cs () suggests that, in addition to K availability, exchangeable Cs is also a significant factor in determining Cs uptake. It has also been reported that 137Cs concentration in rice grown in different soils was related to exchangeable Cs levels (Tsumura et al. Citation1984). The absence of a clear relationship between the total amount of 137Cs in soil and the 137Cs concentration in plants () indicated that the total Cs in soil cannot represent the Cs availability of soil, which is in agreement with previous reports (Tsumura et al. Citation1984; Tsukada et al. Citation2002b; Saito et al. Citation2012). The fraction of exchangeable Cs in total Cs varied greatly among soils (). Exchangeable Cs/total Cs was highest in the soils from Iwaki and lowest in those from Koriyama for both 134Cs and 137Cs. The difference in the fraction of exchangeable forms among soils is known to be related to the Cs fixation ability of clay types. Cs fixation is generally strong in specific clay types, such as vermiculite and mica, because of the high frequency of frayed edge sites (FES; Sawhney Citation1964; Tsumura et al. Citation1984; Cremers et al. Citation1988; Nakao et al. Citation2008). The absence of vermiculite in the Iwaki soil could partly explain the relatively high fraction of exchangeable Cs.
Variation among soils in the fraction of exchangeable forms in the total concentration for 133Cs showed a similar tendency with 134Cs and 137Cs, but the values were lower in 133Cs than with 134Cs and 137Cs (). For 137Cs, the fraction of exchangeable form was larger than that for 133Cs by 2.0–3.4-fold. This was probably because most 137Cs was freshly added to the soil after the accident in March 2011, while 133Cs was mostly indigenous and had stabilized. A higher TF for 137Cs than 133Cs was observed in another study (Tsukada et al. Citation2002b). The form of freshly precipitated 137Cs in soil changes and becomes closer to indigenous 133Cs with time (Roig et al. Citation2007). Elucidating the change in availability of freshly added 137Cs to rice over time would be useful for developing sustainable management to control 137Cs uptake and accumulation.
Cs distribution in shoots and grain
137Cs distribution in the grains, which is the most important factor to finally determine 137Cs accumulation in brown rice, averaged 17.4% and was in a range similar to that in previous reports (Tsukada et al. Citation2002a). Variability among soils was also observed, while the distribution of dry matter was relatively constant (). The 137Cs distribution in brown rice was lowest in the soils from Koriyama, which had the highest soil K availability. Similar trends were obtained for 133Cs, indicating that 137Cs and 133Cs movements in plants have a similar mode, which agrees with previous results (Tsukada et al. Citation2002a). The lower distribution of 137Cs and 133Cs in brown rice in the soils from Koriyama than in other soils suggests the possibility that Cs translocation is suppressed when the plant has a high K status. It is worth examining the relationship between K concentrations and the distribution of Cs among plant parts using soils with diverse K availabilities. The Cs that accumulated in brown rice at maturity is derived from two sources: one is direct transport from roots and the other is reallocation from shoots. To accurately understand Cs dynamics in plants, further analysis of Cs movement in plant pathways is required.
Applicability of exchangeable Cs/K as soil index
The similarity in TF between pot and field () confirmed that exchangeable K and Cs at least partly explain the variation in TF under field conditions. A discrepancy between pot and field conditions was also observed, particularly in Nihonmatsu, which implied the possible involvement of other factors. In Nihonmatsu, the TF seemed to be higher in the field than the pot experiment. Although it is currently difficult to precisely identify the reason for the discrepancy, putative factors may include the poor grain ripening in the pot experiment and the lowest K application rate in the fields in Nihonmatsu. 137Cs contamination from irrigation (Fujimura et al. Citation2013), the difference in horizontal distribution of 137Cs and rooting zones between the field and pot, and rice varieties could also be considered.
The results of this study suggest that the combination of exchangeable K and Cs in soil would provide an effective index to estimate Cs levels in brown rice. It is suggested that the suppressive effect of K on Cs uptake is reduce when the soil K availability becomes high (Tsumura et al. Citation1984; Kato Citation2012). The reproducibility of the relationship between the exchangeable Cs/K ratio and Cs accumulation must be further examined using soils with a wider range of properties, particularly soil with more variable K availability. In addition, an understanding of the comparative dynamics of Cs and K in the root absorption and translocation in plant parts would provide a theoretical basis for the use of exchangeable Cs/K as a soil index.
ACKNOWLEDGMENTS
This work was partly funded by “Research and development projects for application in promoting new policy of Agriculture Forestry and Fisheries,” Ministry of Agriculture, Forestry and Fisheries (MAFF), Japan. The authors thank Ms. S. Saka, Ms. M. Ishida and other staff of the NICS for technical assistance in plant and soil analysis.
REFERENCES
- Bartoli F, Burtin G, Herbillon AJ 1991: Disaggregation and clay dispersion of Oxisols: Na resin, a recommended methodology. Geoderma, 49, 301–317. doi:10.1016/0016-7061(91)90082-5
- Cremers A, Elsen A, De Preter P, Maes A 1988: Quantitative analysis of radiocaesium retention in soils. Nature, 335, 247–249. doi:10.1038/335247a0
- Fujimura S, Yoshioka K, Saito T, Sato M, Sato M, Sakuma Y, Muramatsu Y 2013: Effects of applying potassium, zeolite and vermiculite on the radiocesium uptake by rice plants grown in paddy field soils collected from Fukushima prefecture. Plant Prod. Sci., 16, 166–170.
- Higashi T, Ikeda H 1974: Dissolution of allophane by acid oxalate solution. Clay Sci., 4, 205–211.
- Hossener LR 1996: Dissolution for total element analysis. In Methods of Soil Analysis Part 3. Chemical Methods, Ed.. J. M. Bigham, 49–64. Soil Science Society of America, Wisconsin, USA.
- Kato N 2012: Countermeasures to reduce radiocaesium contamination in paddy rice, soybean and cabbage. In Abstract, International science symposium on combating radionuclide contamination in Agro-soil environment. 3–10 March 2012 Koriyama, Fukushima, pp 317–318.
- Kodaira K, Tsumura A, Kobayashi H 1973: Uptake of radioactive strontium and cesium in rice plants (1) accumulation of Sr and Cs in Rice Grains through Roots. J. Radia. Res., 14, 31–39. doi:10.1269/jrr.14.31
- Mehra OP, Jackson ML 1960: Iron oxide removal from soils and clays by a dithionite-citrate system buffered with sodium bicarbonate. Clays Clay Miner., 7, 317–327. doi:10.1346/CCMN.1958.0070122
- Mikutta R, Kleber M, Kaiser K, Jahn R 2005: Organic matter removal from soils using hydrogen peroxide, sodium hypochlorite, and disodium peroxodisulfate. Soil Sci. Soc. Am. J., 69, 120–135. doi:10.2136/sssaj2005.0120
- Mimura T, Fujiwara T, Fukuda H 2014: As plant scientists who faced the Fukushima nuclear disaster. J. Plant Res., 127, 3–4. doi:10.1007/s10265-013-0606-y
- Miyashita K 2012: Radionuclide contamination of farming land soils and agricultural products caused by the accident of Fukushima Daiichi nuclear power station. In Abstract, International science symposium on combating radionuclide contamination in Agro-soil environment. 3–10 March 2012 Koriyama, Fukushima, pp 237.
- Nakao A, Thiry Y, Funakawa S, Kosaki T 2008: Characterization of the frayed edge site of micaceous minerals in soil clays influenced by different pedogenetic conditions in Japan and northern Thailand. Soil Sci. Plant Nutri., 54, 479–489. doi:10.1111/j.1747-0765.2008.00262.x
- Roig M, Vidal M, Rauret G, Rigol A 2007: Prediction of radionuclide aging in soils from the chernobyl and Mediterranean areas. J. Env. Qual, 36, 943–952. doi:10.2134/jeq2006.0402
- Saito T, Ohkoshi S, Fujimura S, Iwabuchi K, Saito M, Nemoto T, Sato M, Yoshioka K, Tsukada H 2012: Effect of potassium application on root uptake of radiocesium in rice. In Proc. International symposium on environmental monitoring and dose estimation of residents after accident of TEPCO’s Fukushima Daiichi Nuclear Power Stations [CD-ROM]. Kyoto University Research Reactor Institute, Osaka.
- Sawhney BL 1964: Sorption and fixation of microquantities of cesium by clay minerals: effect of saturating cations. Proc. Soil Sci. Soc. Am, 28, 183–186. doi:10.2136/sssaj1964.03615995002800020017x
- Shaw G, Bell JNB 1991: Competitive effects of potassium and ammonium on caesium uptake kinetics in wheat. J. Env. Radi, 13, 283–296. doi:10.1016/0265-931X(91)90002-W
- Smolders E, Vandenbrande K, Merckx R 1997: Concentrations of 137Cs and K in soil solution predict the plant availability of 137Cs in soils. Env. Sci. Tech, 31, 3432–3438. doi:10.1021/es970113r
- Toriyama K, Ishida H 1987: Method of estimating time of NH4-N disappearance in paddy field by soil solution analysis. Jap. J. Soil Sci. Plant Nutri, 58, 747–749. In Japanese.
- Tsukada H, Hasegawa H, Hisamatsu S, Yamasaki S 2002a: Rice uptake and distributions of radioactive 137Cs, stable 133Cs and K from soil. Env. Pollu., 117, 403–409. doi:10.1016/S0269-7491(01)00199-3
- Tsukada H, Hasegawa H, Hisamatsu S, Yamasaki S 2002b: Transfer of 137Cs and stable Cs from paddy soil to polished rice in Aomori, Japan. J. Env. Radio., 59, 351–363. doi:10.1016/S0265-931X(01)00083-2
- Tsumura A, Komamura M, Kobayashi H 1984: Behavior of radioactive Sr and Cs in soils and soil-plant systems. Bull. Natl. Inst. Agric. Sci. Ser., B, 36, 57–113. In Japanese.
- White PJ, Broadley MR 2000: Tansley Review No. 113. New Phytol., 147, 241–256. doi:10.1046/j.1469-8137.2000.00704.x
- Yamaguchi N, Takata Y, Hayashi K et al. 2012: Behavior of radiocaesium in soil-plant systems and its controlling factor. A review. Bull. Nat. Inst. Agro-Environ. Sci., 31, 75–129, In Japanese
- Zhu YG, Smolders E 2000: Plant uptake of radiocaesium: a review of mechanisms, regulation and application. J. Exp. Bot., 51, 1635–1645. doi:10.1093/jexbot/51.351.1635