Abstract
Following the accident at the Tokyo Electric Power Company, Fukushima Daiichi Nuclear Power Plant (FDNPP), radiocesium (134Cs + 137Cs) concentrations in deciduous mature fruits were determined in orchards in the northern area of Fukushima Prefecture. At the time of the nuclear accident, most deciduous fruit trees were in the dormant stage prior to bud burst. To evaluate the relationship between radiocesium deposition in the soil and fruit contamination, radiocesium concentrations were measured from the 5-cm topsoil and from six fruit species across 17 orchards in 2011. The vertical distribution of radiocesium in the topsoil (0–30 cm in depth) and its spatial distribution in the 5-cm topsoil underlying the tree canopy of a peach, Prunus persica (L.) Batsh, orchard (“Akatsuki” cultivar) were also investigated. Significant correlations between the radiocesium concentration in the mature fruit and that in the 5-cm topsoil layer were observed for the 17 orchards as well as for the trees of the peach orchard. However, 93% of the 137Cs found in the 30-cm soil core was retained within the top 3 cm of the soil in the peach orchard. Considering the profile of the root of this deciduous fruit tree, we assumed a negligible level of radiocesium uptake via the roots. However, the possibility of inward migration via the bark was undeniable, because some radiocesium adhered to the tree canopy before bud burst while depositing on the soil surface. Additionally, transfer factors for peach and grape, hybrid of Vitis labrusca L. and Vitis vinifera L., from young, uncontaminated trees cultivated with contaminated soil were lower than those previously reported.
1 INTRODUCTION
Radioactive fallout released from the Tokyo Electric Power Company, Fukushima Daiichi Nuclear Power Plant (FDNPP) accident contaminated orchards throughout the major fruit production area in Fukushima Prefecture. Deposition of radionuclides in this area was caused primarily by rainfall on March 15, 2011, which took place during the dormant phenological stage of deciduous fruit trees. Unfortunately, there are not many reports concerning deciduous fruit trees from previous nuclear accidents. Anguissola Scotti and Silva (Citation1992) measured cesium-137 (137Cs) on the leaves and fruits of Prunus avium (L.) L. (sweet cherry), Prunus persica (L.) Batsch (peach), Pyrus pyrifolia (Burm.f.) Nakai (Japanese pear) and Malus pumila Mill. (apple) during June and September in the Chernobyl accident year of 1986. The content of 137Cs in leaves showed a dependence on the interception ability of the foliage. This report indicates that 137Cs immigration into trees occurred by leaf absorption in the Chernobyl accident. Antonopoulos-Domis et al. (Citation1991) showed that contamination by root uptake is a small fraction of the total contamination of each year’s new tree product, investigating the radiocesium contamination of different parts (fruits, leaves and shoots) of selected apricot, Prunus domestica L., trees in North Greece during 1987 and 1988, regarding Chernobyl fallout. But they did not mention the radiocesium migration into the deciduous fruit trees in the accident year of 1986. In addition, they proposed a compartment model for the long-term contamination of perennial plant products with time-dependent decay by the sum of two exponentials based on experimental observations of the radiocesium contamination of fruits or leaves of various fruits (sweet cherry, peach and apple) on two agricultural experimentation farms in North Greece from 1987 to 1995 (Antonopoulos-Domis et al. Citation1996). In contrast, Al-Oudat et al. (Citation2006) showed that the transfer of 137Cs from contaminated soil by cesium chloride (CsCl) solution mixed 137Cs solution to fruits of olive, Olea europaea L., apricot and grape, hybrid of Vitis labrusca L. and Vitis vinifera L., occurred with no consistent differences for four successive years under irrigated field conditions in arid regions (Syria), indicating no apparent aging effects.
However, there is no information on radiocesium migration pathways into deciduous fruit trees from radioactive fallout during the dormant period. In general, the absorptive rhizosphere of fruit trees (except for some species such as blueberry,Vaccinium corymbosum L., grape, and citrus, Citrus unshiu Marcow. et al.,) is deeper than 10 cm in orchard soils (Antonopoulos-Domis et al. Citation1990). In addition, there were roots of undergrowth running through the surface soil of the ground with high density because sod culture was applied in almost all orchards of these regions. Therefore, radiocesium contamination by root uptake was likely negligible during the accident year, as was reported by Antonopoulos-Domis et al. (Citation1990) in relation to the Chernobyl accident. Carini and Bengtsson (Citation2001), in their review of an interesting experiment conducted by Katana and colleagues, showed that 134Cs migrated into mature apple fruits from the bark of a 2-year-old stem kept in contact with the cesium-134 (134Cs) solution after 10 weeks.
Investigation of radiocesium deposition in orchards and development of decontamination methods were started on April 11, 2011, by the Fruit Tree Research Center of Fukushima Agricultural Technology Center (FTRC). Monitoring surveys for radiocesium contamination of various fruits and soil were carried out in the major fruit production areas in northern Fukushima Prefecture, including Fukushima City, Date City and Kohri Town.
In the present study, the relationship between the radiocesium concentration in mature fruit and soil within the first year following the accident was investigated. In addition, in order to examine how fruit trees take up radiocesium from soil, the transfer factors for peaches and grapes were studied by transplanting uncontaminated young trees or vines into contaminated soils and analyzing radiocesium in mature fruits. In this paper, radiocesium means the sum of 134Cs and 137Cs.
2 MATERIALS AND METHODS
2.1 The relationship between the radiocesium concentration of mature fruit and soil in the accident year
The following 17 orchards were selected for investigation: eight orchards with six different fruits (sweet cherry, peach, grape, Japanese pear, apple and Japanese persimmon, Diospyros kaki Thunb.) in FTRC, three orchards (peach, grape and Japanese persimmon) in Date City, five orchards (sweet cherry, peach, Japanese pear and apple) in Fukushima City, and one peach orchard in Kohri Town. These investigation orchards were approximately 60 to 80 km away from FDNPP. The observation area in each orchard was approximately 300 to 600 m2.
Topsoils of 0 to 5 cm depth were collected in each orchard. Five topsoil samples were collected per orchard using a cylindrical soil sampler made of polyvinyl chloride (70 mm inside diameter), and were combined into one sample. The cylindrical soil sampler was the original equipment that was self-made by the Research Center for Electron Photon Science (RCEPS) of Tohoku University. Soils were sampled on April 26, 2011, in FTRC’s orchards and on April 27 in the other orchards. Fruits were collected at the mature stage from three to five trees in each orchard. The fruit samples were combined into one sample for each orchard and washed. Sweet cherry and grape were measured using the flesh part with peel, and the others were measured using the flesh part without peel. The stone and seeds were removed from mature fruits, and then the fruits were pulped. Radiocesium concentrations in soil and fruit samples were measured as described in section 2.4.
To estimate the contribution of root uptake, the F-to-S ratio was calculated. F is the 137Cs concentration in mature fruit. S is a quarter of the 137Cs concentration in the 5-cm topsoil layer, representing the 137Cs concentration in 20-cm topsoil layer. The International Atomic Energy Agency (IAEA) report (Citation2010a) adopted the standardization of the International Union of Radioecologists (IUR) of rooting depth, which is 20 cm for all plants excluding grasses of 10 cm, instead of the real rooting depth in defining soil-to-plant transfer factors. Since most of the 137Cs in the top soil layer of 20 cm is present in the surface layer within 5 cm, a standardized soil layer was assessed at a quarter of the 137Cs concentration in the 5-cm topsoil layer.
To confirm the dilution effect to the mature fruit’s radioactive Cs level, a regression analysis between fruit weight and radiocesium concentration was conducted. Data on “Benisayaka,” “Satonishiki,” “Benisyuho” (sweet cherry), “Hatsuhime,” “Akatsuki,” “Kawanakajimahakuto,” “Yuzora” (peach), “Kosui,” “Ryoho” (Japanese pear), “Fuji” (apple) and “Hachiya” (Japanese persimmon) collected in FTRC in the year 2011 were used for analysis. The weight of 10 to 30 mature fruits was measured. The averaged fruit weight data and radiocesium concentration were converted in dry base by the dry matter content after freeze-drying for 72 h or more.
2.2 Vertical and spatial distribution of the radiocesium concentration in orchard soils
To examine the vertical distribution of radiocesium, topsoil (0 to 30 cm in depth) in the “Akatsuki” peach orchard at FTRC, where 12 “Akatsuki” trees were planted at 7 m × 7 m intervals, was collected with a cylindrical soil sampler at one location on April 26, 2011, and sub-divided into 3-cm layers. To estimate the spatial distribution of radiocesium contamination by the tree canopy in a peach orchard covering an area of 600 m2, four 5-cm topsoil samples were collected per tree canopy, on June 6, 2011. Four soil sampling locations were selected at a distance of 2 m from trunk. Soil samples were sieved using stainless steel sieves (5.66 mm of opening). The soils were put into counting vessels (U-8 vessel; 5 cm diameter and 5 cm height) after they were homogenized by shaking the plastic bags containing soil. Ten mature fruit samples per tree were collected on August 10, 2011. Radiocesium concentrations in soil and fruit samples were measured as described in section 2.4.
The vertical distribution of radiocesium within the top 0–30 cm soil layer was expressed as a deposition rate, D, according to depth, as calculated by the following equation, assuming a bulk density of 1 for each depth interval:
2.3 Transfer of radiocesium into leaves and fruits from soil
To examine the transfer of radiocesium from the contaminated soil into fruit, three 5-year-old peach trees (cultivar “Akatsuki”), grown in containers with uncontaminated soil, were obtained from the greenhouse at the Institute for Sustainable Agro-ecosystem Services, Graduate School of Agricultural and Life Science, University of Tokyo (ISAS) at Nishi-Tokyo City, about 230 km from FDNPP. These plants, which were free from contamination of radiocesium fallout from the accident, had their roots cleaned with a high pressure washer and were transplanted into the same sites where peach trees had been removed at FTRC on April 14, 2012. Three samples of fruits and leaves were collected from “Akatsuki” trees on August 12, 2012, when the fruit had ripened. The 30-cm topsoil layer was collected with a cylindrical soil sampler at a distance of 35 to 45 cm from the trunk of each tree, on November 11, 2012. The soil layer was sub-sampled at depth intervals of 3, 9, 15 and 21 cm.
Furthermore, in order to examine the transfer factor of mature fruits for grapes and peaches in detail, three 3-year-old grapevines, (cultivar “Pione”) and a 3-year-old peach tree (cultivar “Hakuho”), grown in pots, were obtained from a closed-system greenhouse at ISAS. On April 14, 2012, their roots were cleaned with a high-pressure washer and they were transplanted into black, 60-L plastic pots, which were filled with contaminated soil collected from the top 5-cm soil layer, including undergrowth, from the peach orchard in Date City, on March 22, 2012. Contaminated soil was spread on a sheet and mixed with undergrowth divided in pieces by hand. Three samples of mature fruits were collected from three “Hakuho” trees on August 10, 2012. Fruits and leaves of “Pione” were collected from three vines on September 13 and September 20, 2012. The samples of fruits and leaves collected from “Hakuho” and “Pione” were combined into one fruit sample and one leaf sample for each cultivar to measure the content of radiocesium. One plant was cut into pieces on November 11, 2012. Plant sub-samples included shoots, branches, fine roots (1.5 mm and less) and other roots (> 1.5 mm diameter, where nutrients are stored). Each sub-sample was washed and analyzed for radiocesium content.
The transfer factor (TF) of the fruit or leaf was calculated using the following equation:
The radiocesium concentration of the 20-cm topsoil layer of the “Akatsuki” orchard was used to calculate the TF in the replanting examination of 5-year-old “Akatsuki.” The soil at the site where the peach trees were transplanted was well mixed, so the radiocesium was distributed almost evenly down to a depth of 21 cm. The radiocesium concentrations of the top 5-cm soil layer scraped from the peach orchard in Date City were used to calculate TFs in the pot-planting experiment of “Pione” and “Hakuho.” The soil type in both FTRC and Date City was clay loam – brown forest soil.
2.4 Sample treatments and radiocesium measurements
The radiocesium concentration in soil was converted to a DW basis using a dry-to-wet ratio after drying 1 d at 105°C. The radiocesium concentration in fruit was converted to a wet weight basis using a dry-to-wet ratio after freeze-drying.
The 134Cs and 137Cs concentrations in the samples were analyzed with germanium (Ge) semiconductor detector systems at the Faculty of Science, Gakushuin University, and at the RCEPS of Tohoku University. The counting time for each sample was about 1 to 24 h, depending on the activity level. Measurements were carried out within 14 d after sampling. Decay correction was made to the sampling date.
For the samples described in section 2.3, radiocesium concentrations in plant samples were determined on the Ge detector system at the Foundation for Promotion of Material Science and Technology of Japan, and those in soil samples were determined by an sodium iodide (NaI) scintillation spectrometer (CAN-OSP-NAI, Hitachi Aloka Medical) at FTRC. Radiocesium concentrations were decay-corrected to the sampling date. The radiocesium concentration of the 20-cm topsoil layer in the location of the planted 5-year-old “Akatsuki” was calculated using a deposition rate according to depth that was obtained from Eq. 1.
3 RESULTS
3.1 Relationship between the radiocesium concentrations in mature fruit and soil in the first year following the accident
There was a significant positive correlation between the radiocesium concentration in the mature fruit and that in the 5-cm topsoil layer (y = 4.38x + 4.71 R2 = 0.323, P < 0.01). The F-to-S ratio of each deciduous fruit tree species was almost 10–2 orders (). The F-to-S ratio of sweet cherry, the harvest period of which is earlier than that of others, was significantly higher than that of other fruit species by Tukey’s test at P < 0.01. There was a difference of 11.5 times in the F-to-S ratio from the maximum value of 5.4 × 10–2 for the sweet cherry “Benishuho” in FTRC, to the minimum of 4.7 × 10–3 in the Japanese pear “Kosui” in FTRC. The relationships between the radiocesium concentration in mature fruits and soils were different among the fruit species. For example, radiocesium concentrations in sweet cherries were relatively higher than those in grapes or pears, regardless of the soil radioactive Cs level.
Table 1 Comparison of the radiocesium concentration between the 5-cm topsoil layer and mature fruit of Prunus avium (L.) L. (sweet cherry), Prunus persica (L.) Batsch (peach), hybrid of Vitis labrusca L. and Vitis vinifera L. (grape), Pyrus pyrifolia (Burm.f.) Nakai (Japanese pear), Malus pumila Mill. (apple) and Diospyros kaki Thunb. (Japanese persimmon) in the year of 2011
A significant negative correlation was recognized between the mature fruit weight and the concentration of the radiocesium (134Cs + 137Cs) in the regression analysis conducted using data of sweet cherry, peach, Japanese pear, apple and Japanese persimmon collected in FTRC in the year 2011 ().
Figure 1 Relationship between the mature fruit weight and the concentration of radiocesium (134Cs + 137Cs) in the year of 2011. Note: Regression analysis was conducted using the data of “Benisayaka,” “Satonishiki,” “Benisyuho” (sweet cherry, Prunus avium (L.) L.), “Hatsuhime,” “Akatsuki,” “Kawanakajimahakuto,” “Yuzora” (peach, Prunus persica (L.) Batsch), “Kosui,” “Ryoho” (Japanese pear, Pyrus pyrifolia (Burm.f.) Nakai) “Fuji” (apple, Malus pumila Mill.) and “Hachiya” (Japanese persimmon, Diospyros kaki Thunb.) collected in FTRC in the year of 2011. DW, dry weight.
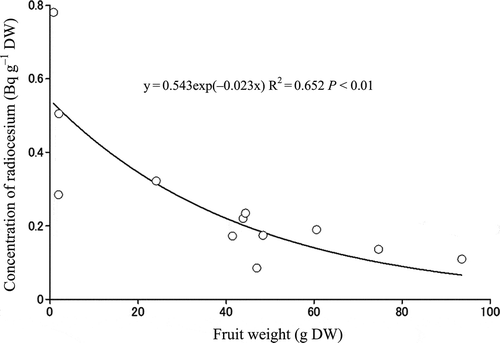
3.2 Vertical and spatial distribution of radiocesium in peach orchard soil
The vertical distribution of radiocesium in the peach orchard soil from FTRC is shown in . Ninety-three percent of the 137Cs found in the 30-cm soil core was retained in the top 3 cm. The concentration thus decreased with depth ().
Figure 2 Distribution of radiocesium (137Cs) along the soil profile in peach, Prunus persica (L.) Batsch, “Akatsuki” orchard. Note: The 30-cm topsoil layer was collected with the cylindrical soil sampler at one undisturbed place in the orchard on 26 April 2011. The soil layer was divided evenly by 3 cm. Decay correction was made to the sampling date. DW, dry weight.
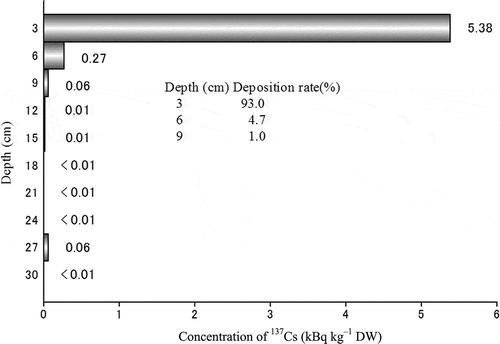
shows the spatial distribution of radiocesium in the top 5 cm of soil and in fruits for 12 trees in the orchard. There was a large variability in the soil radiocesium concentrations, from 2.5 to 11.9 kBq kg−1 (DW). The coefficient of variation was 42.2%. In contrast, the fruit radiocesium concentration ranged from 23.1 to 34.9 Bq kg−1 (FW) with a coefficient of variation of 12.8 % (). A significant correlation (y = 0.766x + 21.8 R2 = 0.664, P < 0.01) was found in the radiocesium concentration between fruits and soils in eight trees, excluding the peripheral trees facing the road and sites growing only grass to avoid the peripheral effect.
Figure 3 Comparison between the radiocesium contamination in soil and fruit collected from the peach, Prunus persica (L.) Batsch, orchard of “Akatuki.” Note: The 5-cm topsoil layer was collected with the cylindrical soil sampler at three places under the canopy of 12 “Akatuki” trees planted in rows of 12 at 7 m × 7 m spacing, on June 6, 2011. We collected 10 fruits per tree on August 10, 2011. (a) Map of radiocesium (134Cs + 137Cs) concentration of 5-cm topsoil layer; (b) radiocesium (134Cs + 137Cs) concentration of mature fruit. Every measured activity in samples was corrected back to the sampling date by decay compensation; FW: fresh weight; DW: dry weight.
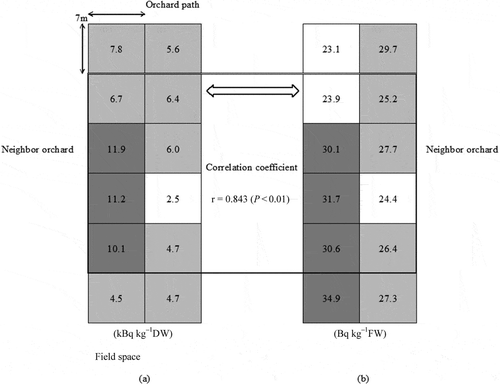
3.3 Transfer of radiocesium into leaves and fruits from soil
Transfer of radiocesium occurred between soil and the leaves or fruits of peach trees and grape vines which were transplanted from an uncontaminated area to contaminated soil at FTRC or to pots with contaminated soil ().
Table 2 Transfer factor of Prunus persica (L.) Batsh (peach) and hybrid of Vitis labrusca L. and Vitis vinifera L. (grape) planted in disturbed soil or cultivated in pots put in the contaminated soil (2012)
Radiocesium concentrations in each sub-sample of the “Pione” vine are shown in . A higher concentration was detected in fine roots than in leaves. The concentration found in branches was the same as that in the “other” roots, which is approximately one third of that in fine roots (). Fine roots have direct contact with contaminated soil and the root tissue is not diluted significantly.
Figure 4 Concentration of radiocesium (134Cs + 137Cs) in different parts of the grape “Pione”, hybrid of Vitis labrusca L. and Vitis vinifera L. tree. Note: fruits and leaves were collected on September 11 and 20, 2012; other parts were collected on November 11, 2012. Samples were combined into one fruit and one leaf sample. One plant was cut into pieces on November 11, 2012. Plant sub-samples included shoots, branches, fine roots (1.5 mm and less in diameter) and other roots (> 1.5 mm in diameter, where nutrients are stored). DW, dry weight.
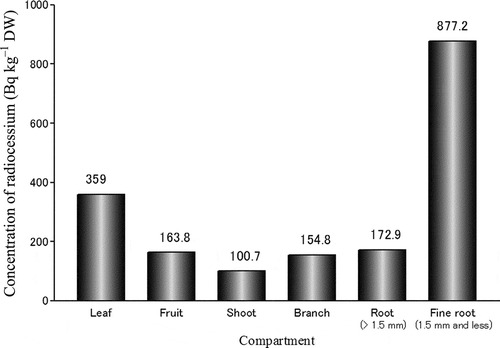
TFs of mature fruits are on the order of 10–4 for peaches and one order of magnitude higher for grapes (10–3). Foliar TFs are on the order of 10–3 for both peach and grape ().
4 DISCUSSION
When the Chernobyl accident occurred on April 26, 1986, deciduous fruit trees were developing leaves. Radiocesium was found to be absorbed into trees mainly via leaves. Radiocesium is translocated from leaves to other organs immediately after foliar absorption. Carini and Lombi (Citation1997) reported that root uptake of 134Cs was 20 times lower than foliar uptake. The 137Cs contents of the deciduous fruits in Italy in the year of the Chernobyl accident were similar to the levels found in our investigation, in spite of the 102 orders lower content of 5-cm topsoil in Italy than that in the location of our study (Anguissola Scotti and Silva Citation1992). However, the FDNPP accident and subsequent radioactive fallout took place before the bud burst of deciduous fruit trees. Consequently, the major concern regarding radionuclide dynamics focused on root absorption from soil. According to an IAEA report (IAEA Citation2003a), the TFs for deciduous fruit trees (except for the chestnut, Castanea crenata Sieold & Zucc.) from soil to mature fruits were in the range of 8.6 × 10–4 to 8.0 × 10–2, and grape was found to have the highest TF.
Antonopoulos-Domis et al. (Citation1996) related that it was impossible to measure the radiocesium contamination of fruits except sweet cherries in 1991 to 1995, indicating higher radiocesium contamination in fruits of sweet cherry trees than other fruit trees. Cherry trees have several scaffold limbs with many lateral branches even after pruning, and the fruit size is smaller than other fruit species. The higher levels observed in sweet cherries may be because of the smaller fruit size, with less material for dilution, or because of the prevalence of lenticels on sweet cherry trees, where radiocesium can accumulate and be absorbed (Takata Citation2013). Since a significant negative correlation was recognized between the weights and the concentrations of the radiocesium in mature fruits by the regression analysis in the present study, a size effect was identified (). Therefore, one of the reasons for the high level radiocesium contamination of sweet cherries () was supposed to be the dilution effect of assimilates transported into fruit.
Although, considering the profile of the root of the deciduous fruit tree, a negligible level of radiocesium by root uptake was supposed, significant correlations in the radiocesium concentration between the mature fruit and the top 5-cm soil layer were recognized in both the investigation of 17 orchards containing six deciduous fruit tree species and the eight peach trees in FTRC in the present study, conducted in 2011. However, the ratio of F to S was in the range of 4.7 × 10–3 to 5.4 × 10–2. The difference among trees was within a factor of 10, and fruits have even less variation, though sweet cherry showed significantly higher values than the others. These facts were different from the IAEA report (IAEA Citation2003b) that showed the difference of a factor of 102 within the same fruit tree species, such as apple. Furthermore, the ratios of F to S in our investigation were one to two orders of magnitude higher than the TFs shown by the IAEA report.
It has been shown that birch canopies intercept 20 to 25% of resuspended radioactive particles during the winter period (IAEA Citation2010b). It was also shown that fruit trees’ canopy, like that of forest trees, intercepted radioactive nuclides (Sato Citation2012). The contamination of fruit is dependent on the growth dilution and the ratio between the leaf area and the corresponding mass of the fruit. The higher the ratio, the higher the radionuclide uptake (Carini Citation2009).
The maldistribution of radiocesium in near-surface soil () agrees with other studies in orchards and undisturbed fields (Ohno et al. Citation2012; Shiozawa Citation2013; Sato Citation2014a). The correlation between radiocesium concentration in the mature fruit and in the topsoil layer is, therefore, likely due to the fact that radiocesium is strongly retained in soil. On the other hand, the observed concentrations were also considered to reflect the levels that are intercepted by the canopy of dormant trees. It was found that radiocesium adhered to the tree canopy before bud burst at the same time as the deposition on the soil surface (Sato Citation2012). It was estimated that high correlation between the contamination level on the bark and the radiocesium concentration in the topsoil layer may be latent. Consequently, the possibility of migration inward via bark was undeniable, whereas it was suspected that the relationship of the radiocesium concentration between the mature fruit and the topsoil layer might be a spurious correlation.
The absorptive rhizosphere of Japanese persimmon and peach trees more than 6 years old is deeper than 20 cm (unpublished data). In previous work regarding Chernobyl fallout in Northern Greece (Antonopoulos-Domis et al. Citation1990, Citation1991), 95% of the radiocesium was found within the top 10 cm of undisturbed soil and, since trees have no roots in this layer, contamination by rοοt uptake was a small or negligible fraction of the total contamination of tree products from every year studied. The principal source of the radiocesium inventory was rather the plant itself for the first few years following deposition. Takata (Citation2013) compared the radiocesium contamination of 5-year-old “Akatsuki” peach trees planted in containers that were covered with sheets and not covered when fallout occurred. They concluded that, in the accident year, root uptake of radiocesium deposited on the soil contributed very little to the overall contamination compared with adsorption through aboveground plant parts such as leaves and bark. These studies further substantiate that it is unlikely that radiocesium detected in sweet cherries, peaches, Japanese pears, grapes, apples and Japanese persimmons was due to root uptake of radiocesium from soil. Therefore, the significant correlation we found between soil and fruit radiocesium concentration (discussed in sections 3.1 and 3.2) may not be indicative of the TF in fruit due to root uptake. In fact, the fruit radiocesium concentrations we found (discussed in sections 3.1 and 3.2) are much higher than the estimated values based upon previously reported TFs (IAEA Citation2003b).
Finally, we concluded, from the results of our investigations, that root uptake of radiocesium was negligible in the deciduous fruit trees before bud burst. Therefore, in order to clarify the influence of radiocesium in soil, an examination of the transfer of radiocesium from the contaminated soil into fruit was conducted.
The TFs for peaches and grapes, calculated in the present experiment, were on the order of 10–4 and 10–3 (). In these pot examinations, undergrowth containing radiocesium was mixed in contaminated soil to increase the content of absorptive radiocesium. However, the TFs of peaches were more than one order of magnitude lower than the minimum value, 1.8 × 10–3, in the IAEA report (IAEA Citation2003b). And the TF of grape was similar to the minimum value, 1 × 10–3, in the previous report (IAEA Citation2003b). Therefore, as stated previously, the radiocesium contamination of fruits found in 2011 ( and ) is likely not due to root uptake from soil, but rather mainly due to migration within the aboveground parts of fruit trees. Following the accident, the exposed portions of the trees and vines showed high levels of radiocesium (Sato Citation2012). Momoshima and Bondietti (Citation1994) examined the radial distributions of 137Cs in wood xylem for eight species in a forest in Tennessee, USA, to evaluate the long-term behavior in the wood stem of radionuclides from atmospheric nuclear tests and nuclear accidents. The 137Cs concentration did not show any correlation with the temporal record of cumulative deposition of fallout. Momoshima and Bondietti (Citation1994) related that 137Cs moved in ray tissue, crossing through phloem and wood from periderm to heartwood. It was suggested that radiocesium migrates inward by way of these organs from the surface of the bark, indicating that the migration source of the deciduous fruit tree before bud burst was deposits on the wet bark from rainfall containing radiocesium after the nuclear accident. Sato (Citation2014b) confirmed radiocesium migration into the leaves of 2-year-old peach trees from trunks pasted with radiocesium extract before bud burst. Translocation of radiocesium therefore likely occurred via the bark (ray tissue–xylem tissue)–leaf (phloem tissue)–fruit pathway. This finding agrees with results obtained by Takata (Citation2013).
ACKNOWLEDGMENTS
The authors thank Dr. Jean Moran (Department of Earth & Environmental Science, California State University) for her useful comments and for improving the English in the manuscript. Part of this work was supported by the research project “Development of Radioactive Materials Removal and Reduction Technology for Forests and Farmland” (2012) conducted by the Ministry of Agriculture, Forestry and Fisheries of Japan.
REFERENCES
- Al-Oudat M, Asfary AF, Mukhalallti H, Al-Hamwi A, Kanakri S 2006: Transfer factors of 137Cs and 90Sr from soil to trees in arid regions. J. Environ. Radioact. 90, 78–88. doi:10.1016/j.jenvrad.2006.06.005
- Anguissola Scotti I, Silva S 1992: Foliar absorption and leaf-fruit transfer of 137Cs in fruit trees. J. Environ. Radioact. 16, 97–108. doi:10.1016/0265-931X(92)90010-Q
- Antonopoulos-Domis M, Clouvas A, Gagianas A 1990: Compartment model for long-term contamination prediction in deciduous fruit trees after a nuclear accident. Health Phys. 58, 737–741. doi:10.1097/00004032-199006000-00005
- Antonopoulos-Domis M, Clouvas A, A 1991: Radiocesium dynamics in fruit trees following the chernobyl accident. Health Phys. 61, 837–842. doi:10.1097/00004032-199112000-00015
- Antonopoulos-Domis M, Clouvas A, Gagianas A 1996: Long term radiocesium contamination of fruit trees following the Chernobyl accident. Health Phys. 71, 910–914. doi:10.1097/00004032-199612000-00007
- Carini F 2009: Radionuclide transfer to fruit in the IAEA TRS 364 revision. J. Environ. Radioact. 100, 752–756. doi:10.1016/j.jenvrad.2008.10.001
- Carini F, Bengtsson G 2001: Post-deposition transport of radionuclides in fruit. J. Environ. Radioact. 52, 215–236. doi:10.1016/S0265-931X(00)00034-5
- Carini F, Lombi E 1997: Foliar and soil uptake of 134Cs and 85Sr by grape vines. Sci.Total Environ., 207, 157–164. doi:10.1016/S0048-9697(97)00261-1
- IAEA 2003a: Report of the Fruits Working Group of BIOMASS Theme 3, Modelling the transfer of radionuclides to fruit, pp.17.
- IAEA 2003b: Report of the Fruits Working Group of BIOMASS Theme 3, Modelling the transfer of radionuclides to fruit, pp.122–135.
- IAEA 2010a: Technical reports series No.472, Handbook of parameter values for the prediction of radionuclide transfer in terrestrial and freshwater environments, pp.39–82.
- IAEA 2010b: Technical reports series No.472, Handbook of parameter values for the prediction of radionuclide transfer in terrestrial and freshwater environments, pp.99–105.
- Momoshima N, Bondietti EA 1994: The radial distribution of 90Sr and 137Cs in trees. J. Environ. Radioact. 22, 93–109. doi:10.1016/0265-931X(94)90016-7
- Ohno T, Muramatsu Y, Miura Y, Oda K, Inagawa N, Ogawa H, Yamazaki A, Toyama C, Sato M 2012: Depth profiles of radioactive cesium and iodine released from the Fukushima Daiichi nuclear power plant in different agricultural fields and forests. Geochemical J. 46, 287–295. doi:10.2343/geochemj.2.0204
- Sato M 2012: Fruit in Fukushima in the nuclear accident year-Report of the first year examination to take measures against the radioactive contamination of nuclear power plant accident. Radiochemistry News. 26, 21–31. in Japanese.
- Sato M 2014a: Investigation of the radiocesium migration pathway into the deciduous fruit tree contaminated at the dormant period and trials to decrease the radiocesium uptake via bark. Jpn. J. Soi Sci. Plant. Nutr. 85, 103–106. in Japanese.
- Sato M 2014b: Investigation of the radiocesium migration pathway into the deciduous fruit tree contaminated at the dormant period. Bull. Fukushima Agr. Technol. Cent. Radioactive Substances Special Issue, 70–73. in Japanese.
- Shiozawa S 2013: Vertical migration of radiocesium fallout in soil in Fukushima. Eds. T. M. Nakanishi, K. Tanoi. Agricultural implications of the Fukushima nuclear accident. pp. 49–60. Springer, UK.
- Takata D 2013: Distribution of radiocesium from the radioactive fallout in fruit trees. Eds. T. M. Nakanishi, K. Tanoi. Agricultural implications of the Fukushimanuclear accident. pp. 143-162. Springer, UK.