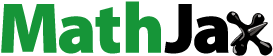
Abstract
An isotopes (15N) tracing study was conducted under laboratory conditions to evaluate the effects of temperature changes (5–35°C) on gross nitrogen (N) transformations in two contrasting soils of subtropical broad-leaved forest (BF) and coniferous forest (CF). With increasing temperature in BF soils, gross N immobilization rates increased to a lesser extent than gross N mineralization rates, which resulted in an increase in net N mineralization. By contrast, in the CF soils, the equally proportional increase in gross N mineralization and immobilization that occurred at 5–25°C led to a lack of change in net N mineralization in response to temperature, whereas increasing the temperature from 25 to 35°C resulted in a greater increase in the gross N mineralization rate than in the gross N immobilization rate, thereby increasing net N mineralization. The temperature sensitivity of gross nitrification was observed in the BF soils but not in the CF soils. The same temperature sensitivity of gross N mineralization and immobilization rates in the CF soils at temperatures below 25°C, combined with negligible nitrification rates, suggests that the N available for plants and microbial growth, and the loss by nitrate (NO3 −) leaching, is probably low in the CFs.
INTRODUCTION
Temperature changes arising from seasonal variation or global warming might influence net nitrogen (N) mineralization and N availability in soils (Dalias et al. Citation2002; Wang et al. Citation2006). Particularly for subtropical forest systems without the application of fertilizers, atmospheric N deposition, N2 fixation, and litter and soil organic matter decomposition are the primary sources of N available to plants. Thus, it is imperative to study the effects of temperature on the supply of soil N to predict the N availability for plant and microbial growth. Net N mineralization rates, which serve as an index of the supply capacity of soil N, represent only the sum of competing processes, i.e., gross N mineralization and immobilization. However, to gain an advanced insight into the actual N turnover, it is imperative to determine gross N mineralization, namely, nitrification and immobilization, as separate microbial processes (Stark and Hart Citation1997; Han et al. Citation2012). For example, the high rates of gross nitrification in undisturbed forest soils are balanced by those of gross nitrate (NO3 −) immobilization, which results in negligible net nitrification rates (Stark and Hart Citation1997). Higher net N mineralization rates beneath alder {Alnus rugosa (Du Roi) Spreng. [A. incana (L.) Moench]} results from lower microbial immobilization rather than greater gross N mineralization (Stottlemyer and Toczydlowski Citation1999). In addition, specific microbial processes may exhibit different sensitivities to temperature changes (Lang et al. Citation2010). Therefore, the response of net and gross N rates to temperature changes could be different.
To date, the effects of temperature on soil gross N transformation rates have been mainly studied at temperatures between 0 and 20°C, a prevailing condition in temperate regions, and contradictory findings have been observed in temperate regions. Gross N mineralization has been shown to be less sensitive to temperature fluctuations than gross N immobilization in forest soils (Lang et al. Citation2010). However, conflicting results have also been obtained (Stottlemyer and Toczydlowski Citation1999). Unlike temperate regions, subtropical regions are characterized by relatively high air temperatures (ranging from 0 to 35°C). Particularly in summer, the temperature often exceeds 35°C, making it imperative to study the temperature dependence of gross N transformation rates at temperatures as high as 35°C. However, there are few reports showing how gross N transformation rates correlate with temperature in unfertilized forest soils from subtropical regions.
Approximately 34% of China’s forest area is dominated by humid subtropical forests, which play an important role in maintaining local climatic conditions and regulating regional soil-atmosphere exchange of carbon (C) and N (Fang et al. Citation2001; Zhou et al. Citation2006). Coniferous and broad-leaved plants are two widely distributed types of trees in subtropical natural secondary forests. N transformation differs in soils of coniferous forest (CF) and broad-leaved forest (BF) (Zhang et al. Citation2011; Staelens et al. Citation2012), caused by differences in soil environment, microflora and litter properties between these forests (Binkley and Giardina Citation1998; Yin et al. Citation2012). Since C and N are stoichiometrically mobilized from decomposing soil organic matter (McGill and Cole Citation1981; Andersson et al. Citation2000), the temperature sensitivity of ammonium (NH4 +) transformations may depend on the temperature sensitivity of soil organic matter decomposition (Magill and Aber Citation2000; Grenon et al. Citation2004). Therefore, it could be expected that the temperature sensitivity of NH4 + transformation would be different between CF and BF soils because of the development of different quality of soil organic matter under respective dominant vegetation.
This study aims to quantify the simultaneously occurring individual N gross transformation rates in soils of two contrasting (broad-leaved vs. coniferous) subtropical tree species at different temperatures (5, 15, 25 and 35°C), a prevailing condition during four distinct seasons in subtropical regions; it also examines whether the short-term effects of temperature on soil gross N transformation rates vary with forest type. A comparison of the tree-species-specific (CF vs. BF) responses of soil gross N transformation rates with increasing temperature could allow for a more accurate prediction of the effects of temperature changes, which arise from seasonal variation or global warming, on soil N availbility, and thus future forest composition and feedback for the climate system in subtropical forests. Although short-term laboratory studies may not resemble actual in situ conditions, as sieving, 15N addition, and soil moisture manipulation could change the N transformation rates in situ, a mechanistic understanding of the dynamics of soil gross N transformations as a response to soil temperature change could still be obtained.
MATERIALS AND METHODS
Site description and soil analysis
The study sites were located in Yingtan City, Jiangxi Province, China (28°23′N, 117°02′E). The study region is characterized by a typical subtropical monsoon climate, with four distinct seasons. The mean annual precipitation is approximately 1788 mm (30-year average), approximately 50% of which occurs from April to June. The mean annual temperature is 17.6°C, with minimum and maximum monthly average temperatures of 5.6°C in January and 29.3°C in July (30-year average), respectively. The daily maximum temperature during July and August frequently exceeds 36°C.
Soil was sampled from each forest in May 2012; sampling was carried out at three different sites (> 10 km apart) with spatial independence for reliably comparing the temperature sensitivity of gross N transformation between BF and CF types. The three sites were abbreviated as BF1, BF2 and BF3 for BF and as CF1, CF2 and CF3 for CF. The forest area in the studied region is approximately 2.0 × 105 ha (55.1% of the forest coverage rate), approximately 25.9% of which was dominated by evergreen BFs by 2008. CFs (approximately 60 years old) are dominated by Pinus massoniana (Lambo), while BFs (approximately 60 years old) are dominated by Cinnamomum camphora (L.). Both forest soils were classified as hapludults, according to US soil taxonomy (Zhao et al. Citation1988). To obtain a representative soil sample from each site, three cores (0–20 cm) were collected from within four randomly selected subplots (approximately 4 m × 4 m) of four 100 m × 100 m plots established in each forest. Before sampling, the O horizon was removed from the sampling spot. Then, 48 fresh mineral soil samples were pooled per forest to form a composite sample, sieved (using a 2-mm mesh) and split into two subsamples. One subsample was stored at 4°C for incubation studies conducted within 1 week, while the other subsample was air-dried for the determination of the chemical and physical properties of the soil. Fresh leaf litter from CFs and evergreen BFs was collected from forest floors. The litter was dried at 60°C for 24 h and then milled to 0.25 mm before analysis.
15N tracing experiment
Two ammonium nitrate (NH4NO3) treatments were carried out, each with triplicate analysis. In one treatment, ammonium (15NH4NO3) was labeled with 15N at an excess of 10 atom%, while in the other treatment, nitrate (NH4 15NO3) was labeled in a similar manner. In total, 576 flasks of a volume of 250 mL (6 forests × 2 15N treatments × 4 temperatures × 4 sampling points × 3 replicates) were prepared. For each forest soil sample, 20 g of fresh soil was added to the flask. The soils in the sealed flasks were then acclimated to four different temperatures (5, 15, 25 and 35°C) in the laboratory for 1 day. Two milliliters of a 15NH4NO3 or NH4 15NO3 solution was then uniformly added to the soil at a rate of 20 mg N kg−1 soil (oven-dried weight), which is equivalent to a moderate rate of N deposition in the studied region (Cui et al. Citation2012). The final soil moisture content was then adjusted to a water holding capacity of 60% using distilled water. All of the flasks containing soil samples previously incubated for 1 d at 5, 15, 25 or 35°C were sealed and subsequently incubated at the same temperature in the dark for an additional 9 d. All flasks were sealed with silicone rubber stoppers. The samples were aerated by removing the stoppers for 1 h every 2 d, and the water lost was replaced with deionized water as needed. After 0.5 h or 2, 5 or 9 d of incubation, three replicate 15NH4NO3 − and NH4 15NO3 − labeled flasks for each temperature treatment were destructively sampled. The samples were extracted using a 2 M potassium chloride (KCl) solution at a soil:extractant ratio of 1:5 (weight:volume) prior to the determination of the concentrations and isotopic compositions of NH4 + and NO3 −. The solution (in a flask) was placed on a 250-rpm mechanical shaker for 60 min at 25°C. After extraction with KCl, the residual soil was subsequently washed with 150 mL distilled water three times to completely remove NH4 +, NO3 − and soluble organic N, oven-dried at 60°C until it reached a constant weight and ground such that it passed through a 0.15-mm sieve for the 15N analysis of insoluble soil organic N.
Analysis
Soil properties were determined following the Soil Agro-Chemical Analysis procedures (Lu Citation2000). The soil pH was measured in a slurry with a soil:water ratio of 1:2.5 (volume/volume) using a DMP-2 mV-pH detector (Quark Ltd., Nanjing, China). Soil organic C was determined by wet digestion with sulfuric acid (H2SO4)-potassium dichromate (K2Cr2O7), and soil organic N was determined by semi-micro Kjeldahl digestion using selenium (Se), copper sulfate (CuSO4), and potassium sulfate (K2SO4) as catalysts. NH4 + and NO3 − concentrations were determined by colorimetric methods using a continuous-flow analyzer (Skalar San++ System, Breda, The Netherlands). Soil texture was determined using a laser particle characterization analyzer (Beckman Coulter, Los Angeles, CA, USA). Water-soluble organic carbon (SOCW) was determined by extracting 10 g of 50 mL of distilled water for 30 min, followed by analysis on a Multi-CN-3000 total organic C analyzer (Analytik Jena, Germany). Lignin and cellulose were determined using the acid-detergent fiber–sulfuric acid procedure (Rowland and Roberts Citation1994). Briefly, acid-detergent fiber (hereafter called “fiber”) was determined as the fraction remaining after the treatment of the litter with the boiling acid-detergent to hydrolyze protein. This fiber residue was then treated with 72% sulfuric acid to destroy cellulose; cellulose represents the difference between the initial and remaining fractions. Finally, the residue was ignited at 550°C to destroy the remaining organic matter, thereby leaving only the inorganic (ash) fraction; acid-detergent lignin (hereafter called “lignin”) represents the difference in this residue between pre- and post-ignition. The isotopic compositions of NH4 +, NO3 − and insoluble soil organic N were measured using an automated C/N analyzer isotope ratio mass spectrometer (Europa Scientific Integra, UK). For 15N measurements, NH4 + and NO3 − were separated by distillation using magnesium oxide and Devarda’s alloy (Feast and Dennis Citation1996; Lu Citation2000). In detail, a portion of the extract was steam-distilled with magnesium oxide (MgO) to separate NH4 + on a steam distillation system. The sample in the flask was distilled again after the addition of Devarda’s alloy to separate out NO3 −. The liberated ammonia (NH3) was trapped using a boric acid solution. To prevent isotopic cross-contamination between samples, 25 mL of reagent-grade ethanol was added to the distillation flasks and steam-distilled for 3 min between sample distillations (Hauck and Page Citation1982). The trapped N was acidified and converted to ammonium sulfate ((NH4)2SO4) using a 0.005 mol L−1 H2SO4 solution. The H2SO4 solution (containing NH4 +) was then evaporated to dryness at 60°C in an oven and analyzed for 15N abundance.
15N tracing model
The FLUAZ model (based on the 15N tracing technique) was utilized to calculate gross N transformation rates (Mary et al. Citation1998). This model combines a numerical method (Runge–Kutta algorithm, 4th order) for solving the differential system given by the N and 15N mass equations and nonlinear fitting program (Haus–Marquardt algorithm) for optimizing the N rate parameters by minimizing the difference between observed and simulated N and 15N data (amounts and isotopic excess of NH4 + and NO3 −). Measurements of insoluble soil organic 15N are also considered to be much more reliable for preparing 15N balances. The optimal fit of the experimental data was calculated by minimizing the mean weighted error criterion, which is a function of the difference between simulated and measured variables and the experimental variance of the measured variables. In this way, the measured variables with the largest experimental variability had the lowest weight in the optimization procedure (Mary et al. Citation1998). The main N transformations considered in FLUAZ include nitrification, mineralization and immobilization of NH4 + and NO3 −. In this study, gross N rates between d0 and d2, d2 and d5, and d5 and d9 were calculated using the FLUAZ model, and the time-weighted average transformation rates between d0 and d9 were presented. Net mineralization rates were calculated as the difference between the final and initial mineral N concentrations (NH4 + + NO3 −) divided by 9 d. Net nitrification rates were calculated in the same manner as the daily mean NO3 − accumulation. The multiplication factor Q 10, which describes the average increase in each process rate (F) resulting from a temperature (T) increase of 10°C, was calculated by a regression of the four temperatures from two exponential equations:
Statistical analysis
Two-way analyses of variance (ANOVAs) were used to analyze the effects of temperature, forest type and their interaction on the time-weighted average gross N transformation and net N transformation rates. All data were natural-log transformed when necessary to meet the assumptions of normality and homoscedasticity. All statistical analyses were performed using SPSS 13.0 software (SPSS, Chicago, IL, USA). All results were reported as mean ± standard deviation for oven-dried soils.
RESULTS
Soil and litter properties
shows the physical and chemical properties of the studied BF and CF soils; all soils were acidic, and the BF soils had a lower pH than the CF soils (P < 0.001). The organic C, organic N and clay contents were consistently higher in the BF soils than in the CF soils (P < 0.01). The inorganic N pool was dominated by nitrate-nitrogen (NO3 −-N) in the three BF soils and by ammonium-nitrogen (NH4 +-N) in the three CF soils. shows the litter quality characteristics of the BF and CF soils; litter C and lignin contents were significantly higher in CFs than in BFs, whereas litter N and cellulose contents were significantly lower in CFs than in BFs.
Table 1 Physical and chemical characteristics of the broad-leaved and coniferous forest soils studied. Values in brackets are standard deviations (n = 3)
Table 2 Litter quality characteristics of the broad-leaved and coniferous forests. Values in brackets are standard deviations (n = 3)
N transformation rates
and list the gross N transformation rates for each time interval calculated by the FLUAZ model and the time-weighted average gross N rates for the BF and CF soils, respectively. shows the gross and net N transformation rates averaged from the three investigated forest sites for each forest type. Overall, temperature (P < 0.001), forest type (P < 0.001) and their interaction (P < 0.05) significantly affected all N transformation rates (net and gross) except the gross NO3 − immobilization rate.
Table 3 Gross rates of nitrogen (N) mineralization (m), immobilization (i), nitrification (n), ammonium (NH4 +) immobilization (ia) and nitrate (NO3 −) immobilization (in) during the three incubation intervals calculated by the FLUAZ model in the three broad-leaved forest (BF1, BF2 and BF3) soils at different temperatures. The rates for the interval d0–9 (bold) are time-weighted average values of the three sampling intervals
Table 4 Gross rates of nitrogen (N) mineralization (m), immobilization (i), nitrification (n), ammonium (NH4 +) immobilization (ia) and nitrate (NO3 −) immobilization (in) during the three incubation intervals calculated by the FLUAZ model in the three coniferous forest (CF1, CF2 and CF3) soils at different temperatures. The rates for the interval d0–9 (bold) are time-weighted average values of the three sampling intervals
Figure 1 Effects of incubation temperature on ammonium (NH4 +) transformation rates [gross and net nitrogen (N) mineralization, and gross N immobilization] in the (a) broad-leaved forest and (b) coniferous forest soils, and nitrate (NO3 –) transformation rates (gross and net nitrification, and gross NO3 – immobilization) in the (c) broad-leaved forest and (d) coniferous forest soils. Vertical bars indicate standard deviations (n = 3).
![Figure 1 Effects of incubation temperature on ammonium (NH4 +) transformation rates [gross and net nitrogen (N) mineralization, and gross N immobilization] in the (a) broad-leaved forest and (b) coniferous forest soils, and nitrate (NO3 –) transformation rates (gross and net nitrification, and gross NO3 – immobilization) in the (c) broad-leaved forest and (d) coniferous forest soils. Vertical bars indicate standard deviations (n = 3).](/cms/asset/67a878e8-7875-4a95-a149-1c132f31e902/tssp_a_994187_f0001_b.gif)
Both gross N mineralization and immobilization rates were sensitive to temperature, and the rates increased exponentially with increasing incubation temperature (from 5 to 35°C) in the BF and CF soils (R2 > 0.91, P < 0.01; , ). In the BF soil, gross N immobilization rates increased with temperature to a lesser extent than gross N mineralization rates, which resulted in an increase in net N mineralization with temperature (). In contrast, the equally proportional increase in gross N mineralization and immobilization that occurred at 5–25°C led to a lack of change in net N mineralization in response to temperature in CF soils, whereas an increase in the temperature from 25 to 35°C caused a more pronounced increase in gross N mineralization than in gross N immobilization, and thus net N mineralization (). In CF soils, net N mineralization rates at 5–25°C were negligible and significantly lower than those at 35°C (), possibly indicating that CF soils may be more prone to experience temperature constraints (5–25°C) on N availability. The gross and net N mineralization rates and gross NH4 + immobilization rates were consistently higher in BF soils than in CF soils across incubation temperatures. The Q 10 values calculated from 5 to 35°C for gross N mineralization and NH4 + immobilization ranged from 2.16 and 2.77, with no significant difference in the values between the BF and CF soils. Irrespective of the incubation temperature, the gross N mineralization rates were positively correlated with clay content and organic C and N concentrations (R2 > 0.75, P < 0.05), whereas the gross NH4 + immobilization rates were positively correlated with organic C concentration alone (R2 = 0.68, P < 0.05).
In comparison with gross NH4 + immobilization, the FLUAZ model indicated that the immobilization of NO3 − did not occur in any of the six forest soils incubated at any temperature, which resulted in equal rates between gross and net nitrification ( and ). Such results may indicate that ammonium was preferentially assimilated by microbes in these forest soils at all temperatures examined. Gross nitrification rates gradually increased with increasing temperature in the BF soils but were not significantly different from zero in the CF soils incubated at any temperature ( and ; , ). Irrespective of the incubation temperature, a negative correlation was observed between gross nitrification rates and soil pH (R2 > 0.68, P < 0.05), while a positive correlation was observed between gross nitrification rates and clay content (R2 > 0.67, P < 0.05). In general, gross nitrification rates were consistently higher in BF soils than in CF soils at all incubation temperatures, except at 5°C, where no difference in nitrification rates was observed, probably caused by a low-temperature constraint on nitrifying activity. A Q 10 value of 2.34 was calculated from 5 to 35°C for gross nitrification in BF soils.
DISCUSSION
Soil N transformations mediated by microbial processes are dependent on temperature (Dalias et al. Citation2002; Wang et al. Citation2006). Increasing the temperature is expected to increase soil microbial activity, and thus increase microbial-mediated N processes. Similarly, the results of this study showed that gross N mineralization and immobilization rates exponentially increased with increasing temperature (from 5 to 35°C) in BF and CF soils in the subtropical region, which is consistent with the general viewpoint that soil microflora as a whole controls both the release and immobilization of N (Booth et al. Citation2005). However, a difference in the sensitivity of gross N mineralization and immobilization rates to temperature was further observed in both soils studied. The different temperature sensitivity of gross N mineralization and immobilization rates has been reported in other climatic zones. In temperate regions, gross N mineralization has been reported to be less sensitive to temperature fluctuations than gross N immobilization in forest soils, whereas both gross N mineralization and immobilization rates have been reported to demonstrate no sensitivity to temperature in grassland soils within a temperature range of 10 to 15°C (Lang et al. Citation2010). On the other hand, in semi-arid soils, gross N immobilization has been reported to show no influence with temperature changes from 5 to 40°C (Hoyle et al. Citation2006). In arctic soils, by increasing the temperature from 5 to 12°C, a greater effect has been observed on gross N immobilization than gross N mineralization (Binkley et al. Citation1994). Therefore, the response of gross N processes to temperature change is complicated; moreover, the response cannot be described by a single function in models (Murphy et al. Citation2003).
N mineralization and immobilization are related to multienzyme and multicomposition of microbial community processes (Nannipieri and Eldor Citation2009; Chaparro et al. Citation2012). Fungi are believed to be more prevalent than bacteria in acidic soils (Rousk et al. Citation2009). Moreover, they are involved in high efficiency of net N mineralization and low microbial N requirements (Kooijman et al. Citation2009). Therefore, as soil temperature increases to a certain degree, fungi-induced low microbial N demand may be responsible for the lower sensitivity of the gross N immobilization rate than the gross N mineralization rate to soil temperature change.
Mineralization is mainly governed by soil organic N, while immobilization may be regulated by soil C (Bengtson et al. Citation2005; Booth et al. Citation2005). It has been suggested that the response of gross N rates to temperature could be regulated by C substrate availability (Murphy et al. Citation2003). Increasing temperature could be associated with the rapid assimilation of labile C, which constrains the C supply to heterotrophic inorganic N immobilizing microorganisms (Hoyle et al. Citation2006; Cookson et al. Citation2007; Rennenberg et al. Citation2009). Therefore, the balance of gross N mineralization and immobilization may be changed at the expense of immobilization, which results in increasing net N mineralization in the BF soil within a temperature range of 5–35°C and CF soil at temperatures above 25°C. However, a question arises as to why the temperature sensitivity of gross N mineralization and immobilization was similar at the three lower temperatures (5, 15, and 25°C) in the CF soil.
Previous studies have suggested that the temperature sensitivity of microbially mediated NH4 + production processes depends on the temperature sensitivity of soil organic matter decomposition (Magill and Aber Citation2000; Grenon et al. Citation2004). Generally, the decomposition of C and N is slower in CF soils than in BF soils mainly because of the lower chemical quality of litter produced by coniferous tree species (Reich et al. Citation2005). Compared with that of deciduous trees, the litter of coniferous trees often contains a lower amount of nutrients and a higher proportion of recalcitrant compounds (Reich et al. Citation2005) and releases more organic acids (Howard and Howard Citation1990). Soil enzyme activities have been demonstrated to be limited by temperature only when the supply rate of a substrate exceeds its reaction rate (Giardina and Ryan Citation2000). As a result, the low chemical quality of CF litter probably leads to the low temperature sensitivity of C and N mineralization, thereby demanding a higher temperature for the decomposition of soil organic matter. As a result, gross N mineralization rates were more stimulated than gross N immobilization rates only at 35°C rather than at the three lower temperatures (5, 15 and 25°C) in the CF soil. In contrast, a higher chemical quality of BF litter may lead to less resistant decomposition of soil organic matter, thereby not requiring a high temperature demand for its decomposition.
Nitrification has been reported to be more sensitive to temperature changes than mineralization or immobilization (Campbell and Biederbeck Citation1972; Hoyle et al. Citation2006). However, there has been a lack of gross nitrification and net nitrification responses to temperature changes, which is attributed to the limited availability of C and NH4 + (Stottlemyer and Toczydlowski Citation1999; Cookson et al. Citation2002). Therefore, nitrification is sensitive to temperature, provided that available C and NH4 + are not limiting factors. However, our results showed that nitrification in the CF soil does not occur at all incubation temperatures, and thus exhibits no temperature sensitivity, even though organic C and NH4 + concentrations are high in the forest soils. As a result, nitrification in the CF soil may be constrained by factors other than temperature.
Plants may play an important role in regulating N cycling by producing secondary compounds as substrates, inhibitors or inducers for microbial growth. Monoterpenes, a group of secondary metabolites that occur in large amounts mainly in conifers, have been found to inhibit nitrification in CF soils (Uusitalo et al. Citation2008). Therefore, it is likely that nitrification in the CF soil was constrained by secondary metabolites. Alternatively, soil pH is a key factor that controls soil nitrification (Ste-Marie and Paré Citation1999; Yao et al. Citation2011). It has been generally reported that nitrification correlates positively with soil pH (Ste-Marie and Paré Citation1999; Cheng et al. Citation2013). In our previous study on forest soil, we found that nitrification is negligible at the original pH, while with increasing pH, nitrification is significantly stimulated (Cheng et al. Citation2013). Probably, low pH was responsible for the absence of nitrification in the CF soil. However, the question arose as to why BF soils, which had lower pH values (approximately 3.6) than coniferous soils (4.2), had higher nitrification rates.
A possible explanation for the occurrence of high nitrification rates in the BF soil studied is that these soils contain acid-tolerant or even acidophilic nitrifying bacteria (Hayatsu Citation1993; Hayatsu and Kosuge Citation1993). Recently, evidence has been reported that ammonia oxidizing archaea, rather than ammonia oxidizing bacteria, are the dominant ammonia oxidizing species in acidic soils and may be the sole drivers of nitrification in soils with pH < 5.5 (Nicol et al. Citation2008; Prosser and Nicol Citation2012). In addition, acidic pH could be beneficial for the existence of fungi, which can drive heterotrophic nitrification (Zhang et al. Citation2011). Further studies are necessary to understand the specific mechanisms responsible for the absence of nitrification in the CF soil and high nitrification rate in the BF soil.
The significance of the present findings
Our study showed that the gross N mineralization and immobilization rates in both forest soils are sensitive to temperature changes. In the BF soils, gross N immobilization rates increased with temperature to a lesser extent than gross N mineralization rates, which resulted in an increase in the net N mineralization rates with increasing temperature (from 5 to 35°C). By contrast, the equally proportional increase in gross N mineralization and immobilization rates that occurred at 5–25°C led to a lack of change in net N mineralization rates in response to temperature in the CF soils, whereas increasing the temperature from 25 to 35°C resulted in a greater increase in the gross N mineralization rate than in the gross N immobilization rate. Gross nitrification rates gradually increased with increasing temperature in the BF soils; however, these rates were not significantly different from zero in the CF soils incubated at any temperature. Overall, CFs were more prone to experience N limitation and low temperature restriction than BFs, and thus N availability likely limits the annual productivity of CF ecosystems. As increasing temperatures are often coupled with low soil moisture content in situ, which would counteract the effects of temperature, and microbial acclimation change probably experiences long-term warming, the extrapolation of laboratory results to field conditions should be carried out with caution.
ACKNOWLEDGMENTS
This work was supported by grants from the Natural Science Foundation of Jiangsu Province (BK20131045), the National Natural Science Foundation of China (41301238, 41222005), the Research Fund of State Key Laboratory of Soil and Sustainable Agriculture, the Nanjing Institute of Soil Science, Chinese Academy of Science (Y412201421) and the Research Fund of Jiangsu Key Laboratory of Environmental Change & Ecological Construction.
REFERENCES
- Andersson S , Nilsson SI , Saetre P 2000: Leaching of dissolved organic carbon (DOC) and dissolved organic nitrogen (DON) in mor humus as affected by temperature and pH. Soil Biol. Biochem., 32, 1–10. doi:10.1016/S0038-0717(99)00103-0
- Bengtson P , Falkengren-Grerup U , Bengtsson G 2005: Relieving substrate limitation-soil moisture and temperature determine gross N transformation rates. Oikos , 111, 81–90. doi:10.1111/j.0030-1299.2005.13800.x
- Binkley D , Giardina C 1998: Why do tree species affect soils? The warp and woof of tree-soil interactions. Biogeochemistry , 42, 89–106. doi:10.1023/A:1005948126251
- Binkley D , Stottlemyer R , Saurez F , Cortina J 1994: Soil nitrogen availability in some arctic ecosystems in northwest Alaska: responses to temperature and moisture. Ecoscience , 1, 64–70.
- Booth MS , Stark JM , Rastetter E 2005: Controls on nitrogen cycling in terrestrial ecosystems: a synthetic analysis of literature data. Ecol. Monogr., 75, 139–157. doi:10.1890/04-0988
- Campbell CA , Biederbeck VO 1972: Influence of fluctuating temperatures and constant soil moistures on nitrogen changes in amended and unamended loam. Can. J. Soil Sci., 52, 323–336. doi:10.4141/cjss72-044
- Chaparro JM , Sheflin AM , Manter DK , Vivanco JM 2012: Manipulating the soil microbiome to increase soil health and plant fertility. Biol. Fertil. Soils , 48, 489–499. doi:10.1007/s00374-012-0691-4
- Cheng Y , Wang J , Mary B , Zhang J-B , Cai Z-C , Chang SX 2013: Soil pH has contrasting effects on gross and net nitrogen mineralizations in adjacent forest and grassland soils in central Alberta. Canada. Soil Biol. Biochem. , 57, 848–857. doi:10.1016/j.soilbio.2012.08.021
- Cookson WR , Cornforth IS , Rowarth JS 2002: Winter soil temperature (2–15°C) effects on nitrogen transformations in clover green manure amended or unamended soils; a laboratory and field study. Soil Biol. Biochem. , 34, 1401–1415. doi:10.1016/S0038-0717(02)00083-4
- Cookson WR , Osman M , Marschner P , Abaye DA , Clark I , Murphy DV , Stockdale EA , Watson CA 2007: Controls on soil nitrogen cycling and microbial community composition across land use and incubation temperature. Soil Biol. Biochem., 39, 744–756. doi:10.1016/j.soilbio.2006.09.022
- Cui J , Zhou J , Peng Y , He YQ , Chan A 2012: Atmospheric inorganic nitrogen in wet deposition to a red soil farmland in Southeast China, 2005–2009. Plant Soil , 359, 387–395. doi:10.1007/s11104-012-1200-0
- Dalias P , Anderson JM , Bottner P , Coûteaux -M-M 2002: Temperature responses of net nitrogen mineralization and nitrification in conifer forest soils incubated under standard laboratory conditions. Soil Biol. Biochem. , 34, 691–701. doi:10.1016/S0038-0717(01)00234-6
- Fang JY , Chen AP , Peng CH , Zhao SQ , Ci LJ 2001: Changes in forest biomass carbon storage in China between 1949 and 1998. Science , 292, 2320–2322. doi:10.1126/science.1058629
- Feast NA , Dennis PF 1996: A comparison of methods for nitrogen isotope analysis of groundwater. Chem. Geol. , 129, 167–171. doi:10.1016/0009-2541(95)00186-7
- Giardina CP , Ryan MG 2000: Evidence that decomposition rates of organic carbon in mineral soil do not vary with temperature. Nature , 404, 858–861. doi:10.1038/35009076
- Grenon F , Bradley RL , Titus BD 2004: Temperature sensitivity of mineral N transformation rates, and heterotrophic nitrification: possible factors controlling the post-disturbance mineral N flush in forest floors. Soil Biol. Biochem., 36, 1465–1474. doi:10.1016/j.soilbio.2004.04.021
- Han W-Y , Xu J-M , Yi X-Y , Lin Y-D 2012: Net and gross nitrification in tea soils of varying productivity and their adjacent forest and vegetable soils. Soil Sci. Plant Nutr. , 58, 173–182. doi:10.1080/00380768.2012.664783
- Hauck RD 1982: Nitrogen-isotope ration analysis. In Methods of Soil Analysis. Part 2. Chemical and Microbiological Properties, Ed. Page AL , pp. 735–779. Soil Science Society of America, Madison, Wisconsin, USA.
- Hayatsu M 1993: The lowest limit of pH for nitrification in tea soil and isolation of an acidophilic ammonia oxidizing bacterium. Soil Sci. Plant Nutr., 39, 219–226. doi:10.1080/00380768.1993.10416993
- Hayatsu M , Kosuge N 1993: Autotrophic nitrification in acid tea soils. Soil Sci. Plant Nutr., 39, 209–217. doi:10.1080/00380768.1993.10416992
- Howard PJA , Howard DM 1990: Titratable acids and bases in tree and shrub leaf litters. Forestry , 63, 177–196. doi:10.1093/forestry/63.2.177
- Hoyle FC , Murphy DV , Fillery IRP 2006: Temperature and stubble management influence microbial CO2-C evolution and gross N transformation rates. Soil Biol. Biochem., 38, 71–80. doi:10.1016/j.soilbio.2005.04.020
- Kooijman AM , Van Mourik JM , Schilder MLM 2009: The relationship between N mineralization or microbial biomass N with micromorphological properties in beech forest soils with different texture and pH. Biol. Fertil. Soils , 45, 449–459. doi:10.1007/s00374-009-0354-2
- Lang M , Cai Z-C , Mary B , Hao XY , Chang SX 2010: Land-use type and temperature affect gross nitrogen transformation rates in Chinese and Canadian soils. Plant Soil , 334, 377–389. doi:10.1007/s11104-010-0389-z
- Lu RK 2000: Soil Agro-Chemical Analyses. Agricultural Technical Press of China, Beijing. in Chinese.
- Magill AH , Aber JD 2000: Variation in soil net mineralization rates with dissolved organic carbon additions. Soil Biol. Biochem., 32, 597–601. doi:10.1016/S0038-0717(99)00186-8
- Mary B , Recous S , Robin D 1998: A model for calculating nitrogen fluxes in soil using 15N tracing. Soil Biol. Biochem., 30, 1963–1979. doi:10.1016/S0038-0717(98)00068-6
- McGill WB , Cole CV 1981: Comparative aspects of cycling of organic C, N, S, and P through soil organic matter. Geoderma , 26, 267–286. doi:10.1016/0016-7061(81)90024-0
- Murphy DV , Recous S , Stockdale EA , Fillery IRP , Jensen LS , Hatch DJ , Goulding KWT 2003: Gross nitrogen fluxes in soil: theory, measurement and application of 15N pool dilution techniques. Adv. Agron., 79, 69–118. doi:10.1016/S0065-2113(02)79002-0
- Nannipieri P , Eldor P 2009: The chemical and functional characterization of soil N and its biotic components. Soil Biol. Biochem. , 41, 2357–2369. doi:10.1016/j.soilbio.2009.07.013
- Nicol GW , Leininger S , Schleper C , Prosser JI 2008: The influence of soil pH on the diversity, abundance and transcriptional activity of ammonia oxidizing archaea and bacteria. Environ. Microbiol., 10, 2966–2978. doi:10.1111/j.1462-2920.2008.01701.x
- Prosser JI , Nicol GW 2012: Archaeal and bacterial ammonia-oxidisers in soil: the quest for niche specialisation and differentiation. Trends Microbiol., 20, 523–531. doi:10.1016/j.tim.2012.08.001
- Reich PB , Oleksyn J , Modrzynski J , Mrozinski P , Hobbie SE , Eissenstat DM , Chorover J , Chadwick OA , Hale CM , Tjoelker MG 2005: Linking litter calcium, earthworms and soil properties: a common garden test with 14 tree species. Ecol. Lett. , 8, 811–818. doi:10.1111/j.1461-0248.2005.00779.x
- Rennenberg H , Dannenmann M , Gessler A , Kreuzwieser J , Simon J , Papen H 2009: Nitrogen balance in forest soils: nutritional limitation of plants under climate change stresses. Plant Biol., 11, 4–23. doi:10.1111/j.1438-8677.2009.00241.x
- Rousk J , Brookes PC , Bååth E 2009: Contrasting soil pH effects on fungal and bacterial growth suggest functional redundancy in carbon mineralization. Appl. Environ. Microb., 75, 1589–1596. doi:10.1128/AEM.02775-08
- Rowland AP , Roberts JD 1994: Lignin and cellulose fractionation in decomposition studies using acid-detergent fibre methods. Commun. Soil Sci. Plan. , 25, 269–277. doi:10.1080/00103629409369035
- Staelens J , Rütting T , Huygens D , De Schrijver A , Müller C , Verheyen K , Boeckx P 2012: In situ gross nitrogen transformations differ between temperate deciduous and coniferous forest soils. Biogeochemistry , 108, 259–277. doi:10.1007/s10533-011-9598-7
- Stark JM , Hart SC 1997: High rates of nitrification and nitrate turnover in undisturbed coniferous forests. Nature , 385, 61–64. doi:10.1038/385061a0
- Ste-Marie C , Paré D 1999: Soil, pH and N availability effects on net nitrification in the forest floors of a range of boreal forest stands. Soil Biol. Biochem. , 31, 1579–1589. doi:10.1016/S0038-0717(99)00086-3
- Stottlemyer R , Toczydlowski D 1999: Nitrogen mineralization in a mature boreal forest, Isle Royale, Michigan. J. Environ. Qual., 28, 709–720. doi:10.2134/jeq1999.00472425002800020040x
- Uusitalo M , Kitunena V , Smolander A 2008: Response of C and N transformations in birch soil to coniferous resin volatiles. Soil Biol. Biochem., 40, 2643–2649. doi:10.1016/j.soilbio.2008.07.009
- Wang C , Wan S , Xing X , Zhang L , Han X 2006: Temperature and soil moisture interactively affected soil net N mineralization in temperate grassland in Northern China. Soil Biol. Biochem., 38, 1101–1110. doi:10.1016/j.soilbio.2005.09.009
- Williams EJ , Guenther A , Fehsenfeld FC 1992: An inventory of nitric oxide emissions from soils in the United States. J. Geophys. Res., 97, 7511–7519. doi:10.1029/92JD00412
- Yao HY , Campbell CD , Qiao XR 2011: Soil pH controls nitrification and carbon substrate utilization more than urea or charcoal in some highly acidic soils. Biol. Fertil. Soils , 47, 515–522. doi:10.1007/s00374-011-0554-4
- Yin H , Chen Z , Liu Q 2012: Effects of experimental warming on soil N transformations of two coniferous species, Eastern Tibetan Plateau, China. Soil Biol. Biochem., 50, 77–84. doi:10.1016/j.soilbio.2012.03.004
- Zhang JB , Müller C , Zhu TB , Cheng Y , Cai ZC 2011: Heterotrophic nitrification is the predominant NO3- production mechanism in coniferous but not broad-leaf acid forest soil in subtropical China. Biol. Fertil. Soils , 47, 533–542. doi:10.1007/s00374-011-0567-z
- Zhao QG , Xie WM , He XY , Wang MZ 1988: Red Soils of Jiangxi Province (In Chinese with English Abstract). Jiangxi Science and Technology Publishing House, Nanchang.
- Zhou GY , Liu SG , Li ZA , Zhang DQ , Tang XL , Zhou CY , Yan JH , Mo JM 2006: Old-growth forests can accumulate carbon in soils. Science , 314, 1417. doi:10.1126/science.1130168