Abstract
To understand the effects of reforestation on soil carbon (C) dynamics, we measured soil carbon dioxide (CO2) effluxes and soil C pools in dragon spruce (Picea asperata Mast.) stands of various ages (22-, 47- and 68-year-old dragon spruce plantations, and a 150-year-old primeval coniferous forest) in the eastern Tibet Plateau. The soil respiration rate of all the stands increased from March, followed by a peak in late July to August with temporal fluctuations, and then dramatically declined in September. The annual total soil CO2 effluxes (Rtot), soil heterotrophic CO2 effluxes (Rh), and soil autotrophic CO2 effluxes (Ra) were higher in the 22- (1004, 656 and 348 g C m−2 year−1, respectively) and 150-year-old stands (1070, 712 and 358 g C m−2 year−1, respectively), and lower in the 47- (792, 543 and 249 g C m−2 year−1, respectively) and 68-year-old stands (952, 607 and 345 g C m−2 year−1, respectively). The soil total organic carbon (TOC), easily oxidizable organic C (EOC), microbial biomass C (MBC), and particulate organic C (POC) pools at depths ranging from 0 cm to 30 cm decreased within 68 years after tree planting. The TOC and its different components in the 0 cm to 10 cm soil layer were significantly and positively related with Rh and Rtot (p < 0.01). The TOC contents (0 cm to 10 cm soil layer) in the primary forest (77.6 g kg−1) were about 1.87, 2.51 and 3.14 times higher than those in the 22-, 47- and 68-year-old plantations, respectively. Our results indicated soil respiration and soil C contents firstly decreased but then increased with stand age, and 68 years of reforestation has caused substantial depletion of soil CO2 effluxes and carbon pools.
1. INTRODUCTION
Soil carbon (C) accumulation is defined as C inputs (litterfall, root mortality and exudates, and transport of dissolved organic C) exceeding C outputs (soil respiration and leaching). Soil respiration is the primary path by which carbon dioxide (CO2) fixed by land plants returns to the atmosphere in forest ecosystems (Raich and Schlesinger Citation1992; Janssens et al. Citation2001; Saiz et al. Citation2006). Soil respiration is an important component of the forest C balance (accounting for 60 to 80% of total ecosystem respiration), is always a critical factor in global C cycling in terrestrial ecosystems and greatly influences interannual variations in net ecosystem production (Law et al. Citation1999; Tedeschi et al. Citation2006). Soil respiration is sensitive to climate, vegetation type and soil properties (Raich and Potter Citation1995; IPCC Citation2001). However, the effects of forest succession on C fluxes are not yet clearly understood (Tang et al. Citation2009). Soil respiration varies with the structure and age of stands (Klopatek Citation2002). Studies have shown that soil respiration decreases with stand age in the first-rotation chronosequence of Sitka spruce (Picea sitchensis (Bong.) Carr.) in central Ireland (Saiz et al. Citation2006), a chronosequence of 17-year-old coppiced oak (Quercus cerris L.) in central Italy (Tedeschi et al. Citation2006), and a 40-year-old Douglas fir (Pseudotsuga menziesii var. menziesii) plantation (Klopatek Citation2002). On the contrary, other studies have reported that soil respiration increases with stand age in a loblolly pine (Pinus taeda L.) chronosequence ranging from 1 to 25 years old (Wiseman and Seiler Citation2004), and a poplar (Populus deltoids cv.) plantation chronosequence 3, 8 and 15 years old (Yan et al. Citation2011). The stands investigated by most previous studies on soil respiration were generally less than 50 years old, which raises the question: how does soil respiration change with forest age in much older stands?
Soil respiration includes autotrophic and heterotrophic respiration. Respiration by roots, associated mycorrhizal fungi and other micro-organisms is considered soil autotrophic respiration, and respiration by microbes and soil fauna is considered heterotrophic respiration. Understanding soil respiration components and their contributions is an essential step in understanding and modeling C cycling in forest ecosystems (Wang and Yang Citation2007).
Generally, soil respiration is positively correlated with soil temperature in forest ecosystems, and is significantly influenced by the interactions between soil temperature and water content (Wang et al. Citation2006). Previous studies have shown that the seasonal patterns of soil respiration are similar to those of soil temperature – that is, an increase in spring followed by a peak in June to July and a decline in autumn (Tang et al. Citation2009). Soil respiration is closely related to soil C storage, particularly soil organic carbon (SOC) (Wang et al. Citation2006). In forest ecosystems, total soil organic carbon (TOC) is composed of different SOC components, such as easily oxidizable organic carbon (EOC), microbial biomass carbon (MBC), particulate organic carbon (POC) and so on (Yang et al. Citation2005). Many studies have indicated that soil C storage is influenced by forest harvest, disturbance, afforestation, reforestation and stand age (Guo and Gifford Citation2002; Rothstein et al. Citation2004; Shirato et al. Citation2004; Tang et al. Citation2009). However, few studies have focused on the changes in TOC and its components, and the relationship of TOC with soil respiration across various stand ages.
Subalpine coniferous forests are dominated by Picea asperata Mast. and Abies faxoniana Rehd., which are mainly distributed along the eastern edges of the Qinghai-Tibetan plateau, particularly along the upper reaches of the Yangtze River. As the second-largest forest biome in China, subalpine coniferous forests were a main source of timber from the 1930s to the 1990s. In recent decades, large-scale logging has turned vast patches of these montane forests into clear-felled areas. Picea asperata Mast., or dragon spruce, is widely used to reforest such areas. As a result, a monoculture of spruce plantations with a wide range of stand ages was established in a number of subalpine coniferous areas, thereby providing ideal field experimental bases for ecological studies on forest chronosequencing. A previous study has shown that forest management practices (deforestation and reforestation) dramatically decrease the soil C pool in this region (Xu et al. Citation2010). However, the dynamics of soil respiration and soil C pool across a range of spruce stand ages in this region have been rarely reported.
In this study, the chronosequence includes 22-, 47- and 68-year-old plantations and a 150-year-old primeval forest, which are much older than previously studied plantations (Ewel et al. Citation1987; Klopatek Citation2002; Saiz et al. Citation2006; Xiang et al. Citation2009; Takahashi et al. Citation2011). The specific purposes of this study were to: (1) compare soil autotrophic, heterotrophic and total respiration, as well as soil C pools among forests of different ages in this region; (2) determine the relationship of soil respiration and it components to SOC.
2. MATERIAL AND METHODS
2.1 Study site
The study sites are located at Miyaluo, Lixian County, Aba (Ngawa) Tibetan, and Qiang Autonomous Prefecture, western Sichuan Province, China, at 31°47´N, 102°42´E, and at an altitude range of 3100–3400 m. This area is located at the southeastern edge of the Qinghai-Tibetan plateau, and is part of the watershed of the upper reaches of the Zagunao River, a tributary of Minjiang River. The climate is classified as montane monsoon – that is, humid and rainy in summer with drought and cold in winter. Average annual air temperature and precipitation measured at a nearby weather station are 7.27°C and 687.3 mm, respectively, with a range of 0.54°C and 8.2 mm, respectively (Liu Citation2002). Soils at the study sites are classified as mountain brown soil (CSTRGISSCAS Citation1995), and Aqualfs (NRCS Citation2006).
Four stand age classes – that is, 22-, 47-, 68- and 150-year-old stands – were chosen for this study in 2007. Each stand typically covered more than 1 ha, and all the stands were within a 15-km radius. The 150-year-old growth stand was a patch of residual primeval coniferous forest dominated by dragon spruce (P. asperata), mixed with faxon fir (A. faxoniana) and broadleaf trees (Betula albo-sinensis Burk., Betula utilis D. Don, and so on). The other three stands were monocultures of spruce (mostly P. asperata) plantations initially established in 1939, 1960 and 1985, respectively, after deforestation. The four stands of different ages were natural growth, undisturbed by human activities, and detailed information is given in .
Table 1 Physical and stand characteristics of a subalpine coniferous forest of four ages in Miyaluo region, western Sichuan province, China
2.2 Experimental design
The experimental design included four different age groups (22-, 47- and 68-year-old pure P. asperata plantations, and one 150-year-old primeval forest). Three sites (20 m × 30 m) per age stand were randomly selected for experimental measurements in this study. Leaf area index (LAI) was measured in late June along a 50-m transect in the stands, using an LAI-2000 (LI-COR Inc., Lincoln, NE, USA).
Four plots of 50 cm × 50 cm in size within each site were also randomly set up in early August 2007. Trenches 50 cm deep were dug along the edges of the plots because few roots were found at a depth of 50 cm (Wang et al. Citation2006). Trenches were lined with a double layer of plastic sheets and then refilled and packed carefully with the soil. We then carefully removed all aboveground vegetation with minimal soil disturbance and kept the trenched plots free of living vegetation throughout the study. One polyvinyl chloride (PVC) collar (10.2 cm inside diameter × 6 cm height) was installed in each trenched plot to measure soil heterotrophic respiration (Rh). To eliminate the early responses of soil respiration to trenching, we started measuring soil respiration 3 months after plot trenching (November 2007). Meanwhile, nine other untrenched plots with PVC collars were set up in each site to measure total soil respiration (Rtot).
2.3 Measuring soil temperature and water content
Soil temperature and water content were measured both discretely and continuously, as described by Wang and Yang (Citation2007). Discrete measurements of soil temperature were made concurrently with soil respiration measurements at a depth of 5 cm (T5) near each collar, using a digital long-stem thermometer. At the same time, soil water content at a depth of 5 cm (W5) was also measured using a hand-held probe (IMKO, Germany). T5 and W5 were continuously measured and recorded at 30-min intervals using dataloggers (Campbell AR5, Avalon, USA) installed in the 68-year-old stand.
2.4 Measuring soil respiration determination and estimating annual CO2 flux
Soil CO2 fluxes of trenched plots (Rh) and untrenched plots (Rtot) were measured every other week during November 2007 and October 2008, using a Li-Cor 6400 portable CO2 infrared gas analyzer equipped with a Li-6400–09 chamber (Li-Cor Inc., Lincoln, NE, USA). The instantaneous rate of soil respiration was measured continuously for five cycles, and the five measurements were averaged to produce a collar’s mean rate of total soil respiration and soil heterotrophic respiration. To minimize diurnal variation in soil respiration, measurements were made from 10:00 a.m. to 2:00 p.m. within 3 days.
As in previous studies (Sims and Bradford Citation2001; Wang et al. Citation2006; Wang and Yang Citation2007), annual total soil respiration (Rtot) and heterotrophic respiration (Rh) for each stand were estimated by interpolating measured soil respiration between sampling dates for every day of the year, and then computing the sum to determine the annual soil total CO2 flux. Annual autotrophic respiration (Ra) was calculated as the difference between Rtot and Rh (Ra = Rtot – Rh).
2.5 Measuring SOC and litter accumulation
We cored 10 soil samples around the areas in each fixed plot where soil respiration was measured. The samples were 7.6 cm in diameter and taken at depths of 0–10 cm, 10–20 cm and 20–30 cm in November 2007. Each sample was passed through a sieve (2 mm mesh size), and any visible living plant material was manually removed from the sieved soil. Soil samples were analyzed for the concentrations of TOC, EOC, MBC and POC. At the same time, four plots (50 cm × 50 cm) were randomly selected in each site, and litter was collected from each plot and dried to determine litter accumulation on the forest floor.
The EOC was determined according to Blair et al. (Citation1995). Air-dried soil samples were reacted with a potassium permanganate (KMnO4) solution for 1 h and the amount of EOC was determined spectrophotometrically from the amount of KMnO4 reduced. POC (53–2000 μm) was determined following Cambardella and Elliott (Citation1992). MBC was estimated using a 20-g oven-dried equivalent of fresh soil sample by fumigating the sample with ethanol-free chloroform (CHCl3) and extraction using 0.5 M potassium sulfate (K2SO4), as described by Vance et al. (Citation1987). The TOC concentration in the soil extractant was measured using a MultiN/C2100TOC/TN (AnalytikJena AG, Germany) determined by means of oxidation with potassium dichromate (K2Cr2O7) (Vance et al. Citation1987). MBC was calculated by using a conversion factor (kc) of 0.35 (Yang et al. Citation2005).
2.6 Statistical analysis
Pearson two-tailed test was performed to examine the correlation between annual soil respiration flux (Rtot, Rh and Ra) and soil C contents at different depths, soil temperature, water content, LAI and litter accumulation. Multiple comparisons were used to identify whether the determined parameters of the various stands significantly differed at the 0.05 probability level. SPSS 12.0 (SPSS Inc. Chicago, IL, USA) was used for all statistical analyses.
3. RESULTS
3.1 Soil temperature, moisture content, LAI and litter accumulation
Daily mean soil temperatures at 5 cm depth of four age stands from November 2007 to October 2008 are shown in . The lowest soil temperature was observed in January and February 2008, and the highest temperature was observed in July 2008 in all stands. No obvious difference of soil temperature was observed in 22-, 68- and 150-year old forests; however, the soil temperature of the 47-year old stand from April to October was lower than that of the other three stands. The melting of the snow (April, May and June) and rainy season (August, September and October) resulted in higher soil water contents in all the stands during these periods.
Figure 1 Seasonal changes in soil temperature and water content at 5 cm depth for forests of different age during 2007 and 2008. The error bars represent standard errors (n = 3).
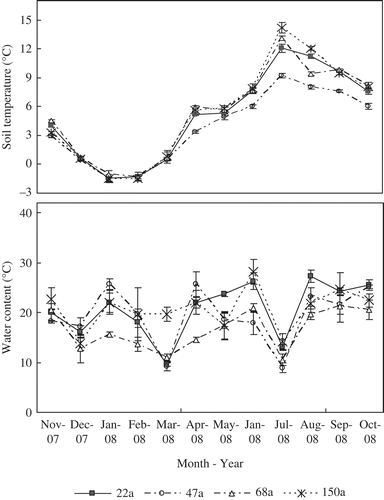
LAI increased as the stand age increased, as demonstrated by the 22-year-old (1.56 ± 0.19 m2 m−2) to the 47-year-old (2.38 ± 0.04 m2 m−2) stands, then decreased and remained at the same level as the stand ages (). The litter accumulation in the examined stands increased as the stand age increased ().
3.2 Seasonal patterns of soil respiration rate and annual soil CO2 flux
The seasonal patterns of soil respiration rate were similar to the patterns of soil temperature. As a general trend, soil total, heterotrophic and autotrophic respirations increased in March (spring), reached their initial peaks with temporal fluctuations from late July to August (late summer), and then gradually decreased in September (autumn), and maintained their low levels for about 4 months (). However, although the patterns of soil respiration in the 22-year-old stand were similar to those of the other three stands, the monthly variations here were more dramatic in July and September.
Figure 3 Seasonal pattern of total, heterotrophic and autotrophic soil respiration rate, annual carbon flux (Rtot, Rh and Ra) and the contributions in the four ages of dragon spruce (Picea asperata Mast.) forests (n = 3). CO2: carbon dioxide.
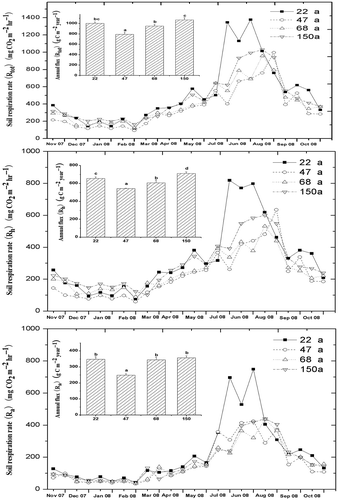
The annual soil total, heterotrophic and autotrophic CO2 fluxes (Rtot, Rh and Ra) had similar trends across various stand ages (). The annual Rtot, Rh and Ra were 1004, 656 and 348 g C m−2 yr−1 in the 22-year-old stand, respectively; 792, 543 and 249 g C m−2 yr−1 in the 47-year-old stands respectively; 952, 607 and 345 g C m−2 yr−1 in the 68-year-old stand, respectively; and 1070, 712 and 358 C m−2 yr−1 in the 150-year-old stand. Therefore, the annual Rtot, Rh and Ra of the 47-year-old stand were lower than the parameters of the other three forest stand ages (). The 150-year-old stand exhibited the highest annual Rtot, Rh and Ra among all stands. There were no significant differences of Rh/Rtot among all the stands. However, the contribution of Rh to Rtot was higher in the 47-year-old stand (68.2%) and lower in the 68-year-old stand (63.4%) ().
3.3 Soil organic C concentrations
The soil C contents in the stands of all age groups decreased as the depth of the soil decreased, particularly in the 150-year-old primeval stand. Compared to the primary forest, TOC and its components were significantly lower in the three younger stands, especially in the upper soil layers (0 – 10; ). The TOC content (0 – 10 cm soil layers) in the primary forest (77.6 g kg−1) was about 1.87, 2.51 and 3.14 times higher than those in the 22-, 47- and 68-year-old plantations, respectively. Soil MBC, EOC and POC contents of the primary forest in the upper layer were also much lower than those in the three plantations. Compared to the primeval stands, the decrements of TOC concentration in 10–20 cm soil layers were 5.1, 16.3 and 19.4 g kg −1 in the 22-, 47- and 68-year-old plantations, respectively. TOC concentration in the 20–30 cm soil layer was only decreased in the 47- and 68-year-old plantations by 3.4 and 11.9 g kg−1, respectively. In the 10–20- and 20–30-cm soil layers, higher contents of MBC, EOC and POC were usually observed both in the 150-year-old primary forest and the 22-year-old stand, and lower contents were observed in the other two plantations, particularly in the 68-year-old plantation.
4. DISCUSSION
4.1 Soil respiration of spruce chronosequence
Although a number of studies suggested that soil respiration decreased with stand age in temperate forests (Wang et al. Citation2002; Saiz et al. Citation2006; Tedeschi et al. Citation2006), other studies reported that soil respiration increased with stand age in tropical and subtropical forests (Law et al. Citation2003; Ewel et al. Citation1987). However, these studies only investigated the soil respiration of approximately 40-year-old plantations (Klopatek Citation2002). In the present study, soil respiration might decrease first from the 22- to 47-year-old forests, and then increase with stand age. Saiz et al. (Citation2006) also reported that soil respiration initially decreased with stand age because of increased productivities, but remained constant in the old stands in the spruce chronosequence composed of 10-, 15-, 31- and 47-year-old stands. It is reported that the variations of soil respiration were dominated by soil temperature, moisture, vegetation, litter accumulation and soil carbon availability (Tedeschi et al. Citation2006; Tang et al. Citation2009; Butler et al. Citation2012; Prolingheuer et al. Citation2014). In this study, soil temperature and soil C pools (upper layer) might be mainly responsible for the changes of soil respiration in the spruce chronosequence, since the two factors were significantly related to soil respiration ().
Table 2 Correlation coefficients of factors Total organic carbon (TOC), Easily oxidizable organic carbon (EOC), Microbial biomass carbon (MBC), Particulate organic carbon (POC), Leaf area index (LAI) and litter accumulation with annual total soil carbon flux (R tot), heterotrophic soil carbon flux (R h) and autotrophic carbon flux (R a)
Soil respiration and its components varied with stand ages in the subalpine dragon spruce forest. Soil heterotrophic respiration (Rh) is mainly controlled by soil environment and substrate availability (Wang and Yang Citation2007), and the changes in Rh largely reflected the ability of leaf litter decomposition (Cisneros-Dozal et al. Citation2006). In the present study, annual Rh initially decreased, and then increased with stand age. The variation of annual Rh across stand age was mostly induced by SOC availability. In addition, heterotrophic respiration was sensitive to soil temperature, litter accumulation and LAI (Hartley et al. Citation2007; Prolingheuer et al. Citation2014). The high LAI of the 47-year-old stands was likely to decrease soil respiration rate by reducing soil temperature (3.84°C) as a result of creating shade, or to directly decrease soil respiration by shade (Hartley et al. Citation2007). Thus, the combined actions of lower soil temperature and higher LAI might contribute to the lower annual Rh in the 47-year-old stand. On the other hand, the higher levels of litter accumulation and soil C pools might play an important role in the higher annual Rh in the 150-year-old primeval stand.
The annual Ra decreased with stand age in the younger plantations (below 47 years old) given that the autotrophic respiration rates decreased as the plantations grew, as a result of increased activities in the young plantations (Ohashi et al. Citation2000). However, contrary to a previous study (Saiz et al. Citation2006), a lack of significant difference was observed among the 22-year-old and older (68- and 150-year-old) stands. This lack of difference might be because the oldest age stand in the previous study was 47 years old (Saiz et al. Citation2006), which was significantly younger than the stands in the present study. On the other hand, a large range of values for the relative contribution of autotrophic soil respiration to total soil respiration has been reported, from 22% to as much as 90% in the literature (Hanson et al. Citation2000). Ra/Rtot ranged from 31.4 to 36.8% by calculation in the present study. The contribution of autotrophic respiration ranged widely from forest to forest given the different tree species, climates and methods of forest management (Saiz et al. Citation2006; Wang and Yang Citation2007; Yan et al. Citation2011). The higher Ra/Rtot was always observed in warmer and wet sites (Malhi et al. Citation1999; Metcalfe et al. Citation2007; Butler et al. Citation2012).
4.2 Relationship of soil respiration to SOC components, LAI and litter accumulation
Soil respiration always depends on the amount of fine roots, and the SOC levels (Wang et al. Citation2006; Wang and Yang Citation2007). Our results showed that the annual Rtot and Rh were positively correlated with TOC, EOC, MBC and POC concentration in soils at 0–10 cm depth (). However, although soil heterotrophic respiration was mostly affected by soil C availability, and largely reflected the input of labile C (Ryan and Law Citation2005; Caprez et al. Citation2012), soil respirations were also dominated by soil temperature. In this study, the soil temperature in the 47-year-old stand was lower due to increased LAI, and soil C contents were lower in both 47- and 68-year-old stands. Therefore, the lowest annual Rtot and Rh were observed in the 47-year-old plantation, owing to the added influences of lower temperature and soil C contents. By contrast, because Ra was always affected by plant species and phenology (Fu et al. Citation2002), the correlation between annual Ra and all SOC components was not significant ().
A strong positive correlation among plant photosynthesis, leaf area, primary productivity and soil respiration was reported (Högberg et al. Citation2001; Davidson et al. Citation2002; Yuste et al. Citation2004; Tang et al. Citation2005). However, LAI, which is an important index of forest production, was negatively correlated with annual Rtot, Rh and Ra in all stands in this study. This discrepancy might be because LAI was correlated with gross primary production (GPP), which could drive respiration over a short period of time (days to months; Högberg et al. Citation2001; Tang et al. Citation2005); increased soil organic C and microbial C pools had stronger effects on soil respiration than LAI (Tang et al. Citation2009). On the other hand, high LAI might result in low soil temperature by reducing solar radiation on the forest floor, and then negatively affect the soil respiration in the plantation, as we discussed earlier. Similar to our results, shade caused by high canopy coverage was reported to significantly reduce soil respiration by changing soil carbon pools (Hartley et al. Citation2007).
Yan et al. (Citation2006) found that the monthly CO2 efflux from soil and litter linearly correlated with the monthly litter input in three forest ecosystems in Dinghushan Biosphere Reserve, Southern China. In our study, litter accumulation increased with stand ages () and only correlated with annual Rh in the stands of all age groups (). This result suggests that the balance between soil C inputs (litterfall, root mortality and exudates, and transport of dissolved organic C) and outputs (decomposition) is complex, and also affected by vegetation type, stand age, soil temperature and moisture (Wang et al. Citation2006; Yan et al. Citation2006).
In conclusion, soil respiration and SOC contents first decreased and then increased with stand age, and the primary forests exhibited the highest level of soil respiration and SOC among the subalpine coniferous forests. This study showed that annual Rtot and Rh were positively related to the content of SOC and its components in upper soil layer (0–10 cm). LAI and litter accumulation of the forest floor also affected soil respiration. Our results suggest that although the reforested ecosystem was developed for approximately 70 years, its soil respiration and soil C pool were far less than those of the primary forest, particularly the SOC contents of the 0–10 cm soil layers. However, a limitation of this study is that the observations on soil respiration and SOC contents across the stand ages were conducted only in 1 year. Long-term experiments are needed to further investigate the effects of reforestation and forest succession on the dynamics of soil respiration and the C pool.
Acknowledgments
This study was funded jointly by the Key Program of the National Natural Science Foundation of China (Nos. 31100446 and 31270552), and the Strategic Priority Research Program of the Chinese Academy of Sciences (No. XDA01050303).
References
- Blair GJ , Lefroy RD , Lisle L 1995: Soil C fractions based on their degree of oxidation and the development of a C management index for agricultural system. Aust. J. Agric. Resour. Econ. ., 46, 1459–1466. doi:10.1071/AR9951459
- Butler A , Meir P , Saiz G , Maracahipes L , Marimon BS , Grace J 2012: Annual variation in soil respiration and its component parts in two structurally contrasting woody savannas in central brazil. Plant Soil ., 352, 129–142. doi:10.1007/s11104-011-0984-7
- Cambardella CA , Elliott ET 1992: Particulate soil organic matter changes across a grassland cultivation sequence. Soil Sci. Soc. Am. J ., 56, 777–778. doi:10.2136/sssaj1992.03615995005600030017x
- Caprez R , Niklaus PA , Körner C 2012: Forest soil respiration reflects plant productivity across a temperature gradient in the Alps. Oecologia , 170 (4), 1143–1154. doi:10.1007/s00442-012-2371-3
- Chinese Soil Taxonomy Research Group, Institute of Soil Science, Chinese Academy of Sciences (CSTRGISSCAS) 1995: Chinese Soil Taxonomy (Revised Proposal), Chinese Agricultural Science and Technology Press, Beijing. (in Chinese)
- Cisneros-Dozal LM , Trumbore S , Hanson PJ 2006: Partitioning sources of soil-respired CO2 and their seasonal variation using a unique radiocarbon tracer. Glob. Change Biol ., 12, 194–204. doi:10.1111/j.1365-2486.2005.001061.x
- Davidson EA , Savage K , Bolstad P , et al. 2002: Belowground carbon allocation in forests estimated from litterfall and IRGAbased soil respiration measurements. Agr. Forest Meteorol ., 113, 39–51. doi:10.1016/S0168-1923(02)00101-6
- Ewel KC , Cropper Jr. WP , Gholz HL 1987: Soil CO2 evolution in Florida slash pine plantations. I. Changes through time. Can. J. Forest Res ., 17, 325–329. doi:10.1139/x87-054
- Fu SL , Cheng WX , Susfalk R 2002: Rhizosphere respiration varies with plant species and phenology: a greenhouse pot experiment. Plant Soil ., 239, 133–140. doi:10.1023/A:1014959701396
- Guo LB , Gifford RM 2002: Soil carbon stocks and land use change: a meta analysis. Glob. Change Biol ., 8, 345–360. doi:10.1046/j.1354-1013.2002.00486.x
- Hanson PJ , Edwards NT , Garten CT , Andrews JA 2000: Separating root and soil microbial contributions to soil respiration: a review of methods and observations. Biogeochemistry , 48, 115–146. doi:10.1023/A:1006244819642
- Hartley IP , Heinemeyer A , Ineson P 2007: Effects of three years of soil warming and shading on the rate of soil respiration: substrate availability and not thermal acclimation mediates observed response. Glob. Change Biol ., 13, 1761–1770. doi:10.1111/j.1365-2486.2007.01373.x
- Högberg P , Nordgren A , Buchmann N , Taylor AF , Ekblad A , Högberg MN , Nyberg G , Ottosson-Löfvenius M , Read DJ 2001: Large-scale forest girdling shows that current photosynthesis drives soil respiration. Nature , 411, 789–792. doi:10.1038/35081058
- IPCC 2001: Climate Change 2001: the Scientific Basis, Cambridge University Press, Cambridge, UK.
- Janssens IA , Lankreijer H , Matteucci G , et al. 2001: Productivity overshadows temperature in determining soil and ecosystem respiration across European forests. Glob. Change Biol ., 7, 269–278. doi:10.1046/j.1365-2486.2001.00412.x
- Klopatek JM 2002: Belowground carbon pools and processes in different age stands of Douglas-fir. Tree Physiol ., 22, 197–204. doi:10.1093/treephys/22.2-3.197
- Law BE , Ryan MG , Anthoni PM 1999: Seasonal and annual respiration of a ponderosa pine ecosystem. Glob. Change Biol ., 5, 169–182. doi:10.1046/j.1365-2486.1999.00214.x
- Law BE , Sun OJ , Campbell J , Van Tuyl S , Thornton PE 2003: Changes in carbon storage and fluxes in a chronosequence of ponderosa pine. Glob. Change Biol ., 9, 510–524. doi:10.1046/j.1365-2486.2003.00624.x
- Liu Q 2002: Ecological research on subalpine coniferous forests in China, 33–52, Sichuan University Press, Chengdu. (in Chinese)
- Malhi Y , Baldocchi DD , Jarvis PG 1999: The carbon balance of tropical, temperate and boreal forests. Plant Cell Environ ., 22, 715–740. doi:10.1046/j.1365-3040.1999.00453.x
- Metcalfe DB , Meir P , Leoc A , Malhi Y , Acl DC , Braga A , Phl G , De Athaydes J , De Almeida SS , Williams M 2007: Factors controlling spatio-temporal variation in carbon dioxide efflux from surface litter, roots, and soil organic matter at four rain forest sites in the eastern Amazon. J. Geophys. Res. . 112. (G04001).
- Natural Resources Conservation Service (NRCS) 2006: Keys to soil taxonomy, United States Department of Agriculture. (Tenth Edition) http://soils.usda.gov/
- Ohashi M , Gyokusen K , Saito A 2000: Contribution of root respiration to total soil respiration in a Japanese cedar (Cryptomeria japonica D. Don) artificial forest. Ecol. Res ., 15, 323–333. doi:10.1046/j.1440-1703.2000.00351.x
- Prolingheuer N , Scharnagl B , Grafa A , Vereecken H , Herbsta M 2014: On the spatial variation of soil rhizospheric and heterotrophic respiration in a winter wheat stand. Agr. Forest Meteorol . doi:10.1016/j.agrformet
- Raich JW , Potter CS 1995: Global patterns of carbon-dioxide emissions from soils. Global Biogeochem. Cy ., 9, 23–36. doi:10.1029/94GB02723
- Raich JW , Schlesinger WH 1992: The global carbon dioxide flux in soil respiration and its relationship to vegetation and climate. Tellus B ., 44, 81–99. doi:10.1034/j.1600-0889.1992.t01-1-00001.x
- Rothstein DE , Yermakov ZY , Al B 2004: Loss and recovery of ecosystem carbon pools following stand-replacing wildfire in Michigan jack pine forests. Can. J. Forest Res ., 34, 1908–1918. doi:10.1139/x04-063
- Ryan MG , Law BE 2005: Interpreting, measuring, and modeling soil respiration. Biogeochemistry , 73, 3–27.
- Saiz G , Byrne KA , Butterbach-Bahl K , Kiese R , Blujdea V , Farrell EP 2006: Stand age-related effects on soil respiration in a first rotation Sitka spruce chronosequence in central Ireland. Glob. Change Biol ., 12, 1007–1020. doi:10.1111/j.1365-2486.2006.01145.x
- Shirato Y , Taniyama I , Zhang T 2004: Changes in soil properties after afforestation in Horqin Sandy Land, North China. Soil Sci. Plant Nutr ., 50, 537–543. doi:10.1080/00380768.2004.10408510
- Sims PL , Bradford JA 2001: Carbon dioxide fluxes in a southern plains prairie. Agr. Forest Meteorol ., 109, 117–134. doi:10.1016/S0168-1923(01)00264-7
- Takahashi M , Hirai K , Limtong P , Leaungvutivirog C , Panuthai S , Suksawang S , Anusontpornperm S , Marod D 2011: Topographic variation in heterotrophic and autotrophic soil respiration in a tropical seasonal forest in Thailand. Soil Sci. Plant Nutr ., 57, 452–465. doi:10.1080/00380768.2011.589363
- Tang JW , Baldocchi DD , Xu L 2005: Tree photosynthesis modulates soil respiration on a diurnal time scale. Glob. Change Biol ., 11, 1298–1304. doi:10.1111/j.1365-2486.2005.00978.x
- Tang JW , Bolstad PV , Martin JG 2009: Soil carbon fluxes and stocks in a Great Lakes forest chronosequence. Glob. Change Biol ., 15, 145–155. doi:10.1111/j.1365-2486.2008.01741.x
- Tedeschi V , Rey A , Manca G , Valentini R , Jarvis PG , Borghetti M 2006: Soil respiration in a Mediterranean oak forest at different developmental stages after coppicing. Glob. Change Biol ., 12, 110–121. doi:10.1111/j.1365-2486.2005.01081.x
- Vance ED , Brookes PC , Jenkinson DS 1987: An extraction method for measuring soil microbial biomass C. Soil Biol. Biochem ., 19, 703–707. doi:10.1016/0038-0717(87)90052-6
- Wang CK , Bond-Lamberty B , Gower ST 2002: Soil surface CO2 flux in a boreal black spruce fire chronosequence. J. Geophys. Res ., 107, 1–8.
- Wang CK , Yang JY 2007: Rhizospheric and heterotrophic components of soil respiration in six Chinese temperate forests. Glob. Change Biol ., 13, 123–131. doi:10.1111/j.1365-2486.2006.01291.x
- Wang CK , Yang JY , Zhang QZ 2006: Soil respiration in six temperate forests in China. Glob. Change Biol ., 12, 2103–2114. doi:10.1111/j.1365-2486.2006.01234.x
- Wiseman PE , Seiler JR 2004: Soil CO2 efflux across four age classes of plantation loblolly pine (Pinus taeda L.) on the Virginia Piedmont. For. Ecol. Manage ., 192, 297–311. doi:10.1016/j.foreco.2004.01.017
- Xiang W , Chai H , Tian D , Peng C 2009: Marginal effects of silvicultural treatments on soil nutrients following harvest in a Chinese fir plantation. Soil Sci. Plant Nutr ., 55, 523–531. doi:10.1111/j.1747-0765.2009.00384.x
- Xu Z , Wan C , Xiong P , Tang Z , Hu R , Cao G , Liu Q 2010: Initial responses of soil CO2 efflux and C, N pools to experimental warming in two contrasting forest ecosystems, Eastern Tibetan Plateau, China. Plant Soil ., 336, 183–195. doi:10.1007/s11104-010-0461-8
- Yan JH , Wang YP , Zhou GY , Zhang DQ 2006: Estimates of soil respiration and net primary production of three forests at different succession stages in South China. Glob. Change Biol ., 12, 810–821. doi:10.1111/j.1365-2486.2006.01141.x
- Yan M , Zhang X , Jiang Y , Zhou G 2011: Effects of irrigation and plowing on soil carbon dioxide efflux in a poplar plantation chronosequence in northwest China. Soil Sci. Plant Nutr ., 57, 466–474. doi:10.1080/00380768.2011.590943
- Yang CM , Yang LZ , Ouyang ZO 2005: Organic carbon and its fractions in paddy soil as affected by different nutrient and water regimes. Geoderma , 124, 133–142. doi:10.1016/j.geoderma.2004.04.008
- Yuste JC , Janssens IA , Carrara A , Ceulemans R 2004: Annual Q (10) of soil respiration reflects plant phenological patterns as well as temperature sensitivity. Glob. Change Biol ., 10, 161–169. doi:10.1111/j.1529-8817.2003.00727.x