Abstract
Radioactive 137Cs concentrations of forage corn (Zea mays L.) and Italian ryegrass (Lolium multiflorum Lam.) in a double cropping system under continuous cattle farmyard manure (FYM) application were observed for more than 2 years after the Fukushima Daiichi Nuclear Power Station accident in 2011. The experiment field is located 110 km southwest of the Fukushima Daiichi Nuclear Power Station, and the soil contains 137Cs of 920 Bq kg−1 on average. For crop cultivation, nitrogen fertilizer was applied in addition to FYM. The 137Cs concentrations in corn decreased significantly between 2011 and 2012, but only differed significantly between 2012 and 2013 for the plot with no FYM application. For Italian ryegrass, no significant differences were observed between the harvest in 2012 and 2013 despite the FYM application rate. To minimize corn 137Cs concentrations, the FYM application rate should be more than and equal to 30 Mg ha−1 when FYM is used as the major nutrient source. Exchangeable potassium oxide (K2O) greater than around 0.3 g kg−1 was mostly maintained with the FYM application rates. Corn 137Cs concentration appeared to increase at exchangeable K2O levels below 0.15 g kg−1. These results suggest that continuous FYM application can maintain soil nutrients including K2O and thereby control radioactive Cs transfer from the soil. FYM application rate of 30 Mg ha−1 is within the levels recommended by the prefectural governments around Fukushima Prefecture for crop production before the accident. These levels are sufficient to decrease the radioactive Cs concentrations for corn. However, unlike corn, differences in soil chemical properties by FYM application did not affect 137Cs concentrations in Italian ryegrass in this study, although low exchangeable K2O seemed to increase concentrations of stable 133Cs. Further experiments should be conducted to understand the observed differences between corn and Italian ryegrass.
1. INTRODUCTION
Cesium-137 (137Cs) and cesium-134 (134Cs) are important radioactive nuclides because of their longer radiological half-lives and high fission yield from uranium-235 and plutonium-239. After the Fukushima Daiichi Nuclear Power Station accident in 2011, the development of countermeasures against food contamination by radioactive Cs became a pressing issue in northeastern Japan. Reducing the radioactive Cs concentration in forage crops helps prevent radioactive Cs contamination in animal products, thereby helping to restart livestock farming in the contaminated area.
Surveys of 137Cs concentrations in forage crops were carried out by prefectural governments, and the results were published by the Ministry of Agriculture and Forestry and Fisheries of Japan (MAFF Citation2013). According to the surveys, after the 2011 summer, annual forage crops such as forage corn (Zea mays L.), sorghum [Sorghum bicolor (L.) Moench] and Italian ryegrass (Lolium multiflorum Lam.) had lower radioactive Cs concentrations than the provisional tolerance level for cattle roughage (MAFF Citation2012). Forage corn and annual grasses are therefore favorable forage crops in the contaminated area. It is important to understand why these annual crops have lower radioactive Cs concentrations.
In Japan, it has been reported that there are differences in the nutritional management of annual crops and some perennial pastures used for grazing (Inoue et al. Citation1999; Kanno et al. Citation2003; Hatanaka et al. Citation2004; Ohtsu et al. Citation2010). In general, annual crops are cultivated intensively with high soil nutrient levels as a result of continuous application of farmyard manure (FYM). Continuous FYM application increases or changes soil chemical contents and properties such as exchangeable potassium (K), total nitrogen (T-N), total carbon (T-C) and soil pH, which tend to influence the transfer of Cs from soil to crops.
Increased soil K reduces the radioactive Cs concentration in plants by preventing Cs uptake by the roots (Bange and Overstreet Citation1960; Tensho et al. Citation1961; Alexakhin Citation1993; Nisbet et al. Citation1993). This is because K strongly competes with Cs for uptake from the soil solution (Shaw and Bell Citation1989; Smolders et al. Citation1997; Zhu Citation2001). Increased soil T-N increases the ammonium ion (NH4+) supply, which has the undesirable effect of enhancing the mobility of radioactive Cs in the soil (Sanchez et al. Citation1999; Takeda et al. Citation2008), although NH4+ is known to compete with Cs for uptake by wheat (Triticum aestivum L.) roots in water culture (Shaw and Bell Citation1991). High levels of soil T-C increased the mobility of 137Cs in a wide range of soils from mineral to organic soils (Van Bergeijk et al. Citation1992; Rigol et al. Citation2002), resulting in a high soil-to-plant transfer factor (TF) in organic soil (Sanzharova et al. Citation2009). It is also known that acidic soil with a pH below 4.8 increases the transfer of radioactive Cs to grasses (Frissel et al. Citation2002). Therefore, the effect of FYM application on the transfer of radioactive Cs occurs through the combined interactions of changes in the soil chemical properties.
Application of organic materials such as FYM and compost has decreased crop radioactive Cs concentrations in cultivation experiments (Nishita et al. Citation1973; Tsumura et al. Citation1984; Lembrechts Citation1993). However, there are few reports regarding the optimal FYM application level to reduce radioactive Cs. This information is required for agricultural practices to control radioactive Cs contamination and simultaneously maintain proper nutrient levels, soil physical properties and crop productivity.
As well as the soil chemical properties, the time-dependent fixation of radioactive Cs by adsorption on soil clay minerals is an important factor in determining plant radioactive Cs concentrations (Takeda et al. Citation2013). In Ukraine, the crop TF for 137Cs has decreased exponentially over time since the Chernobyl nuclear accident in 1986 (Prister et al. Citation2003), with the rate comprising both fast and slow components. However, it should be clarified whether the Cs concentration in crops grown under field conditions changed after the accident. It is a particular concern that these changes might be affected by changes in the soil chemical properties resulting from FYM application.
In this study, we measured the 137Cs concentration in forage corn and Italian ryegrass cultivated in a double cropping system under continuous FYM application for more than 2 years after the 2011 Fukushima accident. We found that FYM application increased soil nutrient contents and decreased the 137Cs concentration in forage corn. Nutritional management using FYM as a major nutrient supply is discussed for crop cultivation in the contaminated area.
2. MATERIALS AND METHODS
2.1 Site description
From 2011 to 2013, a field study was carried out at the NARO Institute of Livestock and Grassland Science (NILGS), Nasu Research Station, Japan (36°55′N, 139°55′E), to observe 137Cs concentrations in a forage corn–Italian ryegrass double cropping system under different FYM application rates after the Fukushima Daiichi Nuclear Power Station accident in 2011. The experiment field is located 110 km southwest of the Fukushima Daiichi Nuclear Power Station. After the accident, the 137Cs concentration in the plowed soil was about 800 Bq kg−1. The soil was classified as a loamy soil over a fragmental, mixed, mesic Entic Haplumbrept (Soil Survey Staff Citation1999) by Kurashima et al. (Citation1993). The topsoil contained volcanic ash.
The nearest fallout measurements were recorded at Utsunomiya, which is 36 km south of NILGS (Tochigi Prefecture Citation2014). The deposition density of 137Cs fallout at Utsunomiya is less than 10 kBq m−2 as predicted from the radioactive Cs distribution map by aerial monitoring (MEXT Citation2011; JAEA Citation2013), while the experimental field is estimated to be about 200 kBq m−2 by soil data in this study. The cumulative 137Cs fallout during crop cultivation totaled 131, 12 and 6 Bq m−2 for corn in 2011, 2012 and 2013, respectively, and 100 and 67 Bq m–2 for Italian ryegrass in 2012 and 2013.
2.2 Experiment design for monitoring 137Cs concentrations
The field had been used for double cropping with continuous FYM application since 2006. Forage corn and Italian ryegrass were cultivated as summer and winter crops in turn in the same field. We took the opportunity to examine the effect of continuous FYM application on radioactive Cs transfer.
The applied FYM was obtained from dairy cattle excrement and sawdust at the Shiobara FYM center located near NILGS. On average, this material contained 600 g kg−1 water, 16 g kg−1 N, 9.4 g kg−1 phosphorus pentoxide (P2O5), and 30 g kg−1 potassium oxide (K2O), with 150 Bq kg−1 137Cs and a C:N ratio of 27. [Note that K and phosphorus (P) in soil and FYM are expressed as K2O and P2O5 values throughout this article.] The FYM application rates were 0, 15, 30 and 45 Mg ha−1 on a fresh-matter (FM) basis.
The plot area for each application rate was 380 m2. There was no replication for the FYM treatment. This experimental design made it difficult to consider the differences between the plots in terms of the FYM treatment. Therefore, the yearly changes in crop and soil data within the same plot were the basis of the experimental results and are mainly discussed. To statistically compare the effects of FYM application, the average results for plots using FYM application rates of 0 and 15 Mg ha–1 were compared with the average results for plots using FYM at 30 and 40 Mg ha–1. Statistical differences in the soil chemical contents between the plots are outlined in .
2.3 Forage corn and Italian ryegrass cultivation
After FYM application, the field was plowed using a moldboard plow at a depth of 23 cm, followed by rotary tillage before crop seeding. Seeds of forage corn (Zea mays L. cv Cecilia) were sown 0.2 m apart in rows spaced 0.75 m apart. Then, ammonium sulfate was immediately applied at a rate of 100 kg N ha−1 to the soil surface. No other fertilizer was applied. The field was then compacted using a land roller before herbicide treatment with alachlor and atrazine at standard recommended concentrations. The forage corn plants were harvested at the yellow-ripe stage by cutting the plants at 10 cm above ground level. Four samples of 10 plants each were collected in 2011 and 2013 and six samples of 10 plants each were collected in 2012 from each FYM plot. Sampled plants were weighed to determine the fresh weight. The respective sowing and harvesting dates were May 31 and September 14 for the 2011 harvest, May 31 and September 12 for the 2012 harvest, and May 27 and September 3 for the 2013 harvest.
For Italian ryegrass (Lolium multiflorum Lam. cv. Nioudachi) cultivation, soil was plowed and tilled using the same method described above for corn after FYM application. Then urea was spread at 120 kg N ha−1 and seeds at 40 kg ha−1, and the soil surface was stirred by tooth harrow to mix them into the soil. The field was compacted using a land roller. Italian ryegrass was harvested by cutting the plants at 10 cm above ground level. Six samples of 1 m2 were collected in 2012 and four samples of 2 m2 were collected in 2013 from each FYM plot and weighed to determine the fresh weight. The respective sowing and harvesting dates were October 11, 2011 and May 8, 2012 for the 2012 harvest, and October 4, 2012 and May 2, 2013 for the 2013 harvest.
The sampled plants were cut into pieces measuring 2–3 cm long using a chopper, and then the pieces were mixed well to produce a composite sample. Between 2 and 4 kg of the mixed material from each sample was packed in a paper bag and dried in an oven at 90°C for 3 d to determine the water content. The dried plant material was then powdered using a Willey mill with a 2-mm screen. The water content and total harvest biomass were used to estimate the dry matter (DM) yield.
The cumulative effective air temperatures above 0°C during corn cultivation were 2436°C in 2011, 2384°C in 2012, and 2280°C in 2013. The lower temperature in 2013 might have resulted in a lower DM yield than those in the other years. During Italian ryegrass cultivation, the cumulative effective air temperatures were 1307°C in 2012 and 1333°C in 2013. The cumulative precipitation totaled 831 mm in 2011, 614 mm in 2012 and 613 mm in 2013 for corn, and 679 mm in 2012 and 663 mm in 2013 for Italian ryegrass. These temperature and precipitation values were within the normal range of variation for the study site.
2.4 Chemical analysis of soil, plants and FYM
A bulk soil sample of about 8 kg was collected from the top 23 cm of soil from the middle of each plant sampling site. Soil samples were collected within 1 week after the crop harvest. Before soil analysis, samples were air-dried and sieved through a 2-mm screen. Soil pH was measured in water (1:2.5 weight/weight) by using a pH glass electrode. Available P was extracted using the method of Truog (Citation1930), and the P content (as P2O5) was determined by spectrophotometry using an autoanalyzer (QuAAtro, BLTEC, Osaka, Japan). T-C and T-N contents were determined by the dry combustion method by using a CN analyzer (JM1000CN; J-Science, Kyoto, Japan). Cation exchange capacity (CEC) was determined using the method of Schollenberger and Simon (Citation1945). Exchangeable cations extracted by ammonium acetate solution (1 M) were measured by atomic absorption spectroscopy (SpectroAA 220, Varian, Tokyo, Japan).
Exchangeable soil 137Cs was extracted using 20 times the soil volume of ammonium acetate solution (1 M, pH 7.0) with shaking for 1 h at room temperature. The mixture was filtered (filter paper 5C, Advantec, Tokyo, Japan) and the extract was centrifuged at 12,000 g for 20 min. The supernatant was filtered through a membrane filter (0.45-µm pore size, nitrocellulose). Both soil total 137Cs before extraction and the extracted 137Cs in extraction were analyzed by gamma spectrometry. The extraction rate was calculated by dividing the extracted 137Cs by the total soil 137Cs on the same weight basis. This experiment was conducted for the soil samples collected from the plot treated with 30 Mg FYM ha−1.
FYM samples were air-dried and then milled in a vibrating sample mill (TI-100, CMT, Iwaki, Japan) to give a fine powder (< 0.1 mm). The mineral contents of plants and FYM samples were analyzed by atomic absorption spectroscopy or spectrophotometry after wet digestion with nitric acid and perchloric acid. T-C and T-N in the FYM were determined as described in soil analysis.
Analyses for 133Cs stable isotope concentrations in plant samples were conducted using inductively coupled plasma mass spectrometry (7700x, Agilent, Tokyo, Japan) after wet digestion according to the method of Harada and Hatanaka (Citation2000).
2.5 137Cs measurements
All plant, FYM and soil samples and extracts were analyzed for 137Cs by high-resolution gamma spectrometry with a germanium detector (GEM20P4-70; resolution 1.8 keV at 1.33 MeV, relative efficiency 20%; ORTEC, Oak Ridge, TN, USA) and a multi-channel analyzer (MCA7600, Seiko EG&G, Tokyo, Japan). In this study, 134Cs concentrations of some samples in 2013 were too low to detect due to physical decay, so 134Cs is not suited for observing yearly TF changes, and thus only the results for 137Cs are discussed here. The 137Cs concentrations were calculated on a DM basis. The counting time was 3600 s for each powdered plant sample of about 0.6–1 kg packed in a 2-L Marinelli vessel, 3000 s for each FYM sample of about 0.5 kg packed in a 1-L Marinelli vessel and 2000 s for each soil sample of about 70 g packed in a 100-mL U-8 vessel. Two liters of soil extract with 1 M ammonium acetate was analyzed for 10,000 s in a 2-L Marinelli vessel. Measurement results for plant samples collected in 2012 and 2013 were about 4 times higher than the detection limit, on average. The average detection limits for 137Cs were about 2, 2, 12 and 0.1 Bq kg−1 for powdered plant material, FYM, soil and extract solution samples, respectively. The correction date used to account for the radioactive decay of 137Cs, with a half-life of 30.1 years, in the soil and plants was October 1 for corn and June 1 for Italian ryegrass in each year.
2.6 Statistical analysis
Differences in mean values were assessed using the Games–Howell test or the Welch’s t-test for two-pair comparison for soil and crop 137Cs concentrations and soil chemical properties due to significant differences in the calculated variances. For other analyses with equal variances, the Tukey–Kramer test for multiple comparisons was used. Linear correlation was analyzed using the Pearson product-moment correlation coefficient. The R statistical software package version 2.11.1 (R Development Core Team Citation2010) was used for the statistical analyses. Regression analysis was also performed using R.
3. RESULTS
3.1 Yearly changes in crop and soil 137Cs concentrations
For corn, 137Cs concentrations decreased significantly from 2011 to 2012 for all plots, whereas the concentrations in 2012 and 2013 did not differ significantly except for the plot without FYM application (). With no FYM application, corn 137Cs concentration increased significantly from 2012 to 2013. For Italian ryegrass, no significant differences were observed in the 137Cs concentrations between the 2012 and 2013 harvests for all plots. Corn 137Cs concentrations were only 40% of the corresponding values for Italian ryegrass in the plot treated with 30 Mg FYM ha−1 in 2012 and 2013.
Figure 1 Plant 137Cs concentration in corn (Zea mays L.) (left) and Italian ryegrass (Lolium multiflorum Lam.) (right) cultivated in a double cropping system for plots with different farmyard manure (FYM) application rates. Bars labeled with different letters are significantly different (Games–Howell test, P < 0.05) within each plot with different FYM application rates. No significant differences were observed for Italian ryegrass (Welch’s t-test, P > 0.05). Error bars indicate standard deviations.
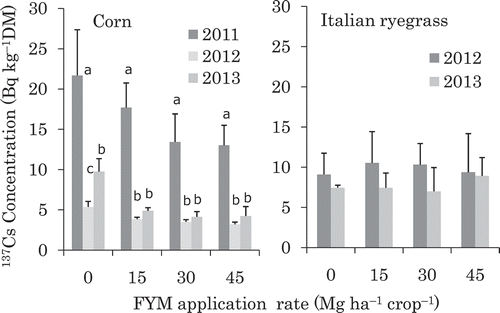
In accordance with the change in crop 137Cs concentration, the ammonium acetate-extractable soil 137Cs decreased significantly in the soil of the plot treated with 30 Mg FYM ha−1 after the 2011 corn harvest, while it did not change significantly in subsequent samples (). No significant differences in soil 137Cs concentration among the plots and sampling date were observed ().
Figure 2 Changes in ammonium acetate-extractable soil 137Cs in the plot treated with 30 Mg FYM ha−1. The two October samples were collected after the corn (Zea mays L.) harvest, and the two May samples were collected after the Italian ryegrass (Lolium multiflorum Lam.) harvest. Bars labeled with different letters are significantly different (Tukey–Kramer test, P < 0.05). Error bars indicate standard deviations.
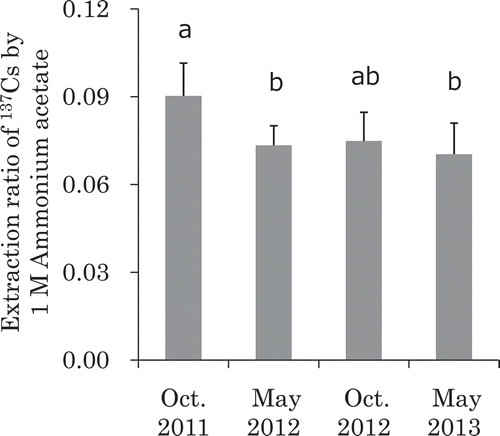
3.2 Soil properties in FYM application plots
summarizes the soil chemical properties for all plots, and values represent the average for the 3 years of the study. Higher soil pH was observed in the plots with higher FYM application rates; however, soil pH did not exceed 7.0. The exchangeable K, magnesium (Mg), calcium (Ca), T-N and T-C contents were also higher in the plots with higher FYM rates, which is not surprising given that FYM contains these components.
Table 1 Chemical properties of soils in manure application plots during cultivation experiment.
Soil exchangeable K2O decreased continuously during the experiment in the plot with no FYM (). We also found no significant differences in exchangeable K2O among sampling dates in the plots treated with 15 and 30 Mg FYM ha−1, and we observed unstable changes in plots treated with 45 Mg FYM ha−1.
Figure 4 Changes in soil exchangeable potassium oxide (K2O) content in plots with different farmyard manure (FYM) application rates. Different lowercase letters indicate significant differences within each plot with different FYM application rate (Tukey–Kramer test, P < 0.05). No significant differences among dates were observed for the 15 and 30 Mg ha−1 plots. Error bars indicate standard deviations.
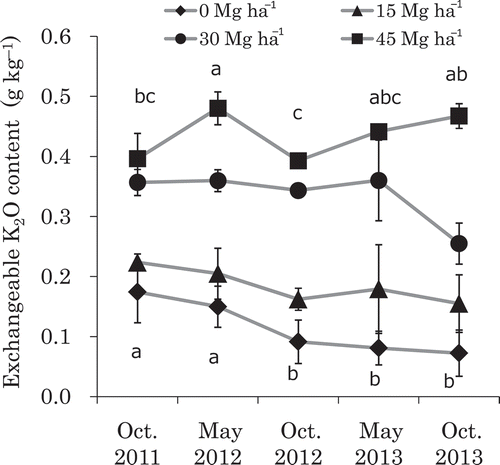
3.3 Effect of exchangeable K2O contents and DM yield on corn Cs concentrations
Radioactive 137Cs and stable isotope of 133Cs concentrations of forage corn were plotted against the exchangeable K2O content for each year (). As was expected, 137Cs and 133Cs concentrations seemed to increase under lower exchangeable K2O conditions in this study. The 133Cs concentrations seemingly increased more sharply with lower exchangeable K2O content than 137Cs. In this experiment, it should be noted that other soil chemicals were also changed, as shown in .
Figure 5 Effect of the exchangeable potassium oxide (K2O) content on plant 137Cs (Cs137) and 133Cs (Cs133) concentration in corn (Zea mays L.). Error bars indicate standard deviations.
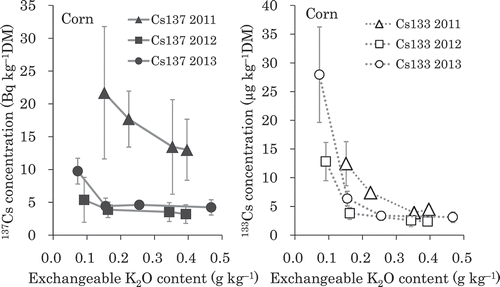
Continuous FYM application might affect crop 137Cs concentration through DM yields. The DM yields of corn and Italian ryegrass are shown in . The DM yield of corn without FYM application decreased slightly but continuously from 2011 to 2013. The DM yield in 2013 was lower than that in 2012, whereas 137Cs concentration increased from 2012 to 2013 (, ).
Figure 6 Dry matter yields in corn (Zea mays L.) and Italian ryegrass (Lolium multiflorum Lam.) cultivated in a double cropping system in fields with different FYM application rates. Bars labeled with different letters are significantly different (Tukey–Kramer test, P < 0.05) within each plot with different (FYM) farmyard manure application rates. No significant differences were observed for Italian ryegrass (Welch’s t-test, P > 0.05). Error bars indicate standard deviations.
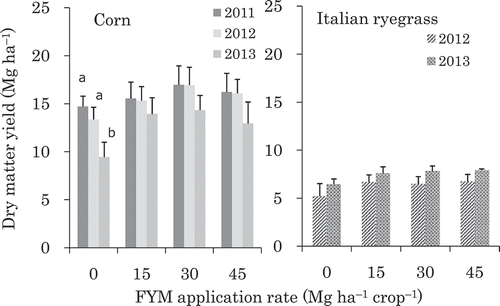
3.4 Effect of exchangeable K2O contents on Italian ryegrass Cs concentrations
Unlike the situation for corn, the exchangeable K2O content did not affect 137Cs concentrations in Italian ryegrass (). The effect of exchangeable K2O content on 133Cs concentrations also seemed to be smaller than that in corn.
Figure 7 Effect of soil exchangeable potassium oxide (K2O) content on the plant 133Cs and 137Cs concentration in Italian ryegrass (Lolium multiflorum Lam.). Error bars indicate standard deviations.
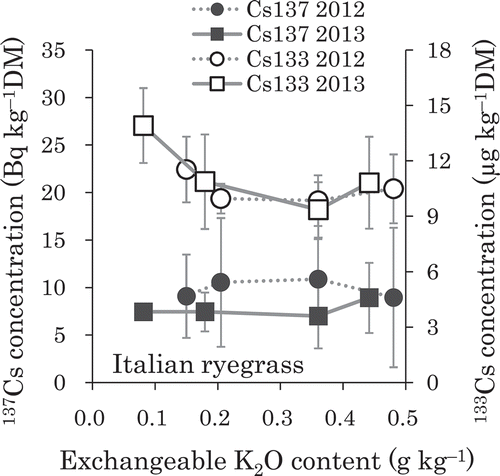
illustrates the relationship between the 133Cs and 137Cs concentrations in both crops. We observed significant correlations for corn in each year and the regression lines were almost the same in 2012 and 2013. However, we found no significant correlations for Italian ryegrass, possibly because of the narrow ranges of the measured values.
Figure 8 Relationship between the 133Cs and 137Cs concentrations for corn (Zea mays L.) and Italian ryegrass (Lolium multiflorum Lam.). Significant correlations (P < 0.05) were observed for corn in all 3 years, but no significant relationships were observed for Italian ryegrass. The lines drawn for corn in 2011, 2012 and 2013 are represented by: y = 1.02x + 9.24 (R2 = 0.968); y = 0.190x + 2.97 (R2 = 0.971); y = 0.220x + 3.49 (R2 = 0.982), respectively.
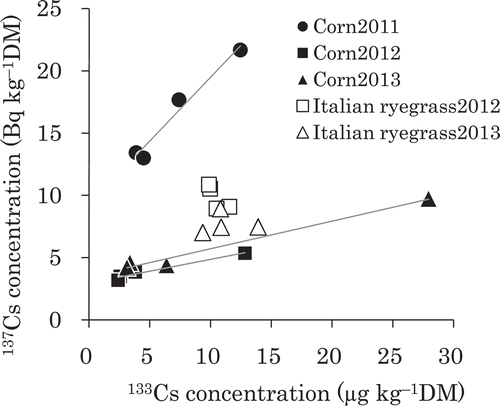
3.5 Effect of exchangeable K2O contents on crop K concentration
The exchangeable K2O content affected the crop K concentrations (). Crop K concentration increased significantly with increasing soil exchangeable K2O content for corn and for Italian ryegrass.
Figure 9 Effect of soil exchangeable potassium oxide (K2O) content on plant K concentration in corn (Zea mays L.) and Italian ryegrass (Lolium multiflorum Lam.). The relationships were well fitted to the logarithmic trend lines drawn for corn (y = 4.84ln(x) + 18.3, R2 = 0.794) and for Italian ryegrass (y = 7.99ln(x) + 41.9, R2 = 0.939).
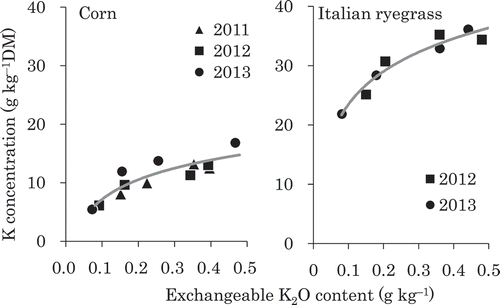
4. DISCUSSION
4.1 Monitoring crop radioactive Cs concentration after the Fukushima accident
Of the yearly changes in crop 137Cs concentration within each plot, only the corn 137Cs concentration clearly decreased from 2011 to 2012. This suggests that a change in the transferability of 137Cs from the soil to the crops might have occurred after the corn harvest in 2011 despite the FYM application rates, and the transferability might have remained roughly constant thereafter. In accordance with the crop 137Cs concentrations, the extractable soil 137Cs decreased significantly after the corn harvest in 2011 (). This accordance between crop and soil 137Cs data at the field level has not been reported after the Fukushima accident. The exchangeable K2O did not explain the 137Cs concentration changes from 2011 to 2012 ().
An aging effect in transferability for a stable isotope of Cs, 133Cs, is considered to be negligible because 133Cs is present in an equilibrium state in the soil. Using this assumption, the decrease in 137Cs transferability observed in this study can be calculated as one fifth from 2011 to 2012 based on the change in the slope of the 137Cs/133Cs regression lines drawn in . This data also indicates that the relative 137Cs transferability compared with 133Cs was not affected by the different soil chemical properties caused by continuous FYM application.
An aging effect after 133Cs addition to Japanese soils was reported by Takeda et al. (Citation2013). They showed parallel changes in the TF for orchard grass and the extractable 133Cs over 1200 d in an allophonic Andosol, and that the extractable 133Cs decreased from just after addition to 100 d later. A time-dependent first-order decrease in the TF of 137Cs due to fixation by soil was reported after the intensive global fallout (Fesenko et al. Citation2009) or the Chernobyl accident (Prister et al. Citation2003). In our monitoring studies, an aging effect is likely to explain the concentration changes observed in the experimental fields after the corn harvest in 2011.
In addition to aging in soil, the effect of 137Cs fallout should be considered. We observed the interception of radioactive Cs on crop surfaces by direct deposition was rapidly decreased in the spring of 2011 in NILGS (Sunaga et al. Citation2015). Based on the previous research, it appears that 18% of total deposition was observed in the Italian ryegrass harvest in 2011. If this rate was applied for the harvests after the summer of 2011, the 137Cs concentrations would be overly large despite using the fallout data at Utsumoniya, where the levels of environmental radioactivity were much less than those at the NILGS experimental field. In this study, the strong correlation between the 133Cs and 137Cs concentrations in corn () can be explained by the hypothesis that both isotopes mainly accumulate in shoots as a result of uptake through the roots. The higher 137Cs fallout in the 2011 summer might explain why the y-intercept of the regression line for corn in 2011 was higher than those in 2012 and 2013 (). The occurrence of fallout could not be neglected.
4.2 Effect of DM yield on crop radioactive Cs concentration
It is possible that changes in DM yields might have apparent effects on crop radioactive Cs concentrations under the condition with stable 137Cs transferability in soil in this study (). For the plot not treated with FYM, corn 137Cs concentration in 2013 was significantly 86% higher than that in 2012 (), but 137Cs transfer from soil to corn showed no significant difference between the years, being only 22% larger in 2013 (data not shown). The significant 43% decrease in DM yield in 2013 partly explains the increase in 137Cs concentration in 2013 (). For the FYM-treated plots, the average corn 137Cs concentration of the three plots in 2013 was 4.5 Bq kg−1, which was significantly 28% larger than that in 2012 (). The average DM yield of the three plots in 2012 was 16.1 Mg ha−1, which was significantly 17% larger than that in 2013, whereas the amount of 137Cs transfer from soil was not significantly different between the years (data not shown). Higher DM yield would lead to reduced 137Cs concentration under constant 137Cs transferability in soil. Therefore, proper FYM application may have a favorable effect on reducing corn 137Cs concentration though enhancing DM yield as well as changes in soil chemical properties.
4.3 Effect of continuous FYM application on corn radioactive Cs concentration
For corn, the 137Cs concentrations in the plots treated with FYM appeared lower than that without FYM (). However, statistical analysis could not examine the differences between the FYM application treatments because of the experimental design. Therefore, plot data were combined based on whether FYM application rates were >= 30 Mg FYM ha−1, or lower. We found that the average corn 137Cs concentration in 0 and 15 Mg FYM ha−1 plots was significantly higher than that from the 30 and 45 Mg FYM ha−1 plots. Thus, continuous FYM application at more than 30 Mg FYM ha−1 has an effect to reduce 137Cs concentration in forage corn.
In this experiment, the exchangeable K2O level measured after cultivation required for a decreasing corn 137Cs concentration would be around 0.3 g kg−1 (, left), because it would lie between the 15 and 30 Mg FYM ha−1 treatments, and seemed to be effective in decreasing 137Cs and 133Cs concentration in (right). This result seemingly harmonizes with the report by Frissel et al. (Citation2002), which illustrated that a higher TF was expected for Cs in cereals grown in soils with an exchangeable K concentration lower than 0.5 cmolc kg−1 (equal to 0.195 g kg−1 as K or 0.235 g kg−1 as K2O). However, it is noted that continuous FYM application increased other soil chemical contents as well as exchangeable K2O, and further experiment will be required to estimate a critical exchangeable K2O level to reduce corn 137Cs concentration safely and effectively under various cultivation conditions.
Deprivation of soil minerals including exchangeable K2O by crop harvesting will be a factor in the yearly 137Cs changes in corn after 2012. The increase in 137Cs concentration from 2012 to 2013 observed for no FYM application () may be partly explained by the slight decrease in exchangeable K2O () in addition to DM dilution effect as described in earlier. Of course, one of the reasons for lower corn DM yield was nutrient deprivation. The data confirm the importance of nutrient supply including K2O in controlling radioactive Cs concentration in corn.
4.4 Effect of continuous FYM application on Italian ryegrass radioactive Cs concentration
The effects of continuous FYM application and exchangeable K2O contents on Italian ryegrass 137Cs concentrations were not clear (, ), whereas the 133Cs concentration appeared to increase at an exchangeable K2O content of less than 0.10 g kg−1 (). A possible explanation for the weak response of Italian ryegrass is that the reducing effect by soil nutrients on Cs transfer may occur at a lower level than in corn. We do not consider that Italian ryegrass is a special annual crop showing lower radioactive Cs concentration despite the soil K2O level, because it is reported that the radioactive Cs concentration of Italian ryegrass planted in contaminated pastures after renovation was more than 500 Bq kg−1 DM under high exchangeable K2O levels, and their TFs ranged from 0.5 to 1.55 (Miyagi Prefecture Citation2013). The TFs are within the range of pasture in the parameter handbook by International Atomic Energy Agency (IAEA) (Citation2010) or comparable to those of other grasses reported by Ogura et al. (Citation2014). Further study should be performed to elucidate the effect of FYM application on radioactive Cs transfer to Italian ryegrass.
4.5 Optimal FYM application rate to control radioactive Cs uptake
To protect animal health, K concentrations in forage crops should be carefully controlled to avoid milk fever, hypocalcemia and other ailments. The recommended K concentration is 20 g kg−1 DM in roughage (National Agriculture and Food Research Organization Citation2006). The K concentration of 30 g kg−1 DM is safe for lactating cows, but not for dry cows. In general, the K requirement for animals can be satisfied even though forage crops have insufficient K for optimal growth (Kurashima and Ishii Citation1977). To date, the recommended FYM application rates for annual forage crops range from 30 to 40 Mg ha−1 per crop in the prefectural fertilization standards around Fukushima. These conventional application rates are optimized from multiple perspectives, including crop yield, mineral concentration in crops, maintaining soil fertility (including exchangeable K2O), farm management and environmental pollution.
In this study, continuous FYM application at rates of 30 Mg FYM ha−1 per crop or higher was capable of decreasing radioactive Cs uptake for corn in the double cropping system (). However, K concentration in Italian ryegrass was more than 30 g kg−1 DM at the conventional recommended FYM levels (). This means there is a trade-off relationship between radioactive Cs and K concentrations to control forage safety. Therefore, total forage K concentration should be controlled by using low-K roughage if necessary (Fukushima Prefecture Citation2013).
The K2O balance between FYM application and crop uptake is a major factor in maintaining soil K2O fertility. The K2O inputs from continuous FYM application during five successive cultivations in the four plots (0, 15, 30 and 45 Mg FYM ha−1) were calculated to be 0, 0.75, 1.50 and 2.25 Mg ha−1, respectively, and the corresponding K2O outputs by crop uptake were 0.63, 1.07, 1.32 and 1.38 Mg ha−1, respectively. Therefore, the estimated K2O balances for the same plots were −0.63, −0.32, 0.18 and 0.87 Mg ha−1, respectively. The K2O data show that an FYM application rate of 30 or 40 Mg FYM ha−1 can maintain exchangeable K2O content.
However, K2O balance analysis could not explain the significant differences in exchangeable K2O observed in the 45 Mg FYM ha−1 plot and the apparent decrease in the 30 Mg FYM ha−1 plot after corn in 2013. Long-term observation is required to show the trend in changes in exchangeable K2O.
4.6 FYM application as countermeasure against radioactive Cs contamination
According to prefectural surveys, annual forage crops harvested after the 2011 summer showed lower radioactive Cs concentrations than the provisional tolerance level for cattle roughage (100 Bq kg–1 of 134Cs + 137Cs at a water content of 0.8 kg kg−1). The present results suggest that the recommended FYM application rate for maintaining soil fertility and higher DM yield in forage crop production will simultaneously decrease the contamination of fodder by radioactive Cs. In field research before the accident, continuous FYM application by local farmers was able to maintain adequate levels of exchangeable K2O in their forage fields (Hatanaka et al. Citation2004). Recently, Harada et al. (Citation2014) reported that application of FYM that was highly contaminated by radioactive Cs from the 2011 accident increased the soil radioactive Cs concentration, but not the TF. Therefore, the conventional nutrient management practiced by farmers—that is, continuous FYM application to keep adequate nutrient levels in soil—is one reason that the radioactive Cs concentrations in annual forage crops have been much lower than the provisional tolerance level after the Fukushima Daiichi Nuclear Power Station accident.
ACKNOWLEDGMENTS
This study was supported in part by grants provided by the Ministry of Agriculture, Forestry and Fisheries of Japan. We thank Ms. H. Kohyama of NILGS for her assistance.
REFERENCES
- Alexakhin RM 1993: Countermeasures in agricultural production as an effective means of mitigating the radiological consequences of the Chernobyl accident. Sci. Total Environ., 137, 9–20. doi:10.1016/0048-9697(93)90374-F
- Bange GGJ, Overstreet R 1960: Some observations on absorption of cesium by excised barley roots. Plant Physiol., 35, 605–608. doi:10.1104/pp.35.5.605
- Fesenko SV, Sanzharova N, Tagami K 2009: Evolution of plant contamination with time. In Quantification of Radionuclide Transfer in Terrestrial and Freshwater Environments for Radiological Assessments, pp. 259–263. International Atomic Energy Agency TECDOC 1616, Vienna, Austria.
- Frissel MJ, Deb DL, Fathony M et al. 2002: Generic values for soil-to-plant transfer factors of radiocesium. J. Environ. Radioact., 58, 113–128. doi:10.1016/S0265-931X(01)00061-3
- Fukushima Prefecture 2013: The method for utilization of high potassium grasses. http://www.pref.fukushima.lg.jp/download/1/future-39kariH250422.pdf (in Japanese)
- Harada H, Amaha K, Abe Y, Kojima Y, Sunaga Y, Kawachi T 2014: Transfer factor of radioactive cesium to forage corn (Zea mays L.) from soil to which contaminated farmyard manure had been applied. Soil Sci. Plant Nutr., 60, 782–789. doi:10.1080/00380768.2014.919835
- Harada H, Hatanaka T 2000: Natural background levels of trace elements in wild plants: Variation and distribution in plant species. Soil Sci. Plant Nutr., 46, 117–125. doi:10.1080/00380768.2000.10408768
- Hatanaka T, Sunaga Y, Kawachi T, Ebato M 2004: Quality of forage crops and soil chemical properties in dairy farm in northern Tochigi. NILGS Technical Report, 4, 24–38. (in Japanese).
- IAEA (International Atomic Energy Agency) 2010: Handbook of parameter values for the prediction of radionuclide transfer in terrestrial and freshwater environments. IAEA technical reports series No.472, 194pp, IAEA, Wien.
- Inoue K, Hujiyama M, Maeda Y, Out Y, Tanaka T 1999: Changes of several physico-chemical properties of upland soils in Nagasaki prefecture. Bull. Nagasaki Agric. Forest. Exp. Stn., 22, 59–68 (in Japanese).
- JAEA (Japan Atomic Energy Agency) 2013: Extension Site of the Distribution Map for Radiation Dose. http://ramap. jmc.or.jp/map/eng/
- Kanno T, Nashiki M, Higashiyama Y, Deguchi S, Meguro R 2003: Present condition of plant vegetation and soil chemical properties of public grasslands in the north Tohoku region—Predominance of Kentucky bluegrass (Poa pratensis L.) in deteriorated pastures. Jpn. J. Grassl. Sci., 49, 336–345 (in Japanese).
- Kurashima K, Ishii K 1977: Mineral supplying power of grassland soils in Tohoku district. 1. Mineral content and composition of pasture plants. Bull. Tohoku Natl. Agric. Exp. Stn., 55, 127–154 (in Japanese).
- Kurashima K, Ota T, Kusaba T 1993: Classification and characteristics of soils at the National Grassland Research Institute. Misc. Publ. Natl. Grassl. Res. Inst., 3, 1–47 (in Japanese).
- Lembrechts J 1993: A review of literature on the effectiveness of chemical amendments in reducing the soil-to-plant transfer of radiostrontium and radiocaesium. Sci. Total Environ., 137, 81–98. doi:10.1016/0048-9697(93)90379-K
- MAFF (Ministry of Agriculture, Forestry and Fisheries) 2012: Revision of the provisional tolerance level of feeds containing radioactive cesium. http://www.maff.go.jp/j/syouan/soumu/saigai/shizai_2.html (in Japanese).
- MAFF (Ministry of Agriculture, Forestry and Fisheries) 2013: Inspection results of radioactive cesium concentration of forage crops in 2012. http://www.maff.go.jp/j/chikusan/sinko/shiryo/24result.html (in Japanese).
- MEXT (Ministry of Education, Culture, Sports, Science and Technology, Japan) 2011: Preparation of distribution map of radiation doses, etc. (Map of radioactive cesium concentration in soil, September, 2013). http://radioactivity.nsr.go.jp/en/contents/5000/4165/24/1750_083014.pdf
- Miyagi Prefecture 2013: Reducing effect by potassium fertilization on radioactive Cs transfer from soil to grasses. http://www.pref.miyagi.jp/soshiki/tikusans/fukyuu88.html (in Japanese).
- National Agriculture and Food Research Organization 2006: Japanese Feeding Standard for Dairy Cattle. Japan Livestock Industry Association, Tokyo (in Japanese).
- Nisbet AF, Konoplev AV, Shaw G, Lembrechts JF, Merckx R, Smolders E, Vandecasteele CM, Lönsjö H, Carini F, Burton O 1993: Application of fertilisers and ameliorants to reduce soil to plant transfer of radiocaesium and radiostrontium in the medium to long term — a summary. Sci. Total Environ., 137, 173–182. doi:10.1016/0048-9697(93)90386-K
- Nishita H, Haug RM, Alexander GV 1973: Influence of organic matter on the availability of certain elements to barley seedlings grown by a modified Neubauer method. Plant Soil, 39, 161–176. doi:10.1007/BF00018054
- Ogura S, Suzuki T, Saito M 2014: Distribution of radioactive cesium in soil and its uptake by herbaceous plants in temperate pastures with different management after the Fukushima Dai-Ichi Nuclear Power Station accident. Soil Sci. Plant Nutr., 60, 790–800. doi:10.1080/00380768.2014.954269
- Ohtsu Y, Fujimaya M, Nagata H, Kawaguchi T, Hirokawa J 2010: Transition of nitrate nitrogen of soil solution in domestic animal feed compost successive application under forage crop field. Bull. Nagasaki Agric. Forest. Tech. Develop. Center, 1, 55–65 (in Japanese).
- Prister BS, Perepelyatnikova LV, Ivanova TN, Vynogradskaya VD, Kalinenko LV, Grytsjuk NR, Rudenko VA, Perepelyatnikov GP, Pojarkov VA 2003: The classification of Ukrainian soil systems on the basis of transfer factors of radionuclides from soils to reference plants. In Classification of Soil Systems on the Basis of Transfer Factors of Radionuclides from Soil to Reference Plants, pp. 153–178. International Atomic Energy Agency TECDOC 1497, Vienna, Austria.
- R Development Core Team 2010: R: a language and environment for statistical computing. R Foundation for Statistical Computing, Vienna, Austria. http://www.R-project.org
- Rigol A, Vidal M, Rauret G 2002: An overview of the effect of organic matter on soil–radiocaesium interaction: implications in root uptake. J. Environ. Radioact., 58, 191–216. doi:10.1016/S0265-931X(01)00066-2
- Sanchez AL, Wright SM, Smolders E, Naylor C, Stevens PA, Kennedy VH, Dodd BA, Singleton DL, Barnett CL 1999: High plant uptake of radiocesium from organic soils due to Cs mobility and low soil K content. Environ. Sci. Technol., 33, 2752–2757. doi:10.1021/es990058h
- Sanzharova N, Shubina O, Vandenhove H, Olyslaegers G, Fesenko S, Shang ZR, Reed E, Velasco H 2009: Root uptake: temperate environment. In Quantification of Radionuclide Transfer in Terrestrial and Freshwater Environments for Radiological Assessments, pp. 139–206. International Atomic Energy Agency TECDOC 1616, Vienna, Austria.
- Schollenberger CJ, Simon RH 1945: Determination of exchange capacity and exchangeable bases in soil. Soil Sci., 59, 13–24. doi:10.1097/00010694-194501000-00004
- Shaw G, Bell JNB 1989: The kinetics of caesium absorption by roots of winter wheat and the possible consequences for the derivation of soil-to-plant transfer factors for radiocaesium. J. Environ. Radioact., 10, 213–231. doi:10.1016/0265-931X(89)90026-X
- Shaw G, Bell JNB 1991: Competitive effects of potassium and ammonium on caesium uptake kinetics in wheat. J. Environ. Radioact., 13, 283–296. doi:10.1016/0265-931X(91)90002-W
- Smolders E, Vandenbrande K, Merckx R 1997: Concentrations of 137Cs and K in soil solution predict the plant availability of 137Cs in soil. Environ. Sci. Technol., 31, 3432–3438. doi:10.1021/es970113r
- Soil Survey Staff 1999: Key to Soil Taxonomy, 8th edn. Natural Resources Conservation Service, United States Department of Agriculture, Pocahontas Press, Blacksburg.
- Sunaga Y, Harada H, Kawachi T 2015: Weathering half-life of radioactive cesium for winter rye (Secale cereale L.) and Italian ryegrass (Lolium multiflorum Lam.) directly contaminated by the 2011 Fukushima Daiichi Nuclear Power Station accident. Soil Sci. Plant Nutr., 61, 200–211. doi:10.1080/00380768.2015.1008936
- Takeda A, Tsukada H, Nakao A, Takaku Y, Hisamatsu S 2013: Time-dependent changes of phytoavailability of Cs added to allophanic Andosols in laboratory cultivations and extraction tests. J. Environ. Radioact., 122, 29–36. doi:10.1016/j.jenvrad.2013.02.005
- Takeda A, Tsukada H, Takaku Y, Akata N, Hisamatsu S 2008: Plant induced changes in concentrations of caesium, strontium and uranium in soil solution with reference to major ions and dissolved organic matter. J. Environ. Radioact., 99, 900–911. doi:10.1016/j.jenvrad.2007.11.011
- Tensho K, Yo K, Mitsui S 1961: Specific mechanisms of cesium uptake by rice plant. Jpn. J. Soil Sci. Plant Nutr., 32, 139–144 (in Japanese).
- Tochigi Prefecture 2014: Monthly measurement results for environmental radioactivity. http://www.pref.tochigi.lg.jp/kinkyu/houshasen.html#koukabutu (in Japanese).
- Truog E 1930: Determination of the readily available phosphorus of soils. J. Am. Soc. Agron., 22, 874–882.
- Tsumura A, Komamura M, Kobayashi H 1984: Behavior of radioactive Sr and Cs in soils and soil–plant systems. Bull. Natl. Inst. Agric. Sci., Ser. B, 36, 57–113 (in Japanese).
- Van Bergeijk KE, Noordijk H, Lembrechts J, Frissel MJ 1992: Influence of pH, soil type and soil organic matter content on soil-to-plant transfer of radiocesium and -strontium as analyzed by a nonparametric method. J. Environ. Radioact., 15, 265–276. doi:10.1016/0265-931X(92)90062-X
- Zhu YG 2001: Effect of external potassium (K) supply on the uptake of 137Cs by spring wheat (Triticum aestivum cv. Tonic): a large-scale hydroponic study. J. Environ. Radioact., 55, 303–314. doi:10.1016/S0265-931X(00)00202-2