Abstract
The cadmium (Cd) accumulation capacity of various vegetables and the relationship between Cd concentrations in edible parts of vegetables and those in soils were assessed by conducting field experiments at Cd-contaminated sites in northern and central Taiwan. In addition, to thoroughly assess Cd concentrations in vegetables and to understand the food safety of vegetables in Taiwan, 2257 paired vegetable and surface soil samples were collected from major vegetable production areas for Cd concentration analysis. According to the bioconcentration factors calculated, the Cd accumulation capacity varied considerably among the vegetable species tested, and the order of the five vegetables with the highest capacities is peanut (Arachis hypogaea L.) > amaranth (Amaranthus tricolor L.) > spinach (Spinacia oleraceae L.) > gynura (Gynura bicolor DC.) > okra (Hibiscus esculentus L.), whereas the order of the five vegetables with the lowest capacities is bitter gourd (Momordica charantia L.) < cucumber (Cucumis sativus L.) < asparagus bean (Vigna unguiculata (L.) Walp. ssp. sesquipedalis (L.) Verdc.) < snap bean (Phaseolus vulgaris L.) < sponge gourd (Luffa cylindrica Roem.). We derived 29 soil–plant transfer models of Cd for individual vegetable species based on available pools of the Cd, manganese (Mn), zinc (Zn), copper (Cu) and iron (Fe) concentrations; soil pH; and cation exchange capacity (CEC). According to the derived models, the available Cd, Mn and Zn concentrations, and pH, served as the main factors affecting Cd concentrations in the edible parts of vegetables, whereas the CEC and available Cu and Fe concentrations are less important factors. The data of previous studies and those of this study from major vegetable production areas, including 30 vegetable crops, were used to evaluate the safety of vegetables in Taiwan. The results indicated that the percentage of vegetables with Cd concentrations exceeding the regulatory concentration was 0.54%; therefore, the food safety concern is low. However, 9.8, 1.0, 0.9 and 0.6% of amaranth, cabbage (Brassica oleracea L. Capitata Group), Chinese cabbage (Brassica pekinensis Skeels.) and carrot (Daucus carota L.), respectively, had Cd concentrations in the edible parts exceeding the regulatory concentration. Particular attention should be paid when planning production areas for these vegetables. We recommend cultivating peanuts in fields with a soil Cd concentration < 0.33 mg kg−1. The bioconcentration factors and soil–plant transfer models derived in this study might serve as assessment tools for planning farming areas for these vegetables.
INTRODUCTION
Chaney (Citation1980) introduced the concept of the “soil–plant barrier” and classified metals into four groups. According to this concept, cadmium (Cd) is classified into group IV because it poses a risk to human and animal health at a plant tissue concentration that is not generally phytotoxic. Through industrialization, man-made pollutants, including Cd, have entered the food chain through the environment and pose a threat to human health. The most widely recognized incident of soil contamination with Cd occurred in Japan. This incident involved itai-itai disease, which was caused by Cd that had leaked into the river from the Kamioka Copper Mine (Toyama Prefectural Itai-itai Disease Museum Citation2012).
According to projects conducted by the Environment Protection Administration of Taiwan (TEPA), the background soil Cd concentrations (extracted using 0.1 M hydrochloric acid, HCl) are below 0.4 mg kg−1 in Taiwan (Chen Citation2000). Emissions from the electroplating industry largely contribute to Cd in soil (Lin et al. Citation2002), resulting in total soil Cd concentrations as high as 30 mg kg−1 (Chen et al. Citation1998; Römkens et al. Citation2009). To ensure food safety in Taiwan, the Ministry of Health and Welfare (MHW) set standards for tolerable Cd levels in rice (Oryza sativa L.), vegetables and fruit crops. In addition, the TEPA set different regulatory standards for heavy metal pollutants, including Cd, in soil for food crop farmland. However, in some instances, rice and vegetables such as garlic (Allium sativum L.) and peanuts (Arachis hypogaea L.) have Cd concentrations exceeding the regulatory level, despite the Cd concentration in food crop farmland being lower than the regulatory level.
The phytoavailability of Cd in soil is affected by many soil characteristics, such as the Cd concentration, soil pH, organic matter content, clay content, cation exchange capacity (CEC), salinity, and concentrations of zinc (Zn) and manganese (Mn; Khoshgoftar et al. Citation2004; Basta et al. Citation2005; Hattori et al. Citation2006; Römkens et al. Citation2009; Sanaeiostovar et al. Citation2011). In addition, the Cd accumulation capacity of crops is affected by their species or cultivars (Alexander et al. Citation2006; Arao et al. Citation2008; Yang et al. Citation2010, Citation2009). Yang et al. (Citation2010) examined the Cd accumulation capacities of 28 vegetable species and several cultivars of five common vegetables [cowpea (Vigna sesquipedalis Koern.), kidney pea (Phaseolus vulgaris L.), bitter gourd (Momordica charantia L.), cucumbe r (Cucumis sativus L.) and squash (Cucurbita pepo L.)] in two soil Cd concentrations, and showed that substantial genotypic variation existed among vegetable species and cultivars when subjected to Cd exposure.
The Taiwan Agricultural Research Institute (TARI) collaborated with the TEPA to conduct many field experiments during 2005–2011, and both have clarified the differences in the Cd accumulation capacity between main rice varieties and derived soil–plant transfer models that use the reactive soil Cd concentration, pH and CEC to predict the Cd concentration in brown rice (Guo et al. Citation2008, Citation2009, Citation2010; Römkens et al. Citation2009). These results are extremely useful for enhancing the safety of rice in Taiwan. Because vegetables are an indispensable part of the daily diet of the Taiwanese population, there are concerns regarding the safety of vegetables in Taiwan. However, large-scale studies of vegetables are very rare in Taiwan (Shih et al. Citation2008; Lin et al. Citation1992, Citation2012), comprising only the surveys conducted by Lin et al. (Citation1992) and Lin et al. (Citation2012) who collected vegetable samples from major arable lands in Taiwan. Lin et al. (Citation1992) reported Cd concentrations in vegetables which were sorted into groups, and reported no data for individual vegetables. By contrast, Lin et al. (Citation2012) determined the Cd concentrations in 30 vegetable crops by analyzing 1900 vegetable samples. However, the authors did not report data on some major vegetables, such as corn (Zea mays L.) and onion (Allium cepa L.).
Most studies on the Cd safety of food crops have involved conducting field surveys (Wolnik et al. Citation1985, Citation1983; Wiersma et al. Citation1986; Yang et al. Citation2007) or hydroponic or pot culture experiments in soils spiked with different Cd concentrations (Alexander et al. Citation2006; Yang et al. Citation2010; Lai and Chen Citation2013). Field surveys can accurately reflect the food safety of the surveyed region, whereas culture experiments can reflect the Cd accumulation capacity of different crops. However, because of the limitations of soil conditions in the field, conducting a field survey that entails collecting samples with various Cd concentrations is challenging. Furthermore, because of the limited space for root expansion, pot experiments usually cannot completely represent field conditions.
In this study, we conducted field experiments at four Cd-contaminated sites in northern and central Taiwan with soil Cd concentrations close to or above the regulatory limit for food crop farmland. For Cd concentration and soil property analysis, vegetable and corresponding surface soil samples were collected at the time of harvest, and another 2257 paired vegetable and surface soil samples were collected from major vegetable production areas of Taiwan. Cd concentrations of soil and vegetable samples and also soil properties collected from both surveys (including data collected in this study and those collected by Lin et al. Citation2012) and field experiments were used to (1) assess the Cd accumulation capacity of various vegetable species; (2) derive soil–plant transfer models for elucidating the relationship between Cd concentrations in different vegetables and arable soils; and (3) evaluate the safety of vegetables in Taiwan.
MATERIALS AND METHODS
Field experiments
Field experiments were conducted from September 2010 to August 2013 at sites located in Bade District, Taoyuan County (Typic Paleustalfs, Oxisol); Dajia District, Taichung City (Typic Udorthents, Inceptisol); Houli District, Taichung City (Typic Hapludox, Oxisol); and Huwei Township, Yunlin County (Typic Fluvaquents, Inceptisol) (). The field size was 0.11 ha, 0.28 ha, 0.20 ha and 0.13 ha for the Bade, Dajia, Houli and Huwei sites, respectively. The soil properties and Cd concentrations of the experimental sites are shown in . Soils from these sites were polluted by irrigation water, which was polluted by the illegal discharge of contaminated water from electroplating, smelting or pigment industries; therefore, the soil Cd concentrations at each site were not uniform (). Soil Cd concentrations at the Bade site varied from 1.90 mg kg−1 to 19.4 mg kg−1, and a part of this site had a Cd concentration that exceeded the control value (5 mg kg−1) for food crop farmland. To increase variability in soil pH, this site was divided into three blocks in September 2011 and amended with 0, 7.7 and 14.8 Mt ha−1 lime to adjust soil pH to 5.02, 6.60 and 7.26, respectively. The average soil Cd concentrations of Houli and Dajia site were 3.16 mg kg−1 and 1.63 mg kg−1, respectively, and parts of these two sites had Cd concentrations exceeding the monitoring value (2.5 mg kg−1). Though the average soil Cd concentration of the Huwei site (0.66 mg kg−1) was lower than the monitoring value of Cd for food crop farmland, edible parts of peanuts with Cd concentrations over tolerance levels still were produced there in the past.
Table 1 Selected soil properties and soil cadmium (Cd) concentrations at experimental sites
Figure 1 Map of Taiwan and locations of municipalities where vegetable fields are located. (BD: Bade District, Taoyuan County; DJ: Dajia District, Taichung City; HL: Houli District, Taichung City; HW: Huwei Township, Yunlin County).
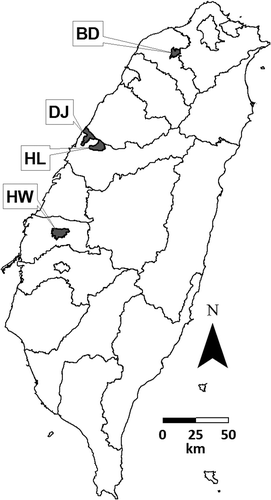
Figure 2 Distribution of cadmium (Cd) concentrations at experimental sites. (a) Bade site; (b) Dajia site; (c) Huwei site; (d) Houli site. Both the Bade and Houli sites were composed of two adjacent fields.
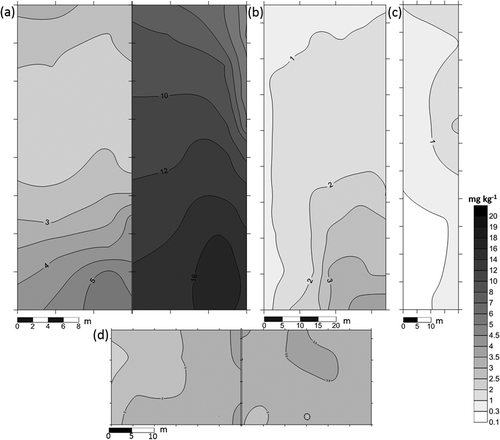
We grew 29 vegetable species at these sites. The plot sizes in the fields were 3 m × 2 m for sponge gourd (Luffa cylindrica Roem.), cucumber, tomato (Lycopersicon esculeutum Mill.), corn, snap bean (Phaseolus vulgaris L.) and asparagus bean (Vigna unguiculata (L.) Walp. ssp. sesquipedalis (L.) Verdc.), and 2 m × 1 m for other vegetables. No replicates were set because the distribution of Cd in the fields was not uniform. Besides, our aim is to relate the Cd concentrations in edible parts of vegetables to Cd concentrations in soils and soil properties; therefore, to acquire vegetable samples with various Cd concentrations, all vegetables were cultivated in a field in a specific direction along a soil Cd gradient. Cabbage (Brassica oleracea L. Capitata Group), Chinese cabbage (Brassica pekinensis Skeels.), cauliflower (Brassica oleracea L. convar. Botrytis), broccoli (Brassica oleracea L. Italica Group), and sweet potato leaves (Ipomoea batatas (L.) Lam.) were transplanted, whereas the other vegetables were planted through sowing. All vegetables were planted in suitable growing seasons and were cultivated and fertilized using conventional methods practiced by farmers in Taiwan.
Survey sites and vegetable selection
To determine Cd concentration in vegetables, and vegetable food safety in Taiwan, 29 vegetable crops including 2257 samples were collected from major vegetable production areas from 2011 to 2013. Vegetable crops were selected according to their importance among consumers based on the sales volume of vegetables (Council of Agriculture Citation2006). In addition, for validating the soil–plant transfer models derived in this study, 699 vegetable samples were collected from vegetable production areas and some home gardens in 2014. After each vegetable sample was collected, a corresponding surface soil sample was also collected from the immediate vicinity of the roots of the vegetables. To simplify the description, the field survey conducted from 2011 to 2013 is denoted as the “general survey,” whereas that conducted in 2014 is denoted as the “validation survey” in the following sections.
Sampling
Vegetable samples from both the field experiments and surveys, and the corresponding surface soil (0–15 cm) samples, were collected at the time of harvest. Vegetable and soil samples from the field experiments were collected from each plot, and those from the field surveys were collected from farmlands in major vegetable production areas and some home gardens of Taiwan. Only the edible parts of vegetables were collected. The collected quantities were sufficient to provide a representative sample of each vegetable type—for example, 10 carrots (Daucus carota L.), five radishes (Raphanus sativus L.), five ears of field corn, five onions, 1 kg of leaf vegetables and five heads of head vegetables. Soil samples were taken at a 0–15-cm depth in the immediate vicinity of the roots of vegetable samples. Both vegetable and soil samples were placed in clean plastic bags and immediately transported to the laboratory for sample treatment. Vegetable samples were shipped and refrigerated with Blue Ice to prevent decomposition.
Sample preparation
The vegetable samples were washed thoroughly with tap water, rinsed with de-ionized water, surface-dried using paper towels, cleared of nonedible portions and weighed. Potato (Solanum tuberosum L.), onion, carrot, taro (Colocasia esculenta (L.) Schott), and radish samples were peeled using a ceramic peeler before weighing. After washing and weighing, all samples were cut into small pieces using a ceramic knife, and oven-dried at 70°C. Dried samples were weighed, ground to fine powder using pure titanium knives in standard grinders, and stored in plastic bottles for subsequent analysis. The soil samples were air-dried, mechanically ground using a hardwood roller, and passed through a 2-mm sieve for determining pH, the CEC and available pools of metals. A 20–30-g subsample was drawn from the bulk soil (< 2-mm fraction), reground and passed through a 0.5-mm sieve. This fine material was used to determine the organic carbon and total Cd concentrations.
Chemical analyses
To determine Cd concentrations in vegetable samples, each sample (0.25 g) was digested with 5.5 mL of nitric acid (HNO3):hydrogen peroxide (H2O2) [10:1, volume/volume (v/v)] in a microwave oven (MARS 5; CEM, Mathews, NC, USA) as follows. Step 1: maximum power, 1600 W; ramp, 10 min; temperature, 180°C; hold, 5 min; step 2: maximum power 1600W; ramp, 5 min; temperature, 200°C; hold, 10 min. Cd concentrations in the digested samples were determined using inductively coupled plasma mass spectrometry (ICP-MS; Agilent 7500C, Santa Clara, CA, USA). To determine total Cd concentration in soil samples, each sample (0.5 g) was digested with 8 mL of HCl:HNO3 (3:1, v/v) in a microwave oven (MARS 5; CEM, Mathews, NC, USA) as follows: maximum power, 1600W; ramp, 20 min; temperature, 180°C; hold, 20 min. Cd concentrations in the digested samples were determined through inductively coupled plasma optical emission spectrometry (ICP-OES; Ultima 2C, Horiba Jobin Yvon, Irvine, CA, USA). Available pools of metals were determined using ICP-OES after extracting with 0.1 M HCl (Nelson et al. Citation1959). Soil pH was measured at a soil-to-water ratio of 1:1 (McLean Citation1982). Soil organic matter content was measured using an elemental analyzer (Vario MAX C Macro, Elementar, Hanau, Germany). The CEC was measured using 1 M ammonium acetate (NH4OAc) buffered at pH 7.0 (USDA Citation1996).
Analytical quality
For quality control and to monitor instrument variability, three certified reference soil samples and two reference plant materials were digested in the same manner as the collected samples. The two certified reference plant materials, spinach (Spinacia oleraceae L.) leaves (NIST 1570a) and tomato leaves (NIST 1573a), were uncontaminated and contained low Cd concentrations (2.89 and 1.52 mg kg−1 dry weight, respectively). Acid digestion results indicated that 95% and 89% of the Cd were recovered from the certified reference plant materials. The reference soils included CRM 023 with a low Cd concentration (0.92 mg kg−1), and CRM 026 and 032 with high Cd concentrations (11.7 and 130 mg kg−1, respectively). Acid digestion results indicated that 101, 97 and 95% of the Cd were recovered from CRM 023, 026 and 032, respectively.
Bioconcentration factor, soil–plant transfer model and statistical analyses
The bioconcentration factor (BCF) of Cd was calculated using the following equation:
where Cdveg represents Cd concentrations (fresh weight) in the edible parts of vegetables, and Cdsoil represents the total Cd concentration (dry weight) in the soil where the vegetables were grown.
The soil–plant transfer models developed by Rodrigues et al. (Citation2012) were used:
where log(Cd)veg represents log10 values of Cd concentrations in vegetables, log(Cd)soil represents log10 values of Cd concentrations in soil, log(soil_properties) represents log10 values of soil properties (except pH) and a, b…f, and n represent coefficients.
Instead of the total metal concentration used by Rodrigues et al. (Citation2012), we used a 0.1 M HCl-extractable concentration of metals. The coefficients were determined through stepwise multiple linear regression analysis with forward selection of variables. In each step, Microsoft Office Excel 2007 for Windows was used for performing linear regression, and the variables of models were selected manually according to the coefficient of determination (R2). The analysis was terminated when the increment of R2 was lower than 0.01 or when three variables had been added to the soil–plant transfer model. The variables used for the analysis were the pH, CEC and 0.1 M HCl-extractable iron (Fe), Mn, copper (Cu) and Zn concentrations.
RESULTS
Soil properties and Cd concentrations
Data collected from the general survey and field experiments and those collected by Lin et al. (Citation2012) were used in this study. An overview of the pH, CEC, total Cd and available metal concentrations in soil for each vegetable studied is provided in . The data range of all samples was 3.27–8.89 for pH; 0.22–28.8 cmol kg−1 for CEC; 0.01–19.4 mg kg−1 for the total Cd concentration; and 0.01–14.8 mg kg−1, 0.01–2,031 mg kg−1, 0.18–462 mg kg−1, 0.01–237 mg kg−1 and 0.01–266 mg kg−1 for 0.1 M HCl-extractable Cd, Fe, Mn, Cu and Zn concentrations, respectively (). All aforementioned soil properties showed great variability and had extremely wide ranges. Regarding individual vegetables, except for potatoes, taro, onion and bitter gourd, soil properties of sites where vegetable samples were collected also showed considerable variability. Because potatoes, taro, onion and bitter gourd were not planted in the field experiments, data on these four vegetables were collected only from the general survey and by Lin et al. (Citation2012). Therefore, the Cd concentrations of soil samples collected from the vicinity of the roots of these four vegetables were much lower than those of other vegetables.
Table 2 Overview of pH, cation exchange capacity (CEC), total cadmium (Cd) and available metal concentrations in soil along with Cd concentrations, bioconcentration factor (BCF), and BCF rank of vegetables†
Cd accumulation capacity of vegetables
Data collected from the general survey and field experiments, and those collected by Lin et al. (Citation2012), were used to calculate the BCF of vegetables (). According to the BCFs calculated, considerable variation existed in the Cd accumulation capacity among the vegetables tested, and the peanut was the strongest Cd accumulator among all vegetables tested. The remaining vegetables tested are ranked according to Cd accumulation capacity as follows: amaranth (Amaranthus tricolor L.) > spinach > gynura (Gynura bicolor DC.) > okra (Hibiscus esculentus L.) > ceylon spinach (Basella rubra L.) > garland chrysanthemum (Chrysanthemum coronarium L.) > carrot > potato > lettuce (Lactuca sativa L. var. crispa) > water spinach (Ipomoea aquatica Forsk.) = taro > eggplant (Solanum melongena L.) > celery (Apium graveolens L.) > green onion (Allium fistulosum L.) > pak choi (Brassica campestris L. ssp. chinensis) > Chinese leek (Allium tuberosum Rottl. ex K. Spreng.) > garlic > Chinese cabbage > radish > tomato > sweet pepper (Capsicum annuum L.) > sweet potato leaf > broccoli > cabbage > corn > cauliflower = onion > sponge gourd > snap bean > asparagus bean > cucumber > bitter gourd. The edible part of the bitter gourd was the weakest Cd accumulator among all vegetables tested.
Soil–plant transfer models of Cd in vegetables
In this study, 29 soil–plant transfer models of Cd were derived. Data collected from the field experiments and general survey and those collected by Lin et al. (Citation2012) were used to increase the variability in soil properties. Results of stepwise regression analysis for deriving the soil–plant transfer models for vegetables are shown in . Most of the models, except those for the sponge gourd, radish, carrot and snap bean, could explain > 80% of the variability in Cd concentrations in vegetables. According to the results of stepwise regression analysis, the available soil Cd concentration was the most explanatory variable for the presence of Cd in vegetables. The other explanatory soil properties were available Mn and Zn concentrations and pH, whereas the CEC and available Fe and Cu concentrations were less explanatory variables. In addition, Cd concentrations in vegetables were positively correlated with those in soil and were negatively correlated with soil pH and Mn and Zn concentrations.
Table 3 Derived soil–plant transfer models of cadmium (Cd) concentrations in vegetables used in field experiments†
Vegetables with corresponding soil sample pairs collected from the validation survey were analyzed to validate the model performance. In total, 699 vegetable samples were collected, comprising 395 samples of leafy vegetables, 57 samples of Brassica vegetables, 169 samples of fruiting vegetables and 78 samples of legume vegetables. As shown in , although scattered data points for some vegetables were observed, the models derived could satisfactorily explain the data on Cd concentrations in most vegetables.
Cd concentration in vegetables in major vegetable production areas
Data reported by Lin et al. (Citation2012) were incorporated with those collected in the general survey to establish a database of Cd concentrations in major vegetables in Taiwan. Thirty vegetable crops were studied, accounting for more than 80% of the sales volume of vegetables in Taiwan. In total, 3737 vegetable samples were used, comprising 2257 from this study and 1480 from Lin et al. (Citation2012). Among the samples, 1531 were leafy vegetables, 613 were Brassica vegetables, 978 were root and tuber or stalk and stem vegetables, 500 were fruiting vegetables, 83 were bulb vegetables and 32 were pulses and legume vegetables.
Cd concentrations in most samples were within the regulatory concentration of Cd for vegetable and fruit crops (). Amaranth, spinach, cabbage, Chinese cabbage and carrot samples exceeded the regulatory concentration, and the percentages of each vegetable with Cd concentrations exceeding the regulatory concentration were 9.8, 1.0, 0.9 and 0.6%, respectively. Overall, the percentage of vegetables with Cd concentrations (in their edible parts) exceeding the regulatory concentration was 0.54%.
Table 4 Cadmium (Cd) concentrations in vegetables (maximum, minimum, mean and standard deviation values) collected from major production areas, and percentages of vegetables exceeding the regulatory concentration of Cd in Taiwan
Relationship between different soil Cd concentrations and the food safety of vegetables
To more thoroughly evaluate the food safety of vegetables grown in soils with Cd concentrations lower than the regulatory concentrations for food crop farmland (5 mg kg−1), data collected from field experiments at Cd-contaminated sites and those collected from the general survey and by Lin et al. (Citation2012) were integrated for assessing the probability of vegetable and fruit crops exceeding the regulatory concentrations of Cd. The percentages of vegetables with Cd concentrations in edible parts exceeding regulatory concentrations, but grown in soil with different Cd concentrations (≤ 5 mg kg−1), are shown in . The percentage of water spinach, amaranth, ceylon spinach, gynura, celery, spinach, garland chrysanthemum, green onion, lettuce, pak choi, Chinese cabbage, cabbage, broccoli, carrot, garlic, tomato, sweet pepper, eggplant, okra and peanut with Cd concentrations in edible parts exceeding the regulatory concentrations increased greatly. However, cucumber samples grown in soil with Cd concentrations of 4 mg kg−1 and radish, snap bean, asparagus bean and sponge gourd samples grown in soils with Cd concentrations of 5 mg kg−1 had Cd concentrations lower than the regulatory concentration of Cd for vegetable and fruit crops.
Table 5 The percentage distribution of vegetables with cadmium (Cd) concentrations in edible parts exceeding the regulatory concentrations of Cd for plants grown in different soil Cd
DISCUSSION
Cd accumulation capacity of different vegetables
According to the BCFs calculated, substantial differences existed in the Cd accumulation capacities of various vegetables (). According to the BCFs in , the order of the five vegetables with the highest Cd accumulation capacities is peanut > amaranth > spinach > gynura > okra, whereas the order of the five vegetables with the lowest capacities is bitter gourd < cucumber < asparagus bean < snap bean < sponge gourd. According to McLaughlin et al. (Citation2000), peanuts cultured in pots showed BCFs of 4.24 and 2.96 when soil Cd concentrations were 0.044 and 0.032 mg kg−1, respectively. Bell et al. (Citation1997) reported that peanuts grown in sandy coastal areas of Australia accumulated Cd in seeds at a concentration as high as 0.348 ± 0.068 mg kg−1, even in soil with an available Cd concentration of < 0.5 mg kg−1. According to Taiwan’s present regulatory criteria for the maximum Cd concentration in pulse and legume vegetables, i.e., 0.2 mg kg−1, and the average BCF of peanuts obtained in this study, we recommend cultivating peanuts in fields with a soil Cd concentration of < 0.33 mg kg−1.
In this study, amaranth and spinach were also major Cd accumulators. Zhang et al. (Citation2014) reported that amaranth was the greatest Cd accumulator among 12 vegetables collected from the Pearl River Delta region, South China. Because of its extraordinary capacity to accumulate Cd, amaranth is often used as material for researching Cd contamination and phytoremediation (Abe et al. Citation2008; Fan and Zhou Citation2009; Alamgir et al. Citation2011; Li et al. Citation2012). Lune and Zwart (Citation1997), Wang et al. (Citation2006) and Yang et al. (Citation2010) concluded that spinach had the greatest Cd accumulation capacity. These results are in accordance with the results obtained in this study.
Our study results showed that gynura and okra also had high Cd accumulation capacities. These two vegetables are not commonly eaten worldwide; therefore, scant research has examined them. However, the field- and market-basket study conducted by the Ministry of Agriculture Forestry and Fisheries of Japan (Citation2002) showed that approximately 25% of 136 samples of okra contained Cd concentrations > 0.05 mg kg−1 (maximum Cd concentration for fruit vegetables adopted by the Codex Alimentarius Commission). Thus, okra also has a considerably high Cd accumulation capacity.
According to the BCFs in , considerable variation in the Cd accumulation capacity of each vegetable was observed, along with a large standard deviation in the BCF. Because the phytoavailability of Cd in soil is considerably affected by soil characteristics, the Cd accumulation capacity of different vegetables may be slightly different from that reported by many researchers (Lune and Zwart Citation1997; Wang et al. Citation2006; Yang et al. Citation2009, Citation2010; Zhang et al. Citation2014). However, in general, leafy vegetables have a high Cd accumulation capacity, whereas fruiting vegetables and legume vegetables have a lower Cd accumulation capacity. In our study, the BCFs of most fruiting vegetables (except for okra and eggplant) and legume vegetables were much lower than those of other vegetables, whereas the BCFs of most leafy vegetables were high.
The effects of soil properties on Cd concentrations in vegetables
The derived soil–plant transfer models of Cd for 29 vegetables are shown in . The variables of each model equation were arranged according to the sequence in which they were input into the stepwise multiple linear regression models. According to the sequence of variables in each equation, the available soil Cd concentration was the main factor affecting Cd concentrations in the edible parts of vegetables. In most of the models, except those for the radish and sponge gourd, the available soil Cd concentration could explain > 50% of the variability in Cd concentrations in vegetables. In addition to the available soil Cd concentration, the pH and the available Mn and Zn concentrations also had profound impacts on Cd concentrations in the edible parts of vegetables. In most of the models, except those for the radish, carrot, corn, sponge gourd and snap bean, the HCl–Cd, HCl–Mn and HCl–Zn variables and soil pH could explain > 80% of the variability in Cd concentrations in vegetables.
Basta et al. (Citation2005) reviewed trace element chemistry in residual-treated soil and showed that pH is often the main chemical property governing trace element sorption, precipitation, solubility and availability. Some studies have indicated that Cd concentrations in plants decreased as the soil pH increased (McBride Citation2002; Hattori et al. Citation2006; Römkens et al. Citation2009). McBride (Citation2002) reanalyzed data from published literature and available documents on Cd uptake by lettuce, Swiss chard (Beta vulgaris ssp. cicla) and corn leaves from sewage-sludge-amended soils, and showed that an equation which included the predictive factors of soil pH and total soil Cd concentration was reasonably predictive of aboveground plant tissue Cd concentrations. In the present study, soil pH had negative impacts on Cd concentrations in the edible parts of several vegetables. For example, the BCFs of garland chrysanthemum, water spinach, amaranth, gynura and spinach grown at the Bade site after September 2011 decreased with increasing soil pH ().
Figure 4 Relationships between the bioconcentration factor (BCF) of cadmium (Cd) and soil pH for garland chrysanthemum (Chrysanthemum coronarium L.), water spinach (Ipomoea aquatica Forsk.), amaranth (Amaranthus tricolor L.), gynura (Gynura bicolor DC.), and spinach (Spinacia oleraceae L.; only the trend lines derived using linear regression are shown).
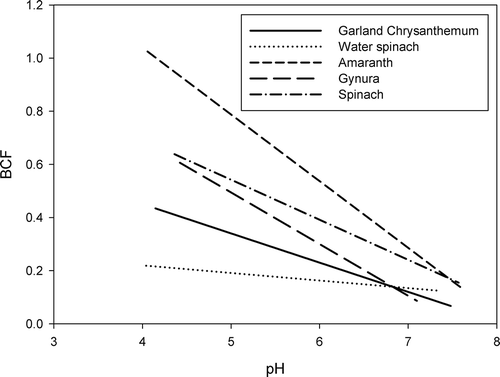
Although most of the aforementioned studies indicated that soil pH is a crucial factor affecting the Cd accumulation capacity of plants (McBride Citation2002; Basta et al. Citation2005; Hattori et al. Citation2006; Römkens et al. Citation2009; Rodrigues et al. Citation2012), in our study, soil Mn and Zn concentrations seemed to play a more crucial role. Among the 29 soil–plant transfer models derived, the HCl–Mn variable could explain more variation in Cd concentrations in vegetables than the other variables (except HCl–Cd) tested in this study did. Manganese in the soil had a negative influence and decreased the predicted concentrations of Cd in 20 vegetable species tested. Because oxides and hydroxides of Fe, aluminum (Al), and Mn provide surface sites for the chemisorption of trace elements, they are vital sinks for trace elements in soil (McBride Citation1994). Soils that are rich in manganese oxides have a high adsorption capacity for Cd (Adriano Citation1986) and hence reduce the uptake of Cd by plants. Bešter et al. (Citation2013) developed regression models for predicting the concentration of Cd in nine vegetable species grown in selected home gardens of the Municipality of Celje. They also determined that the concentration of Mn is negatively correlated with Cd in tomato and carrot. The effects of the Zn–Cd interaction on uptake and distribution in crops are relevant for plant nutrition as well as for the quality of food crops. Zn reduces the uptake and toxic effects of Cd (Khoshgoftar et al. Citation2004; Sanaeiostovar et al. Citation2011). These results are in accordance with the results obtained in this study.
According to the soil–plant transfer models of Cd derived by Römkens et al. (Citation2009), the contribution of the CEC in the HNO3 model was significant (P < 0.01) for most rice cultivars in Taiwan. Unexpectedly, the CEC was less crucial than other factors in the models derived in this study. This difference may have arisen because the models derived by Römkens et al. (Citation2009) did not include the available metal concentrations of soil as variables.
Food safety of vegetables in Taiwan
Although the field experiment results showed that Cd concentrations in some vegetables exceeded regulatory concentrations of Cd for vegetable and fruit crops even when the soil Cd concentration was lower than that stipulated for food crop farmlands (), our survey results indicated that the percentage of vegetables from main production areas with Cd concentrations exceeding the regulatory concentration of Cd was only 0.54% (), indicating that food safety concern is low. However, 9.8, 1.0, 0.9 and 0.6% of amaranth, cabbage, Chinese cabbage and carrot, respectively, had Cd concentrations in the edible parts exceeding the regulatory concentrations. In some instances, vegetables such as garlic and peanuts had Cd metal concentrations exceeding the regulatory level, despite the Cd concentration in food crop farmland being lower than the regulatory level. Therefore, particular attention should be paid when planning farming areas for these vegetables.
Because of the low dietary exposure risk of peanut, most countries—for example, the European Union, the United Nations and the USA—have not established a regulatory concentration for cadmium in peanuts. The Food Standard Australia New Zealand (FSANZ) set the maximum levels for Cd in peanut as 0.5 mg kg−1 (Standard 1.4.1), also based on the low dietary exposure risk. Similarly, though the Cd accumulation ability of peanut was very high, it accounted for only less than 0.1% of the sales volume of vegetables of Taiwan, and with very rare concern for food safety.
For other high-risk vegetables concerned, it is necessary to carefully select farmland for cultivation. From 1992 to 2008, an island-wide soil fertility survey program designed as a grid sampling with a cell resolution of 250 m × 250 m grid was conducted by TARI and seven district agricultural research and extension stations of Taiwan. In total, more than 130,000 soil samples were collected. The surveyed soil characteristics include: pH, Mehlich’s No. 3-extractable nutrients, exchangeable bases, CEC, organic matter, 0.1 M HCl-extractable heavy metals and exchangeable Al (data not shown). By using the database of the soil fertility survey, the BCFs calculated and soil–plant transfer models derived in this study therefore might serve as assessment tools for planning farming areas for vegetables to enhance the food safety of vegetables in Taiwan.
Acknowledgments
Financial support from the Environment Protection Administration, Executive Yuan, Taiwan (project names: Study on crop(rice) absorption mechanism of heavy metal and its influence on safety of agricultural products (4) [2010–2011]; Study on heavy metal absorption characteristics of vegetables and survey of emerging contaminated metals in farmland around main science parks of Taiwan [2012–2013].) and Council of Agriculture, Executive Yuan, Taiwan [grant nos. 100AS- 6.2.1-CI-C1, 101AS- 7.2.1-CI-C1 and 102AS- 7.2.1-CI-C1] is sincerely appreciated.
References
- Abe T, Fukami M, Ogasawara M 2008: Cadmium accumulation in the shoots and roots of 93 weed species. Soil Sci. Plant Nutr., 54, 566–573. doi:10.1111/j.1747-0765.2008.00288.x
- Adriano DC 1986: Trace Elements in the Terrestrial Environment. Springer-Verlag, New York, NY.
- Alamgir M, Kibria MG, Islam M 2011: Effects of farm yard manure on cadmium and lead accumulation in Amaranth (Amaranthus oleracea L.). J. Soil Sci. Environ. Manage., 2, 237–240.
- Alexander PD, Alloway BJ, Dourado AM 2006: Genotypic variations in the accumulation of Cd, Cu, Pb and Zn exhibited by six commonly grown vegetables. Environ. Pollut., 144, 736–745. doi:10.1016/j.envpol.2006.03.001
- Arao T, Takeda H, Nishihara E 2008: Reduction of cadmium translocation from roots to shoots in eggplant (Solanum melongena) by grafting onto Solanum torvum rootstock. Soil Sci. Plant Nutr., 54, 555–559. doi:10.1111/j.1747-0765.2008.00269.x
- Basta NT, Ryan JA, Chaney RL 2005: Trace element chemistry in residual-treated soils: key concepts and metal bioavailability. J. Environ. Qual., 34, 49–63.
- Bell MJ, McLaughlin MJ, Wright GC, Cruickshank A 1997: Inter- and intra-specific variation in accumulation of cadmium by peanut, soybean, and navybean. Aust. J. Agric. Res., 48, 1151–1160. doi:10.1071/A97038
- Bešter PK, Lobnik F, Eržen I, Kastelec D, Zupan M 2013: Prediction of cadmium concentration in selected home-producedvegetables. Ecotox. Environ. Safe., 96, 182–190. doi:10.1016/j.ecoenv.2013.06.011
- Chaney RL 1980: Health risks associated with toxic meatls in Municipal sluge. In Sludge–Health Risks of Land Application, Eds. Bitton G, Damro BL, Davidson GT, Davidson JM, pp. 59–83. Sci Pub, Ann Arbor, MI.
- Chen ZS 2000: Relationship between heavy metal concentrations in soils of Taiwan and uptake by crops. Technical Bulletins of Food & Fertilizer Technology Center. http://www.fftc.agnet.org/library.php (April, 2015).
- Chen ZS, Li YC, Tsai CC 1998: Investigation on background values of heavy metals in soil environment of Taiwan. Project report of Environment Protection Administration (project no.: EPA-87-H104-03-03). Environment Protection Administration, Taipei. (in Chinese with English abstract)
- Council of Agriculture 2006: Statistics of agricultural product trading volume and price. http://amis.afa.gov.tw (January, 2011, in Chinese).
- Fan H-L, Zhou W 2009: Screening of amaranth cultivars (Amaranthus mangostanus L.) for cadmium hyperaccumulation. Agric. Sci. China, 8, 342–351. doi:10.1016/S1671-2927(08)60218-7
- Guo HY, Chu CL, Chiang CF, Liu TS, Chen TK 2008: Research of crop (rice) absorption mechanism of heavy metal and the influence on safety of agricultural products (1). Project report of Environment Protection Administration. Environment Protection Administration, Taipei. (in Chinese with English abstract)
- Guo HY, Chu CL, Chiang CF, Liu TS, Chen TK 2009: Research of crop (rice) absorption mechanism of heavy metal and the influence on safety of agricultural products (2). Project report of Environment Protection Administration. Environment Protection Administration, Taipei. (in Chinese with English abstract)
- Guo HY, Chu CL, Chiang CF, Liu TS, Chen TK, Chiang CM 2010: Research of crop (rice) absorption mechanism of heavy metal and the influence on safety of agricultural products (3). Project report of Environment Protection Administration. Environment Protection Administration, Taipei. (in Chinese with English abstract)
- Hattori H, Kuniyasu K, Chiba K, Chino M 2006: Effect of chloride application and low soil pH on cadmium uptake from soil by plants. Soil Sci. Plant Nutr., 52, 89–94. doi:10.1111/j.1747-0765.2006.00007.x
- Khoshgoftar AH, Shariatmadari H, Karimian N, Kalbasi M, Zee SEATM, Parker DR 2004: Salinity and zinc application effects on phytoavailability of cadmium and zinc. Soil Sci. Soc. Am. J., 68, 1885–1889. doi:10.2136/sssaj2004.1885
- Lai H-Y, Chen B-C 2013: The dynamic growth exhibition and accumulation of cadmium of pak choi (Brassica campestris L. ssp. chinensis) grown in contaminated soils. Int. J. Environ. Res. Public Health, 10, 5284–5298. doi:10.3390/ijerph10115284
- Li N, Li Z, Fu Q, Zhuang P, Guo B 2012: Effects of chelators and small organic acids on phytoextraction of Cd from soil with Amaranth hypochondriacus L. Fresen. Environ. Bull., 21, 1879–1884.
- Lin HT, Wong SS, Li GC 1992: The concentrations of heavy metals in crop of Taiwan and the daily intake of heavy metals by R.O.C. people. J. Chin. Agric. Chem. Soc., 30, 463–470. (in Chinese with English abstract)
- Lin Y-P, Teng T-P, Chang T-K 2002: Multivariate analysis of soil heavy metal pollution and landscape pattern in Changhua county in Taiwan. Landsc. Urban Plan., 62, 19–35. doi:10.1016/S0169-2046(02)00094-4
- Lin YW, Liu TS, Chen CT et al. 2012: Contents of cadmium and lead in vegetable crops produced in Taiwan. J. Agric. Res. Taiwan, 61, 38–51. (in Chinese with English abstract)
- Lune P, Zwart KB 1997: Cadmium uptake by crops from the subsoil. Plant and Soil, 189, 231–237. doi:10.1023/A:1004270910925
- McBride MB 1994: Environmental Chemistry of Soil. Oxford University Press, New York, NY.
- McBride MB 2002: Cadmium uptake by crops estimated from soil total Cd and pH. Soil Sci., 167, 62–67. doi:10.1097/00010694-200201000-00006
- McLaughlin MJ, Bell MJ, Wright GC, Cozens GD 2000: Uptake and partitioning of cadmium by cultivars of peanut (Arachis hypogaea L.). Plant Soil, 222, 51–58. doi:10.1023/A:1004771712840
- McLean EO 1982: Soil pH and lime requirement. In Methods of Soil Analysis, Part 2, Chemical and Microbiological Properties, Eds. Page AL, Miller RH, Keeney DR, pp. 199–223. ASA and SSSA, Madison, WI.
- Ministry of Agriculture Forestry and Fisheries of Japan 2002: Survey of the cadmium contained in domestic vegetables. http://www.maff.go.jp/cd/PDF/C12.pdf (November, 2014, in Japanese).
- Nelson JL, Boawn LC, Viets FG 1959: A method for assessing Zinc status of soils using Acid-extractable Zinc and “titratable alkalinity” values. Soil Sci., 88, 275–283. doi:10.1097/00010694-195988050-00007
- Rodrigues SM, Pereira ME, Duarte AC, Römkens PFAM 2012: Soil–plant–animal transfer models to improve soil protection guidelines: a case study from Portugal. Environ. Int., 39, 27–37. doi:10.1016/j.envint.2011.09.005
- Römkens PFAM, Guo HY, Chu CL, Liu TS, Chiang CF, Koopmans GF 2009: Prediction of cadmium uptake by brown rice and derivation of soil–plant transfer models to improve soil protection guidelines. Environ. Pollut., 157, 2435–2444. doi:10.1016/j.envpol.2009.03.009
- Sanaeiostovar A, Khoshgoftarmanesh AH, Shariatmadari H 2011: Effects of zinc activity in nutrient solution on uptake, translocation, and root export of cadmium and zinc in three wheat genotypes with different zinc efficiencies. Soil Sci. Plant Nutr., 57, 681–690. doi:10.1080/00380768.2011.617290
- Shih RC, Chen WY, Kao YM, Shih YC 2008: Investigation of heavy metals in marketed vegetables in Taiwan. Annu. Report of BFDA, 26, 212–224. (in Chinese with English abstract)
- Toyama Prefectural Itai-itai Disease Museum 2012: Web site of Toyama Prefectural Itai-itai Disease Museum. http://itaiitai-dis.jp/lang/english/index.html.
- USDA 1996: Soil survey laboratory methods manual. Soil Survey Investigations, Report No. 42, pp. 622–623. USDA, Washington, DC.
- Wang G, Su M-Y, Chen Y-H, Lin F-F, Luo D, Gao S-F 2006: Transfer characteristics of cadmium and lead from soil to the edible parts of six vegetable species in southeastern China. Environ. Pollut., 144, 127–135. doi:10.1016/j.envpol.2005.12.023
- Wiersma D, Van Goor BJ, Van der Veen NG 1986: Cadmium, lead, mercury, and arsenic concentrations in crops and corresponding soils in The Netherlands. J. Agric. Food Chem., 34, 1067–1074. doi:10.1021/jf00072a033
- Wolnik KA, Fricke FL, Capar SG, Braude GL, Meyer MW, Satzger RD, Bonnin E 1983: Elements in major raw agricultural crops in the United States. 1. Cadmium and lead in lettuce, peanuts, potatoes, soybeans, sweet corn, and wheat. J. Agric. Food Chem., 31, 1240–1244. doi:10.1021/jf00120a024
- Wolnik KA, Fricke FL, Capar SG, Meyer MW, Satzger RD, Bonnin E, Gaston CM 1985: Elements in major raw agricultural crops in the United States. 3. Cadmium, lead, and eleven other elements in carrots, field corn, onions, rice, spinach, and tomatoes. J. Agric. Food Chem., 33, 807–811. doi:10.1021/jf00065a010
- Yang J, Guo H, Ma Y, Wang L, Wei D, Hua L 2010: Genotypic variations in the accumulation of Cd exhibited by different vegetables. J. Environ. Sci., 22, 1246–1252. doi:10.1016/S1001-0742(09)60245-X
- Yang Q-W, Li H, Long F-Y 2007: Heavy Metals of vegetables and soils of vegetable bases in Chongqing, southwest China. Environ. Monit. Assess., 130, 271–279. doi:10.1007/s10661-006-9395-2
- Yang Y, Zhang F-S, Li H-F, Jiang R-F 2009: Accumulation of cadmium in the edible parts of six vegetable species grown in Cd-contaminated soils. J. Environ. Manage., 90, 1117–1122. doi:10.1016/j.jenvman.2008.05.004
- Zhang H, Chen J, Zhu L, Yang G, Li D 2014: Transfer of Cadmium from soil to vegetable in the Pearl River Delta area, South China. PLoS ONE. doi:10.1371/journal.pone.0108572