Abstract
Effective soil diagnostic criteria for exchangeable potassium (Ex-K) combined with inorganic potassium (K) application rates were developed to lower K input in forage corn (Zea mays L.) production using experimental fields with different application rates and histories of cattle manure compost. Two corn varieties, ‘Cecilia’ as a low K uptake variety and ‘Yumechikara’ as a high K uptake variety, were selected from among 20 varieties and tested to make diagnostic criteria for K fertilization applicable to varieties with different K uptakes. The K uptakes increased from 96 to 303 kg K ha−1 for ‘Cecilia’ and from 123 to 411 kg K ha−1 for ‘Yumechikara’ with increasing Ex-K content on a dry soil basis from 0.11 to 0.92 g kg−1 with no inorganic K fertilizer application. The K uptake by corn for achieving the target dry matter yield of 18 Mg ha−1 was estimated to be approximately 200 kg K ha−1 in common between the two varieties. Yields of both varieties achieved the target yield at an Ex-K content of approximately 0.30 g kg−1 with no K fertilization, although ‘Yumechikara’ reached the target yield at a lower Ex-K content. At the low Ex-K content of 0.1 g kg−1, inorganic K fertilizer application at 83 kg K ha−1 was needed to gain the target yield, and apparent K recovery rate for K fertilizer was calculated to be 70% for both varieties. The K uptakes for gaining the target yield by the K fertilization were lower than that by soil K supply. Based on these results, diagnostic criteria of Ex-K and inorganic K application rates were set up as follows: at an Ex-K content of < 0.15 g kg−1, inorganic K fertilizer is applied at 83 kg K ha−1 (100 kg ha−1 as potassium oxide (K2O) equivalent); at an Ex-K content of 0.15–0.30 g kg−1, the application rate is reduced to 33 kg K ha−1 (40 kg K2O ha−1); at an Ex-K content of ≥ 0.30 g kg−1, inorganic K fertilizer is not applied because of sufficient K in the soil. Additionally, we propose that cattle manure compost be used to supplement soil K fertility.
INTRODUCTION
In Japan, corn (Zea mays L.) is a major forage crop for silage production, with a planted area of 91,900 ha in 2014 corresponding to about 10% of total planted area for forage crops (MAFF Citation2015). Forage corn for silage is more commonly used as self-supplying feed in dairy farming because of its high energy, and is cultivated with the application of organic fertilizer from cattle excretion in combination with inorganic potassium (K) fertilizer. Cattle excretion is mainly used as compost after the enforcement of the “Act on the Appropriate Treatment and Promotion of Utilization of Livestock Manure” in 1999. Soil fertility of K and the other nutrients in a forage corn field probably increases with successive and excessive applications of K fertilization. Soil diagnosis is an essential tool to achieve a target yield and to avoid excessive fertilization.
The exchangeable potassium (Ex-K) content extracted by 1 mol L−1 ammonium acetate solution based on the method of Schollenberger and Simon (Citation1945) is generally used to evaluate soil K fertility in Japan. In addition to ammonium acetate solution, Bray No. 1 (Bray and Kurtz Citation1945), Mehlich 3 (Mehlich Citation1984) and the Olsen method (Olsen et al. Citation1954) were used as the extraction methods for Ex-K in the United States where corn for silage was cultivated over large areas (Ketterings et al. Citation2003; Leikam et al. Citation2003; Bundy Citation2004; Gerwing and Gelderman Citation2005; Shapiro et al. Citation2008; Brown et al. Citation2010).
In Kanto and Tokai regions, in the central area of Honshu, Japan, diagnostic ranges of Ex-K content based on dry soil weight for obtaining a stable yield are 0.12–0.42 g kg−1 according to regional K fertilizer recommendation for volcanic ash soils with a cation exchange capacity (CEC) of 20–35 cmolc kg−1 (NGRI Citation1988). Additionally, diagnostic ranges of Ex-K are 0.12–0.25 g kg−1 for other (non-volcanic ash) soils with a CEC of 10–20 cmolc kg−1. Regional recommended application rates of inorganic K fertilizer for corn showed a median value of 133 kg K ha−1 (160 kg ha−1 as potassium oxide (K2O) equivalent) with a median target yield of 65 Mg ha−1 on a fresh weight (FW) basis for 14 prefectures, not including Hokkaido Prefecture in Japan. The mean rate for Hokkaido prefecture was 110 kg K ha−1 with the mean target yield of 60 Mg FW ha−1 (MAFF Citation2009). Application rates of K fertilizer are decreased or increased to respond to excess or deficient soil Ex-K contents. However, most of the levels of soil Ex-K content in forage corn fields were much higher than the diagnostic Ex-K ranges (Hatanaka et al. Citation1983; Sano et al. Citation1984; Ide and Inomoto Citation1994; Minegishi et al. Citation1995; Anzai et al. Citation1998; Yamada and Kubotera Citation1999; Wakahara et al. Citation2001). These data showed that soil K fertility in forage corn field trended to increase. Therefore, the application rate of inorganic K fertilizer for forage corn production could be reduced by clarifying the levels of Ex-K content needed for achieving the target yield with low K fertilization. However, relationships between soil K fertility expressed as Ex-K content and corn yield or K uptake are not clear in Japan.
Harada et al. (Citation2001) reported that the K concentration of corn was a genetic character and K uptake by corn was positively correlated with K concentration among the corn varieties. Consideration of the varietal differences for K uptake is required for evaluating relationships between corn production and soil K fertility or K fertilizer application. Commercial corn varieties are replaced after several years in Japan. Therefore, K fertilization based on soil diagnosis having flexibility for the varietal turnover would be developed by clarifying those relationships for varieties with minimum and maximum K uptakes among commercial varieties.
In the present study, varieties with different K uptakes were selected, and relationships between yields or K uptakes and Ex-K contents used as an indicator for evaluating soil K fertility, were examined in field experiments. Our objectives were to develop effective soil diagnostic criteria for Ex-K content combined with inorganic K fertilizer application rates for high yield and low inorganic K fertilizer input in forage corn production as a model and to propose a new management of K fertilizer application replacing the conventional K management (NGRI Citation1988).
MATERIALS AND METHODS
We designed three experiments for achieving the objectives: using corn varieties with different K uptakes selected in 2003–2004 (Experiment 1), responses of yield, K concentration and K uptake of corn to soil K fertility were examined to clarify the level of soil Ex-K content for achieving a target yield with no inorganic K fertilizer application as a diagnostic value in 2005–2007 (Experiment 2). Additionally, effects of K fertilizer application on corn growth under conditions of low and high soil K fertility were also examined to set up K fertilizer application rates corresponding to soil Ex-K contents in 2008–2010 (Experiment 3).
The target yield was set at 18 Mg dry weight (DW) ha−1 in this study: we considered using 65 Mg FW ha−1 as the mean target FW yield in 14 prefectures, not including Hokkaido Prefecture (MAFF Citation2009), and 0.27–0.28 kg kg−1 as the dry matter content in corn harvested at the yellow-ripe stage. The target yield was higher in comparison with the mean actual yield of approximately 14 Mg DW ha−1 in Japan during 2002–2010 (MAFF Citation2012). A developing K management for achieving this target yield can be expected to be in widespread use in Japan excluding Hokkaido.
Study site
Forage corn varieties were cultivated under different levels of Ex-K content in experimental fields with or without applications of cattle manure compost (CMC) at the Nasu Research Station of the National Agriculture and Food Research Organization (NARO) Institute of Livestock and Grassland Science (NILGS) from 2003 to 2010. The NILGS is located at latitude 36°55′N, longitude 139°55′E and 322 m above sea level in Tochigi Prefecture, the northern Kanto region of Honshu, Japan. Its surrounding area is one of the main dairy farming areas in Honshu. The soil type was a Brown Lowland soil, which can be classified as Entic Humudept, fragmental, mixed, mesic (Kurashima et al. Citation1993; Soil Survey Staff Citation2014). The soil surface horizon contains volcanic ash.
Weather data were obtained from meteorological observation equipment at the NILGS. The mean air temperature from June 1 to September 10 (102 d), as the main growing period of corn in this study, was 20.7, 22.1, 22.5, 22.0, 21.9, 21.6, 21.4 and 23.7°C for each year in 2003–2010, versus a three-decade average (normal value) of 21.6°C. The total precipitation for June 1 to September 10 was 618, 552, 1073, 770, 803, 1015, 754 and 861 mm each for 2003–2010, with 741 mm as the normal value. The cumulative sunshine received totaled 1128, 1534, 1249, 1300, 1519, 1529, 1483, 1647 and 1335 MJ m−2 for the respective periods. Low air temperatures in 2003 and 2009 were observed as compared with the normal value. The amount of rainfall in 2005–2010 through the Exp. 2 and 3 periods tended to be higher than the normal value. The lowest duration of sunshine was observed in 2003, as was the lowest air temperature. The highest precipitation occurred in 2005 with low duration of sunshine.
Practices common to all experimental cultivations of forage corn
The sowing rate of corn varieties tested was 6.67 plants m−2 with a row and intrarow spacing of 0.75 m and 0.20 m, respectively. Herbicides, alachlor and atrazine, were sprayed on the soil surface of experimental fields for weed control after sowing. All corn samples were harvested at the yellow-ripe stage as the optimum stage for silage making. In 2004, portions of the corn varieties were harvested at an earlier stage (from the end of dough stage to the early yellow-ripe stage), because of lodging resulting from a typhoon. Italian ryegrass (Lolium multiflorum Lam.), rye (Secale cereale L.), triticale (× Triticosecale Wittmack) and oat (Avena sativa L.) for animal consumption were grown as winter forage crops after corn cultivation every year.
All CMC applied consisted of composted cattle excretion with sawdust and was mainly obtained from Shiobara compost center near the NILGS. The CMC had been biannually applied in fields at each rate before establishment of each corn and winter forage crop. Average water content (FW basis) and nutrient contents (DW basis) in the CMC during the experimental periods were as follows: water content 0.612 ± 0.064 kg kg−1, carbon/nitrogen (C/N) ratio 22.0 ± 4.4, N 18.6 ± 3.3 g kg−1, phosphorus (P) 7.70 ± 2.83 g kg−1, and K 22.6 ± 4.4 g kg−1. Details of the CMC analysis are provided in the following section.
Experiment 1
Twenty commercial varieties, 33G26, 33J24, Cecilia, DK567, DK708, DK727, DKC61-24, FC113GP, KD620, KD640, KD670, KD720, Nasuhomare, NS68, NS68A, NS80A, TS115, TX105, TX230 and Yumechikara, were cultivated for 2 years. Randomized block design with two replications was used. All varieties tested were early maturing with relative maturity (RM) values from 105 to 118. The RM value is used as an indicator for earliness of corn variety in Japan. Seeds were sown in a plot area per variety of 5.75 m2 on July 2, 2003 and July 1, 2004. The corn plants were harvested on September 16–17, 2003 and September 2, 2004.
These plots were maintained under high N and K fertilizer application rates with 150 Mg FW ha−1 CMC in both years, similar to the method used by Harada et al. (Citation2001). Nutrient inputs from the CMC were 700 and 780 kg ha−1 for N, 371 and 340 kg ha−1 for P and 805 and 1038 kg ha−1 for K in 2003 and 2004, respectively.
Soil chemical properties in the field before sowing were as follows; pH 6.1 ± 0.2, total C 76.1 ± 0.5 g kg−1, total N 5.98 ± 0.23 g kg−1, available P 0.58 ± 0.07 g kg−1; and Ex-K, exchangeable calcium (Ex-Ca) and exchangeable magnesium (Ex-Mg) 1.04 ± 0.13, 5.19 ± 0.22 and 0.85 ± 0.08 g kg−1, respectively. The Ex-K content was 8.7 times higher than the conventional diagnostic value for Ex-K content (0.12 g kg−1), which was likely caused by repeated CMC applications. The method for soil analysis is described in the following section.
Ammonium sulfate, fused magnesium phosphate and potassium chloride were stripe-applied on the soil surface as a basal dressing at 150 kg N ha−1, 65.5 kg P (150 kg as phosphorus pentoxide (P2O5) equivalent) ha−1, and 125 kg K (150 kg as K2O) ha−1, respectively, on May 30, 2003, and June 1, 2004. Ammonium sulfate and potassium chloride were also applied as an additional dressing of 150 kg N ha−1 and 125 kg K (150 kg as K2O) ha−1 at a time on July 2, August 6 and 29, 2003, and July 9, August 6 and 25, 2004, respectively. Thus, total nutrient inputs from the inorganic fertilizers were 450, 65.5 and 500 kg ha−1 each for N, P and K in both years.
Experiment 2
This experiment was conducted using five experimental fields, named fields A, B, C, D and E, with or without CMC applications. No inorganic K fertilizer was applied, so that we could examine the effects of soil K on corn production. The CMC application rates were 0 Mg FW ha−1 for fields A and B, 50 Mg FW ha−1 for field C and 100 Mg FW ha−1 for fields D and E. In fields A, B and D, CMC application had been carried out since 2002. For fields C and E, CMC had been applied for more than 10 years. The five fields had different levels of Ex-K contents because of the different application rate and history of CMC. Calcium carbonate, to prevent soil acidification, was spread on fields A and B with no CMC application at 117 g m−2 after plowing and was mixed with the topsoil by rototilling. Ammonium sulfate and fused magnesium phosphate were applied to all experimental fields as basal dressings at 220 kg N ha−1 and 87.3 kg P (200 kg as P2O5) ha−1, respectively. Two commercial varieties with different K uptakes (cv. ‘Cecilia’ and ‘Yumechikara’) were used based on results from Exp. 1. Seeds of the corn varieties were sown in a plot area of 5.75 m2 on June 1, 2005, June 6, 2006, and June 1, 2007. The basal dressing dates were June 2, 2005, June 5, 2006, and May 29, 2007. Corn plants were harvested on September 7, 8 and 12, 2005, September 11–13, 2006, and September 12–13, 2007.
Randomized block design with three replications was used in each field. The tested varieties were randomly assigned to a block, with six plots (2 varieties × 3 replications) in each field.
Experiment 3
Varieties ‘Cecilia’ and ‘Yumechikara’ were cultivated in field A with a low soil K fertility to examine inorganic K fertilizer application rate from 2008 to 2010. Field A was also used as an experimental field with the lowest Ex-K content in Exp. 2. Additionally, field F with 30 Mg FW ha−1 CMC was used to clarify the effects of inorganic K fertilizer application on corn growth under a high soil Ex-K content in 2009.
In field A, potassium chloride was applied at 0, 83, 166 and 249 kg K ha−1, corresponding to 0, 100, 200 and 300 kg ha−1 as K2O equivalent, respectively. Ammonium sulfate and fused magnesium phosphate were applied at 220 kg N ha−1 and 87.3 kg P (200 kg as P2O5) ha−1, respectively. Seeds were sown in a plot area of 5.75 m2 on June 4, 2008, June 2, 2009, and May 28, 2010. Fertilizer applications were carried out as basal dressings on June 2, 2008, June 1, 2009, and May 28, 2010. Corn plants were harvested on September 5, 2008, September 8, 10 and 15, 2009, and September 8, 2010.
For field F, potassium chloride was applied at 0, 41.5, 83, 166 and 249 kg K ha−1 (0, 50, 100, 200 and 300 kg ha−1 as K2O equivalent for the respective rates) on June 1, 2009. Ammonium sulfate and fused magnesium phosphate were applied with K fertilization at 220 kg N ha−1 and 87.3 kg P (200 kg as P2O5) ha−1, respectively. Seeds were sown in a plot area of 6 m2 on June 2, 2009. Corn plants were harvested on September 8, 10 and 15, 2009.
Randomized block design with three replications was used in each field; the treatments of K fertilization were randomly assigned as a primary factor to a block. Thus, there were 24 plots in total (2 varieties × 4 treatments × 3 replications) in field A and 30 plots (2 varieties × 5 treatments × 3 replications) in field F.
Plant harvesting and analysis
Five plants in a plot area were harvested at the yellow-ripe stage in the experimental fields by cutting at a height of 10 cm above ground, cut into leaf, stem and ear sections, dried in an oven at 70°C for 72 h, and weighed. Yield was determined as DW of whole crop above ground, including leaf, stem and ear, per unit area. Samples were then ground using a Wiley mill with a 2-mm screen (1029-JC; Yoshida Seisakusho, Tokyo, Japan) and a high-speed vibrating sample mill (TI-100;CMT, Fukushima, Japan) for analysis. Total K content was determined using atomic absorption photometers (Z-8100 and Z-2300; Hitachi, Tokyo, Japan) after wet digestion with nitric acid and perchloric acid.
K uptake is defined as K amount in corn above ground per unit area calculated from multiplying yield by K concentration. Apparent K recovery rate of K uptake by corn to K application rate, and K balance between K uptake by corn and K application rate were calculated as follows:
where R is the apparent K recovery rate (%), UF is the K uptake (kg K ha−1) at each K fertilizer application rate, U0 is the K uptake (kg K ha−1) at the K fertilizer application rate of 0 kg K ha−1, FR is the K fertilizer application rate (kg K ha−1) and B is the K balance (kg K ha−1).
Soil sampling and analysis
After CMC was applied to the fields, followed by plowing and rototilling, we collected soil samples from the topsoil to a depth of 10 cm at three points in each block. The three samples from each block were then mixed to give one soil sample per block, corresponding to three soil samples per field for soil chemical property analysis. The samples were collected by shovel before sowing and fertilizing on May 29, May 31, May 30, July 2, May 28, July 2, May 27 and May 21 of each year, respectively, from 2003 to 2010. In addition, we divided the field used in Exp. 1 into three parts to collect soil samples by the same method as the other fields.
Fresh soil samples were put through a 2-mm sieve to remove coarse materials and plant materials such as roots. Part of the soil sample was dried in an oven at 105 °C for 24 h and weighed to determine water content. Soil pH was measured using a grass electrode pH meter (F-21 and F-52; Horiba, Kyoto, Japan) by adding 10 g DW equivalent of an undried soil to 25 mL of water (including soil water). The nitrate N content of soil was spectrophotometrically analyzed with a flow injection system (L4250 UV-VIS detector; Hitachi, Tokyo, Japan) using the sulfanilamide-naphthyl ethylene diamine method after extracting 20 g DW equivalent of an undried soil sample with 80 mL of 2 mol L−1 potassium chloride solution. The ammonium N content of soil was analyzed using the indophenol method (Ohyama et al. Citation1991) after the same method of extraction. The inorganic N content was calculated as the sum of the nitrate- and ammonium-N contents.
The soil was then air-dried, and exchangeable cations (Ex-K, Ex-Ca and Ex-Mg) were extracted from 1 g of air-dried soil with 200 mL of a solution of 0.05 mol L−1 ammonium acetate and 0.0114 mol L−1 strontium chloride (Kamewada and Shibata Citation1997) and determined by atomic absorption spectrometry (AAS). Kamewada and Shibata (Citation1997) reported that the measured values of K contents in the extraction corresponded to those extracted by 1 mol L−1 ammonium acetate solution adjusted to pH 7.0 based on the method of Schollenberger and Simon (Citation1945). The CEC was determined by ammonium ion analysis of extraction with an automatic stirrer (CEC-10; Fujihira Industry, Tokyo, Japan). The ammonium N content of the extract was analyzed by the indophenol method. Total C and N contents were analyzed using a CN microanalyzer (JM1000CN; J-Science Lab, Tokyo, Japan). The available P content was spectrophotometrically determined at 710 nm using the molybdenum blue colorimetric method after extraction by the Truog method (Truog Citation1930). All soil chemical properties except pH were expressed on a dry soil weight basis.
CMC analysis
A sample of fresh CMC was dried by the same method as soil to determine water content. The CMC samples were dried in an oven at 70°C for 72 h, and then were ground into a powder in the same way as the plant samples were for analysis. The C and N contents were analyzed using the CN microanalyzer. After the wet digestion with nitric acid and perchloric acid for the dried samples, the P and K contents were determined by the molybdenum blue colorimetric method and AAS, respectively.
Statistical analysis
We tested the differences in yield, and K concentration and K uptake between the corn varieties in Exp. 1 and between the K treatments in Exp. 3 by Tukey’s honestly significant difference (HSD) test, the yearly and the varietal differences of yield in Exp. 2 by analysis of variance (ANOVA), and the differences of regression equation in Exp. 2 by analysis of covariance (ANCOVA) using version 3.0.2 of the R software (R Core Team Citation2013). We defined significance at P < 0.05. Regression analyses for relationships between Ex-K content in soil and K uptake, K concentration or yield for corn in Exp. 2 were performed using JMP version 8.0.2.2 (SAS Institute, Cary, NC, USA).
RESULTS
Selection of corn varieties with characteristics for K uptake (Exp. 1)
There were significant differences in yield, and K concentration and K uptake of corn (). Differences between minimum and maximum values were 1.5 times for yield, 1.4 times for K concentration and 1.7 times for K uptake. K uptake had significant positive relationships with both yield (Pearson’s correlation coefficient r = 0.70) and K concentration (r = 0.66). We evaluated varieties with low productivity unsuitable for the target yield of 18 Mg DW ha−1 in this study, and so selected two varieties based on K concentration among 17 varieties, excluding three varieties with lower yields as compared with the target yield. Additionally, the varieties ‘33G26ʹ and ‘Nasuhomare’ were also omitted from the subjects of selection due to end of sale, although these varieties showed low K concentrations. The varieties ‘Cecilia’ and ‘Yumechikara’ were selected for testing in Exp. 2 and 3, as they were the lowest and the highest variety for K concentration, respectively (). The K uptake of ‘Yumechikara’ was about 1.3 times higher than that of ‘Cecilia,’ corresponding to the difference in K concentration. Both varieties had similar RM values (‘Cecilia’, 115; ‘Yumechikara’, 114). The yields of both varieties were on the same level with the mean value of the 20 varieties ().
Table 1 Varietal differences in yield, potassium (K) concentration and K uptake for forage corn (Zea mays L.) at the yellow-ripe stage (Experiment 1).
Responses of yield, K concentration and uptake in corn to soil K fertility (Exp. 2)
An outline of the design of CMC applications and soil chemical properties in five fields used for Exp. 2 is shown in . The Ex-K contents in soil tended to increase from 0.11 to 0.92 g kg−1 with the increase in CMC application rate. Total C and N, available P, Ex-Ca and Ex-Mg contents and CEC in field E were higher than those in the other fields because of its long history of high CMC application. Total N and available P contents also tended to rise with CMC application. Adequate N and P fertilization for corn growth were achieved despite differences in soil fertility.
Table 2 Field management and soil chemical properties of five experimental fields in Experiment 2.
Yields were within the ranges of 14.4 to 21.1 Mg DW ha−1 for ‘Cecilia’ and 16.3 to 20.8 Mg DW ha−1 for ‘Yumechikara’, when K uptakes by corn plants changed from 96 to 303 kg K ha−1 for ‘Cecilia’ and from 123 to 411 kg K ha−1 for ‘Yumechikara’ (). Yields increased with the increases in K uptake by each corn variety under the conditions where inorganic K fertilizer was not applied. There was no significant varietal difference of regression equations (not shown) for the relationships between corn yield and K uptake resulting from ANCOVA. Therefore, the yield of corn could be explained by the K uptake according to a common regression equation for both varieties as follows:
Figure 1 Relationship between the potassium (K) uptake by corn (Zea mays L.) and the corn yield in Experiment 2. Results consist of the data in 2005 (n = 4), 2006 (n = 4) and 2007 (n = 4), and are expressed as means ± standard deviation. The varieties ‘Cecilia’ and ‘Yumechikara’ are shown as circles (○) and squares (□), respectively. The solid lines are expressed as a regression curve in common with both varieties. The regression curve with the coefficient of determination (R2) using the yield as an outcome variable (y) and the K uptake by corn as a predictor variable (x) was y = 3.581 ln(x) – 1.11 with R2 = 0.623. The vertical and horizontal dashed lines indicate a K uptake of 200 kg ha−1 and a target yield of 18 Mg dry weight (DW) ha−1, respectively.
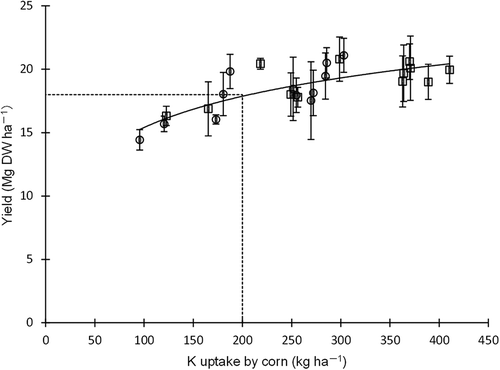
where Y is the yield of corn (Mg DW ha−1) and ln (U) is the natural logarithm of K uptake by corn (kg K ha−1). The K uptake by corn for achieving the target yield of 18 Mg ha−1 was estimated to be approximately 200 kg K ha−1 in common with the two varieties using Eq. 3.
The K uptake by corn plants increased with the increase in soil Ex-K contents (). The changes in K uptake could be simulated by the soil Ex-K content with high degrees of accuracy using the equations, as follows:
Figure 2 Relationship between the exchangeable potassium (K) content as an indicator for soil K fertility and the potassium uptake by corn (Zea mays L.) in Experiment 2. Results consist of the data in 2005 (n = 4), 2006 (n = 4) and 2007 (n = 4), and are expressed as means ± standard deviation. The varieties ‘Cecilia’ and ‘Yumechikara’ are shown as circles (○) with a lower solid line and squares (□) with an upper solid line, respectively. The regression curve with the coefficient of determination (R2) using the K uptake by corn as an outcome variable (y) and the exchangeable potassium (Ex-K) content in soil as a predictor variable (x) was y = 82.65 ln(x) + 298.4 with R2 = 0.923 for cv. Cecilia and y = 114.2 ln(x) + 402.1 with R2 = 0.941 for cv. Yumechikara. The vertical dashed lines indicate Ex-K contents of 0.17 and 0.30 g kg−1. The horizontal dashed line shows a K uptake of 200 kg ha−1. DW, dry weight.
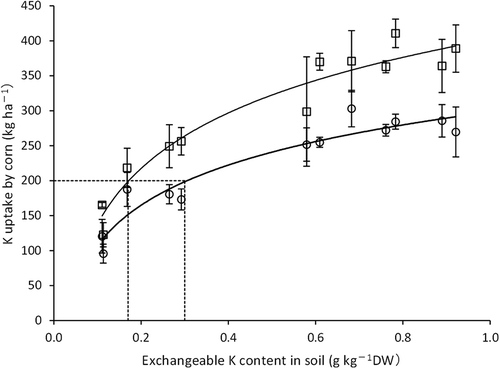
where U is the K uptake by corn (kg K ha−1) and ln (Cs) is the natural logarithm of Ex-K content in soil (g kg−1). The soil Ex-K content for achieving the corn K uptake of 200 kg K ha−1 with no K fertilizer application was calculated to be 0.30 g kg−1 for ‘Cecilia’ and 0.17 g kg−1 for ‘Yumechikara’ using Eq. 4 and 5, respectively. Therefore, the target yield of 18 Mg DW ha−1 was probably obtained at these Ex-K contents each for variety.
The K concentrations of ‘Cecilia’ ranged from 6.6 to 15.5 g kg−1 DW and were lower than those of ‘Yumechikara,’ which ranged from 7.5 to 20.6 g kg−1 DW throughout the tested range of soil Ex-K content (). The K concentrations of both varieties increased with the increase in the soil Ex-K contents, and could be simulated with high degrees of accuracy using the equations:
Figure 3 Relationship between the exchangeable potassium (Ex-K) content in soil and the potassium (K) concentration in corn (Zea mays L.) in Experiment 2. Results consist of the data in 2005 (n = 4), 2006 (n = 4) and 2007 (n = 4), and are expressed as means ± standard deviation. The varieties ‘Cecilia’ and ‘Yumechikara’ are shown as circles (○) with a lower solid line and squares (□) with an upper solid line, respectively. The regression curve with the coefficient of determination (R2) using the K concentration in corn as an outcome variable (y) and the Ex-K content in soil as a predictor variable (x) was y = 3.804 ln(x) + 15.62 with R2 = 0.969 for cv. Cecilia and y = 5.221 ln(x) + 20.24 with R2 = 0.915 for cv. Yumechikara. The vertical dashed lines indicate Ex-K contents of 0.17 and 0.30 g kg−1. The horizontal dashed line shows a K concentration of 11 g kg−1 dry weight (DW).
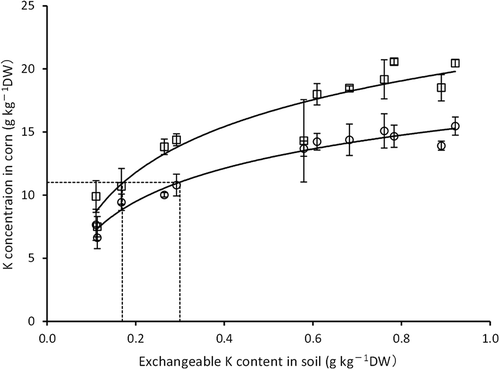
where Cp is the K concentration in corn (g kg−1 DW) and ln (Cs) is the natural logarithm of Ex-K content in soil (g kg−1). Calculated K concentration of ‘Cecilia’ at the Ex-K content of 0.30 g kg−1 for the target yield were 11 g kg−1 DW corresponded with that of ‘Yumechikara’ at the Ex-K content of 0.17 g kg−1. Differences in K concentration between minimum and maximum values each for variety were larger than those for yield. Therefore, K uptake had a higher positive relationship with K concentration (r = 0.964 for ‘Cecilia’ and 0.986 for ‘Yumechikara’) than that with yield (r = 0.799 and 0.699 each for variety, respectively), although there were statistically significant relationships with both factors (P < 0.05). The changes in K concentration () were similar to those in K uptake by corn ().
The yields increased with the increase in the soil Ex-K contents (). The yields of ‘Cecilia’ and ‘Yumechikara’ had significant positive relationships with soil Ex-K content, with r-values of 0.578 and 0.587 (P < 0.05), respectively. It was observed for both varieties that the target yield of 18 Mg DW ha−1 was obtained at the Ex-K of 0.30 g kg−1 or more for achieving the K uptake of 200 kg K ha−1 (). The relationships could be described by quadratic regression curves according to following equations. However, the degrees of accuracy were low for ‘Cecilia’ and moderate for ‘Yumechikara’.
Figure 4 Relationship between the exchangeable potassium (Ex-K) content in soil and the yield of corn (Zea mays L.) in Experiment 2. Results consist of the data in 2005 (n = 4), 2006 (n = 4) and 2007 (n = 4), and are expressed as means ± standard deviation. The varieties of ‘Cecilia’ and ‘Yumechikara’ are shown as circles (○) with a lower solid line and squares (□) with an upper solid line, respectively. The regression curve with the coefficient of determination (R2) using the yield as an outcome variable (y) and the Ex-K content in soil as a predictor variable (x) was y = − 7.843x2 + 11.52x + 14.91 with R2 = 0.389 for cv. Cecilia and y = − 11.64x2 + 14.37x + 15.75 with R2 = 0.561 for cv. Yumechikara. The vertical and horizontal dashed lines indicate an Ex-K content of 0.30 g kg−1 and a yield of 18 Mg dry weight (DW) ha−1, respectively. K, potassium.
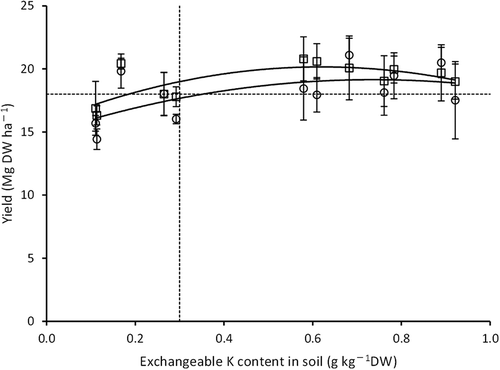
where Y is the yield of corn (Mg DW ha−1) and Cs is the Ex-K content in soil (g kg−1). When the soil Ex-K contents were 0.30 g kg−1 for ‘Cecilia’ and 0.17 g kg−1 for ‘Yumechikara’ as the levels for satisfying the minimum K requirement for the target yield with no K fertilization (), their yields were estimated to reach approximately 18 Mg DW ha−1 using Eq. 8 and 9. These results indicated the target yield could be obtained at the soil Ex-K content of 0.30 g kg−1 or more for both varieties; hence, the soil Ex-K content of 0.30 g kg−1 was commonly available as a diagnostic value for achieving the target yield with no K fertilizer application.
The mean yield of 17.9 Mg DW ha−1 for ‘Yumechikara’ was significantly higher than that of 16.8 Mg DW ha−1 for ‘Cecilia’ at Ex-K contents under 0.30 g kg−1 (ANOVA, P < 0.05), while no statistical significant difference for yield was observed between ‘Cecilia’ and ‘Yumechikara’ at Ex-K contents of 0.30 g K kg−1 or more. The variety ‘Yumechikara’ had a higher productivity at the low soil K fertility as compared with ‘Cecilia’. Field C with an Ex-K content of 0.58–0.68 g kg−1 was used through the experimental period. There were no significant differences of yield in this field between years: 20.6 Mg DW ha−1 in 2005, 19.3 Mg DW ha−1 in 2006 and 19.6 Mg DW ha−1 in 2007 (ANOVA). This result indicates that environmental conditions such as the high rainfall and low duration of sunshine in 2005 had little effect on corn growth in Exp. 2.
Effect of K fertilizer application on corn growth under conditions of low and high soil K fertility (Exp. 3)
Soil Ex-K contents in field A were approximately 0.1 g kg−1 during the test periods for Exp. 3 (). The lowest yields for both varieties ‘Cecilia’ and ‘Yumechikara’ were observed at this level of Ex-K content in Exp. 2 (). In contrast, field F had a higher soil Ex-K content of 0.37 g kg−1 than the diagnostic value (i.e., 0.30 g kg−1) for the target yield with no K application resulting from Exp. 2 (). Soil pH values were generally within the proper range of 6.0–6.5, and the available P contents were adequate levels for corn production, within the diagnostic range of 0.04–0.13 g kg−1 for available P as suggested by NGRI (Citation1988).
Table 3 Soil chemical properties of fields A and F in Experiment 3.
In field A with low soil K fertility, the yield of ‘Cecilia’ with no K fertilization was at the same level as that of ‘Yumechikara’ (). Yields of ‘Cecilia’ increased significantly with increases in K application, although yield differences were not observed for ‘Yumechikara’. These yields achieved the target yield of 18 Mg DW ha−1 at a K fertilizer application rate of 83 kg K ha−1 (100 kg K2O ha−1). There was strong evidence that low soil K fertility was the limiting factor for yield in Exp. 3 based on the positive effect of K fertilization on the increased yields.
Table 4 Response of corn (Zea mays L.) to potassium fertilizer application under low soil potassium fertility (Experiment 3).
The K concentrations of both varieties were also increased by high K application rates, and the K concentrations of ‘Yumechikara’ were higher than those of ‘Cecilia’ at all K application levels (). The K uptake of both varieties increased linearly with the change in K application rate from 0 to 166 kg K ha−1, and plateaued at a rate of 166 kg K ha−1 (). The K uptakes at the K application rate of 83 K ha−1 achieving the target yield—that is, 164 kg K ha−1 for ‘Cecilia’ and 191 kg K ha−1 for ‘Yumechikara’—were lower than the minimum K requirement of 200 kg K ha−1 resulting in Exp. 2 (). The high-K-uptake variety ‘Yumechikara’ had negative K balances at all K application rates, while ‘Cecilia’ showed a positive K balance at a K application rate of 249 Kg K ha−1. These results for K balance indicate that soil K fertility is consumed by harvesting corn plants at a K application rate of 83 kg K ha−1 for the target yield. Linear increases in K uptake were observed at application rates of 0 to 166 kg K ha−1, and the apparent K recovery rate was approximately 70% at K application rates of 83 and 166 kg K ha−1 for both varieties. The apparent K recovery rate of ‘Yumechikara’ was similar to that of ‘Cecilia’ despite its high K uptake. Therefore, ‘Yumechikara’ had a relatively high absorption capacity of K accumulated in soil. However, ‘Cecilia’ had a relatively high dry matter productivity to inorganic K fertilizer application because of its higher increase of yield by the K application as compared with that of ‘Yumechikara’ ().
In field F with high soil K fertility, yields exceeded the target yield of 18 Mg DW ha−1 with no K application and were not changed by K application for both varieties (). There were no significant differences in K uptakes and K concentrations for both varieties between K application rates from 0 to 249 kg K ha−1 excluding the K concentration in ‘Cecilia’ (). Large amounts of K applied as inorganic fertilizer were retained in soil because of the low K recovery rates, with only 11% as the maximum value.
Table 5 Response of corn (Zea mays L.) to potassium fertilizer application under high soil potassium fertility (Experiment 3).
Formulation of K fertilizer recommendation with effective use of soil K fertility
When Ex-K content in soil was more than 0.30 g kg−1, the K uptake of 200 kg K ha−1 and the target yield of 18 Mg ha−1 were achieved with no inorganic K fertilizer application ( and ), and inorganic K fertilizer application had no effect on corn yield () in both varieties. Hence, we determined the Ex-K content of 0.30 g kg−1 as the diagnostic value at which there is no need for inorganic K fertilizer application. Inorganic K fertilizer application of 83 kg K ha−1 was required for achieving the target yield at the Ex-K content of about 0.1 g kg−1 (). The increase in K uptake between the K application rates of 83 and 166 kg K ha−1 contributed mainly to raise the K concentration in corn, because differences of the K concentrations between the K application rates (1.26 times for ‘Cecilia’ and 1.32 times for ‘Yumechikara’) were larger than those of the yield (1.10 times for ‘Cecilia’ and 1.01 times for ‘Yumechikara’; ). Therefore, we concluded that inorganic K fertilizer application at rates in excess of 83 kg K ha−1 were not required at a soil Ex-K content of 0.1 g kg−1 because higher K levels did not contribute to yield increase.
We defined the Ex-K content of 0.15 g kg−1 corresponding to half of the diagnostic value of 0.30 g kg−1 as a middle condition of soil K fertility. K uptakes at the Ex-K content of 0.15 g kg−1 were calculated to be about 142 kg K ha−1 for ‘Cecilia’ and 185 kg K ha−1 for ‘Yumechikara’ using Eq. 4 and 5 (). The minimum K requirements of corn plants using inorganic K fertilizer for achieving the target yield were 164 kg K ha−1 for ‘Cecilia’ and 191 kg K ha−1 for ‘Yumechikara’ based on the data of K uptake at the K application rate of 83 kg K ha−1 (). The K shortages at the Ex-K content of 0.15 g kg−1 were estimated to be 22 kg K ha−1 for ‘Cecilia’ and 6 kg K ha−1 for ‘Yumechikara’ by subtracting the calculated K uptake from the minimum K requirement each for variety. Inorganic K fertilizer application rates for supplying these K deficiencies were calculated to be 31 kg K ha−1 for ‘Cecilia’ and 9 kg K ha−1 for ‘Yumechikara’ using the apparent K recovery rate of 70% (). Therefore, the inorganic K fertilizer application rate for achieving the target yield of corn at an Ex-K content of 0.15 g kg−1 was determined to be 31 kg K ha−1 in consideration of its adaptability to other varieties.
We considered a safety margin by adapting ranges of Ex-K content to the suggested K fertilizer application rates, especially under a low Ex-K condition: inorganic K fertilizer application rates at Ex-K contents of 0.1 g kg−1 and 0.15 g kg−1 were adapted for Ex-K content ranges of < 0.15 g kg−1 and 0.15–0.30 g kg−1, respectively. The applicable Ex-K range to each K fertilizer application rate was set up as follows: at an Ex-K content of < 0.15 g kg−1, the inorganic K fertilizer application rate is 83 kg K ha−1; at 0.15–0.30 g kg−1, the K application rate is 31 kg K ha−1; at ≥ 0.30 g kg−1, the K application rate is 0 kg K ha−1. Additionally, we determined the K fertilizer application rate of 31 kg K ha−1 (38 kg K2O ha−1) is expressed 33 kg K ha−1 (40 kg K2O ha−1) as the recommended rate from a practical standpoint.
DISCUSSION
Fertilizer recommendations are typically established presupposing a moderate soil fertility, and application of organic matter such as CMC is suggested to maintain and improve soil fertility in farmland. Therefore, careful consideration for the maintenance of soil K is required for the new K fertilization suggested. At the low soil Ex-K content of 0.1 g kg−1 in Exp. 3, K removal by harvesting was larger than the amount of K supplied from the inorganic K fertilizer at the application rate of 83 kg K ha−1 shown in . Therefore, K supplementation would be required to avoid consumption of soil K. High application of inorganic K fertilizer caused a high K concentration in corn but not a high yield, although inorganic K fertilizer application at 249 kg K ha−1 or more was needed to change to a positive K balance (). In addition, it is necessary to discuss the use of CMC because forage corn is generally produced with a CMC application in Japan. Thus, we suggest that CMC self-produced in cattle farming be applied at 20–30 Mg FW ha−1 to maintain soil K fertility, instead of using inorganic K fertilizer. The suggested CMC application rate corresponds to 180–270 kg K ha−1 based on approximately 9 g kg−1 FW of K contained in average CMC (Yamaguchi et al. Citation2000). The minimum K supplement for maintaining soil K fertility was calculated to be 81–108 kg K ha−1 based on the K balances at the inorganic K fertilizer application rate of 83 kg K ha−1 (). The recommended CMC application rate was set higher than the minimum inorganic K supplement on the assumption that K uptake would be increased by the CMC application and would differ among varieties. If K uptake by corn plants was 350 kg ha−1 (as mean of the estimated maximum values of ‘Cecilia’ and ‘Yumechikara’ by Eq. 4 and 5), this CMC application rate could compensate for K removal from harvested corn plants in combination with the inorganic K fertilizer application at the rate of 83 kg K ha−1. At an Ex-K content of 0.15 g kg−1, the inorganic K fertilizer application rate was estimated to be 31 kg K ha−1 in the preceding section. The estimated K application rate would correspond to a CMC application rate of only 3.4 Mg FW ha−1 based on the average CMC (Yamaguchi et al. Citation2000). With a CMC application, it would be possible to need no inorganic K fertilizer application. Suggested K fertilization based on soil Ex-K content for corn production is formulated in .
Table 6 Newly suggested potassium fertilization based on soil diagnosis of exchangeable potassium for low potassium input production of forage corn (Zea mays L).
Conventional management of K fertilization was prepared by NGRI (Citation1988) (). Inorganic K fertilizer application in conventional K management basically follows the recommended rate of K fertilization in each prefecture below the Ex-K content of 0.25 g kg−1 for non-volcanic ash soils and 0.42 g kg−1 for volcanic ash soils. Regional K fertilizer recommendations for corn had a median value of 133 kg K ha−1 with a range of 83 to 315 kg K ha−1 (MAFF Citation2009). Our suggested K fertilizer application rate of 83 kg K ha−1 under the low level of Ex-K content (< 0.15 g kg−1) is more than 30% lower than the median value, and corresponds to the minimum rate. The diagnostic criteria of Ex-K for volcanic ash soils are set up to be higher than those for non-volcanic ash soils because of the higher CEC of volcanic ash soils. However, it is unclear to what degree different soil CEC levels affect K uptake by corn. In a pot experiment using three types of volcanic ash soils with different CEC levels (4.7, 15.6 and 23.8 cmolc kg−1), K uptake by timothy (Phleum pratense L.) had a highly positive relationship with soil Ex-K content (r = 0.933, P < 0.01) despite the different soil CEC (Saigusa Citation1996). This report indicates that soil CEC has little effect on K uptake by timothy plants. The Brown Lowland soil in the experimental fields was classified as non-volcanic ash soils with a CEC of 10–20 cmolc kg−1 by the conventional diagnosis shown in . However, the soil CEC values of the fields in Exp. 2 and 3 were in the range of 20.7 to 33.4 cmolc kg−1 ( and ) and the variability was probably caused by CMC application. It is noted that application of organic fertilizers made from cattle excretion, such as compost, increases the CEC of soil (During and Weeda Citation1973; Ito et al. Citation1982; Yamada and Kamata Citation1989; Gil et al. Citation2008). Therefore, we set up the diagnostic criteria of soil Ex-K content with no distinction between the soil types on the assumption of positive CMC use. The Ex-K content of 0.30 g kg−1 as the novel diagnostic value for evaluating no K fertilizer application was lowered to 60% of the conventional value for non-volcanic ash soils (0.50 g kg−1) and 36% of that for volcanic ash soils (0.83 g kg−1).
Table 7 Conventional soil diagnosis criteria of exchangeable potassium content and potassium fertilization for forage crops for Kanto and Tokai regions in Japan (NGRI Citation1988).
In Exp. 2, corn yields increased with increasing Ex-K content mainly derived from CMC applications (). The maximum yield of 21 Mg DW ha−1 for both varieties was observed with no K fertilizer application. At low Ex-K conditions in Exp. 3, yields of ‘Cecilia’ significantly increased to 20 Mg DW ha−1 with increasing K fertilizer application rates, reaching a plateau at 166 kg ha−1 or more, while those of ‘Yumechikara’ tended to increase to 18 Mg DW ha−1 at a K fertilizer application rate of 83 kg K ha−1, as shown in . Therefore, corn yields over the maximum yield provided by K fertilization were probably the result of not only soil K fertility but also improved soil chemical and physical factors related to CMC applications. However, high input of CMC leads to excess K soil fertility as well as an excess of other nutrients. The K uptake values by corn plants at the soil Ex-K content of 0.30 g kg−1 achieving the target yield with no K fertilizer were calculated to be approximately 200 kg K ha−1 for ‘Cecilia’ and 265 kg K ha−1 for ‘Yumechikara’ using Eq. 4 and 5 (), respectively. These calculated K uptakes corresponded to the K amounts containing the average CMC (Yamaguchi et al. Citation2000) at the rate of 22 Mg FW ha−1 for ‘Cecilia’ and 29 Mg FW ha−1 for ‘Yumechikara’. Estimated maximum K uptakes by the same equations were 292 kg K ha−1 for ‘Cecilia’ and 393 kg K ha−1 for ‘Yumechikara’ at the maximum Ex-K content observed in Exp. 2 (), corresponding to about 32 and 44 Mg FW ha−1 for the CMC application rates, respectively. Therefore, a CMC application rate at 30 Mg FW ha−1 is recommended for corn production with a yield level of 18 Mg DW ha−1 because the permissible ranges of CMC application amounts were 22–32 Mg FW ha−1 for ‘Cecilia’ and 29–44 Mg FW ha−1 for ‘Yumechikara,’ which were balanced with K removal from harvested corn plants for each variety.
The amount of K in excretions of lactating dairy cows is generally changed by dietary K intake. Urinary K linearly increases with increasing K intake by lactating dairy cows (Kume et al. Citation2004). The amounts of K in excretion (feces and urine) of lactating Holstein cows were reported to be 285 and 212 g d−1 by Kume et al. (Citation1987), 257 g d−1 by Kume et al. (Citation2004), and 257 and 177 g d−1 by Ohtani et al. (Citation2010) in response to the K intake of 339, 266, 410, 333 and 259 g d−1 per unit cow, respectively. The yearly mean amount of K excreted by one lactating dairy cow was estimated to be 87 kg K y−1, multiplying the daily mean K amount in excretion based on the reported values by 365 days. The calculated K amount of 270 kg contained in the CMC 30 Mg FW as the recommended application rate was comparable to the estimated K amounts excreted by approximately three lactating dairy cows. Therefore, rising soil K fertility is probably caused by feeding large numbers of dairy cows and applying the resulting CMC to corn fields above our calculation of approximately three lactating dairy cows ha−1. Careful application of CMC is required to prevent excess K accumulation in soil.
The K requirement of lactating dairy cattle is 8 g kg−1 DW of total diet in the thermoneutral conditions in Japan (NARO Citation2006), and the maximum tolerable concentration is about 30 g kg−1 DW (NRC Citation2001; NARO Citation2006). High K intake from diet above the maximum can cause lowering of Ca and Mg use efficiencies and may cause some dairy cattle diseases, such as milk fever, grass tetany and udder edema (NRC Citation2001; NARO Citation2006). Low K concentrations in forage crops are desirable for fodder. The K concentrations of both corn varieties with the target yield were within the range between the K requirement and tolerance levels for dairy cattle (; and ), although the K concentrations of ‘Cecilia’ were lower than those of ‘Yumechikara’ under all of the experimental conditions. Therefore, corn is a suitable crop for management of K intake by dairy cattle.
The minimum K uptake for obtaining the target yield of corn with no K fertilizer application was estimated to be 200 kg K ha−1 for both varieties in Exp. 2 (; Eq. 3). On the other hand, lower K uptakes, i.e., approximately 160 kg K ha−1 for ‘Cecilia’ and 190 kg K ha−1 for ‘Yumechikara’, were observed as the minimum K requirement for the target yield with inorganic K application rate of 83 kg K ha−1 under the low soil K fertility in Exp. 3 (), when the K concentration of 8.9 g kg−1 for ‘Cecilia’ was obviously lower than the 11 g kg−1 estimated for the K uptake of 200 kg ha−1 in Exp. 2. It is likely that these differences of the K uptake as the K requirement resulted from different patterns for supplying K to corn plants between soil K and inorganic K fertilizer. Examining patterns of K absorption by corn during its growing period is required for clarifying these differences in K requirements. In this study, topsoil to a depth of 10 cm was used for evaluating the soil Ex-K content. Ito et al. (Citation1982) reported the effect of continuous heavy application of CMC on soil fertility in the Kyushu region of Japan. In their study, high accumulations of Ex-K in subsoil below the plow layer, i.e., a depth of 40 cm, were observed in experimental fields with CMC application rates of 200 Mg ha−1 y−1 or more in the fifth year after the application started, while it was not observed under CMC application rates of 100 Mg ha−1 y−1 or less. This study was unclear about the effects of subsoil K fertility on corn growth and K uptake. These points should be studied in future research.
In conclusion, we developed effective K fertilization and soil diagnostic criteria for low-K-input production of corn. Optimum practices for N and P fertilization and cultivation methods are also necessary for achieving the target yield of 18 Mg DW ha−1. Additional cultivation experiments for demonstrating its adaptability to different regions and soils will be required for use of the novel K fertilization based on soil diagnosis to become widespread in Japan. Our study should be used as the basis for lowering K input in corn production in regional K fertilizer recommendations.
ACKNOWLEDGMENTS
This work was partly supported by the Ministry of Agriculture, Forestry and Fisheries of Japan for “Integrated Research for Developing Japanese-Style Forage Feeding System to Increase Forage Self-Support Ration” and “Research for Production of Valuable Livestock by Feeding Self-Sufficient Forage Crops.” We thank Ms. Hiromi Kohyama of NILGS for her technical assistance in the field and in the laboratory work, and the staff of the Grassland Research Support Center of NILGS for their support during cultivation of the crops.
REFERENCES
- Anzai T, Shinoda M, Yamaki A, Tobe O, Arihara K, Watanabe H 1998: Status and changes of the properties of cultivated soil during a period of fifteen years in Chiba prefecture. Bull. Chiba Agric. Exp. Sta., 39, 71–86 (in Japanese with English summary).
- Bray RH, Kurtz LT 1945: Determination of total, organic, and available forms of phosphorus in soils. Soil Sci., 59, 39–46. doi:10.1097/00010694-194501000-00006
- Brown B, Hart J, Horneck D, Moore A 2010: Nutrient management for field corn silage and grain in the Inland Pacific Northwest. PNW615. University of Idaho Extension, the Oregon University Extension Service, Washington State University Extension, United States Department of Agriculture. http://www.cals.uidaho.edu/edcomm/pdf/PNW/PNW0615.pdf (December, 2013).
- Bundy LG 2004: Corn fertilization. A3340. University of Wisconsin-Extension, Cooperative Extension, United States Department of Agriculture. http://www.soils.wisc.edu/extension/pubs/A3340.pdf (December, 2013).
- During C, Weeda WC, Dorofaeff FD 1973: Some effects of cattle dung on soil properties, pasture production, and nutrient uptake. NZ J Agric. Res., 16, 431–438. doi:10.1080/00288233.1973.10421126
- Gerwing J, Gelderman R 2005: Fertilizer recommendation guide. EC750. South Dakota State University, South Dakota Cooperative Extension Service, United States Department of Agriculture. http://agbiopubs.sdstate.edu/articles/EC750.pdf (December, 2013).
- Gil MV, Carballo MT, Calvo LF 2008: Fertilization of maize with compost from cattle manure supplemented with additional mineral nutrients. Waste Manag., 28, 1432–1440. doi:10.1016/j.wasman.2007.05.009
- Harada H, Sunaga Y, Hatanaka T 2001: Variations in nutrient concentration among corn (Zea mays L.) varieties. Jpn. J. Grassl. Sci., 47, 289–295 (in Japanese with English summary).
- Hatanaka T, Kurashima K, Kimura T 1983: Studies on soil management with livestock excreta application. I. Soil chemical characteristics and problems of soil condition in the forage crop field and pasture. Bull Natl. Grassl. Res. Inst., 25, 48–59 (in Japanese with English summary).
- Ide T, Inomoto Y 1994: Soil properties of forage crop fields applied continuously large amounts of cattle feces. Kyusyu Agric. Res., 56, 69 (in Japanese).
- Ito Y, Shiozaki H, Hashimoto H 1982: Effects of continuous heavy-application of farmyard manure on the fertility of a humus-rich volcanic ash soil. Bull. Kyusyu Agric. Expt. Sta., 22, 259–320 (in Japanese with English summary).
- Kamewada K, Shibata K 1997: Simple extraction method for measuring exchangeable cations in soils that is not required for measuring cation exchange capacity. Jpn. J. Soil Sci. Plant Nutr., 68, 61–64 (in Japanese with English summary).
- Ketterings QM, Klausner SD, Czymmek KJ 2003: Potassium recommendations for field crops in New York. Second release. Department of Crop and Soil Extension Series, E03-14, Cornell University, NY. http://nmsp.cals.cornell.edu/publications/extension/Kdoc2003.pdf (December, 2013).
- Kume S, Kurihara M, Takahashi S, Shibata M, Aii T 1987: Effect of hot environmental temperature on major mineral balance in lactating cows. Jpn. J. Zootech. Sci., 58, 764–770.
- Kume S, Nonaka K, Oshita T, Kozakai T, Kojima H 2004: Potassium excretion of dry, pregnant and lactating cows fed forage. Nihon Chikusan Gakkaiho 75, 179–184 (in Japanese with English summary). doi:10.2508/chikusan.75.179
- Kurashima K, Ota T, Kusaba T, Amano Y, Yamamoto K, Kimura T, Kondo H, Saito G 1993: Classification and characteristics of soils at the national grassland research institute. Misc. Publ. Natl. Grassl. Res. Inst., 3, 1–47 (in Japanese).
- Leikam DF, Lamond RE, Mengel DB 2003: Soil test interpretations and fertilizer recommendations. MF-2586. Kansas State University Agricultural Experimental Station, Cooperative Extension Service, United States Department of Agriculture. http://www.agronomy.ksu.edu/soiltesting/doc1813.ashx (December, 2013).
- MAFF 2012: Yields of forage crops (herbage plant, corn and sorghum) in 2011. http://www.maff.go.jp/j/tokei/sokuhou/syukaku_siryou_11/ (December, 2014).
- MAFF 2015: Yields of forage crops (herbage plant, corn and sorghum) in 2014. http://www.maff.go.jp/j/tokei/sokuhou/syukaku_siryou_14/index.html (May, 2015).
- MAFF (Ministry of Agriculture, Forestry and Fisheries) 2009: Standard fertilizer application rate. http://www.maff.go.jp/j/seisan/kankyo/hozen_type/h_sehi_kizyun/ (November, 2009).
- Mehlich A 1984: Mehlich 3 soil test extractant: a modification of Mehlich 2 extractant. Commun. Soil Sci. Plant Anal., 15, 1409–1416. doi:10.1080/00103628409367568
- Minegishi S, Sunaga F, Saruta M, Imai Z, Tadaki M 1995: Soil fertility changes on Teiten soil survey in Gunma prefecture for the last 15 years. Bull. Gunma Agric. Exp. Stn., 1, 17–50 (in Japanese with English summary).
- NARO (National Agriculture and Food Research Organization) 2006: Potassium, sodium and chlorine. In Japanese Feeding Standard for Dairy Cattle (2006), Ed. NARO, pp. 15–16. Japan Livestock Industry Association, Tokyo.
- NGRI (National Grassland Research Institute) 1988: Report on the formulation of soil diagnostic criteria for forage field in the Kanto and Tokai region. Publication of the National Grassland Research Institute (Sôchi Shikenjyô Shiryô). 62–15, 30–37 (in Japanese).
- NRC (National Research Council) 2001: Potassium. In Nutrient Requirements of Dairy Cattle, Ed. NRC, 7th revised ed. 124–128. National Academy Press, Washington, DC.
- Ohtani F, Takusari N, Amari M, Ogasawara S, Morita S, Matsuura S, Suzuki T, Kurihara M, Higuchi K, Nonaka I 2010: Effect of low potassium diet feeding on urine volume reduction for lactating dairy cows. Bull. Natl. Inst. Livest. Grassl. Sci., 10, 1–8 (in Japanese with English summary).
- Ohyama T, Ito M, Kobayashi K et al. 1991: Analytical procedures of N, P, K contents in plant and manure materials using H2SO4-H2O2 Kjeldahl digestion method. Bull. Facul. Agric. Niigata Univ., 43, 111–120 (in Japanese with English summary).
- Olsen SR, Cole CV, Watanabe FS, Dean LA 1954: Estimation of available phosphorus in soils by extraction with sodium bicarbonate. United States Department of Agriculture Circ., 939, 1–19.
- R Core Team 2013: R: A Language and Environment for Statistical Computing, R Foundation for Statistical Computing, Vienna, Austria. http://www.R-project.org/
- Saigusa T 1996: Fertilizer application corresponding to fertility of grassland in andosols popular in eastern Hokkaido. Rep. Hokkaido Pref. Agric. Exp. Stn., 89, 1–76 (in Japanese with English summary).
- Sano Y, Kamata T, Tsukimori Y 1984: Actual conditions and problematic points of chemical properties of soils fertilized with manure of domestic animals in main dairy regions of Shimane prefecture. Bull. Shimane Prefectural Anim. Husb. Exp. Stn., 20, 41–73 (in Japanese).
- Schollenberger CJ, Simon RH 1945: Determination of exchange capacity and exchangeable bases in soil-ammonium acetate method. Soil Sci., 59, 13–24. doi:10.1097/00010694-194501000-00004
- Shapiro CA, Ferguson RB, Hergert GW, Wortmann CS, Walters DT 2008: Fertilizer suggestions for corn. EC117. University of Nebraska-Lincoln Extension, United States of Agriculture. http://ianrpubs.unl.edu/live/ec117/build/ec117.pdf (December, 2013).
- Soil Survey Staff 2014: Keys to Soil Taxonomy, 12th ed. 1–360. USDA-Natural Resources Conservation Service, Washington, DC.
- Truog E 1930: The determination of readily available phosphorus of soils. J. Am. Soc. Agron., 22, 874–882. doi:10.2134/agronj1930.00021962002200100008x
- Wakahara S, Satake Y, Murakami Y, Yamamoto M 2001: Study on chemical character contained in soil of corn fields for feed in Ehime prefecture. Bull. Ehime Anim. Husb. Exp. Stn., 18, 34–36 (in Japanese).
- Yamada H, Kamata H 1989: Agricultural technological evaluation on organic farming and gardening. I. Effects of organic farming on yields of vegetables and soil physical and chemical properties. Bull. Agric. Res. Inst. Kanagawa Pref., 131, 1–13 (in Japanese with English summary).
- Yamada I, Kubotera H 1999: Dynamics of inorganic ions in soil of the livestock farming area. Bull. Kyushu Natl. Agric. Exp. Sta., 36, 63–79 (in Japanese with English summary).
- Yamaguchi T, Harada Y, Tsuiki M 2000: Basic data of animal waste composts. Misc. Natl. Agric. Res. Cent., 41, 1–178 (in Japanese).