Abstract
The aim of this study was to evaluate the impact of land use on nitrate nitrogen (NO3-N) in shallow groundwater (G-N) and total nitrogen (N) in river water (R-N). The study area consisted of 26 watersheds (1342 km2) covering 72% of Kagawa Prefecture in Japan. We estimated G-N specific concentrations, which showed the magnitude of the upland fields, paddy fields, forests and urban land-use contributions to watershed-mean G-N. G-N specific concentrations were gained as partial regression coefficients using a multiple regression analysis of the watershed-mean G-N concentrations and the land-use ratios in each of the 26 watersheds. The results showed that the G-N specific concentration, which was gained as the partial regression coefficient for the multiple regression analysis, was 15.2 mg L−1, 10.3 mg L−1, 2.3 mg L−1 and 2.5 mg L−1 for the upland fields, paddy fields, forests and urban land-use types, respectively. R-N pollution load runoff to the river mouth was calculated by multiplying R-N specific concentration (previously reported) by river flow at the river mouth. Similarly, G-N pollution load arrival to groundwater was calculated by multiplying G-N specific concentration by the groundwater flow. The R-N pollution load runoff was 19.3 kg ha−1 y−1, 7.7 kg ha−1 y−1, 1.7 kg ha−1 y−1 and 7.6 kg ha−1 y−1, while the G-N pollution load arrival was 7.3 kg ha−1 y−1, 5.0 kg ha−1 y−1, 1.1 kg ha−1 y−1 and 1.2 kg ha−1 y−1, for upland fields, paddy fields, forests and urban areas, respectively. These results showed that the N in river water and groundwater was derived mainly from runoff and leaching from croplands. Therefore, the relationships between watershed-mean non-absorbed, applied nitrogen (NAA-N: nitrogen applied to cropland via fertilizer and manure without being absorbed by crops), R-N concentration and watershed-mean G-N concentration were investigated. A curvilinear correlation was observed between NAA-N and R-N concentrations (r2 = 0.68) except for one small, high-density, urban watershed, and a weak linear correlation was observed between NAA-N and G-N concentrations (r2 = 0.42).
INTRODUCTION
The deterioration of water quality due to increased nitrate levels is a serious problem affecting the management of water resources. Increased nitrate levels in drinking water, eutrophication of closed water bodies, and algal blooms are all major environmental issues (Cooper Citation1993; Kumazawa Citation2002; Nagumo et al. Citation2004). It is therefore important to quantify nutrient loads from both non-point sources and point sources. The increasing proportion of cropland area in drainage basins has been found to be strongly correlated with the nitrogen (N) concentration of river water (Tabuchi et al. Citation1995; Woli et al. Citation2004; Mochizuki et al. Citation2013). Woli et al. (Citation2002, Citation2004) employed regression slope, or the increase in nitrate nitrogen (NO3-N) concentration in river water (mg L−1) vs. the increase in the proportion of upland in the drainage basins (%), as “the impact factor” to assess stream water quality. They also found a correlation between impact factor and watershed-mean surplus N, except in watersheds with large point sources of N. This impact factor can be employed as an index of the land-use characteristics of a watershed. Other studies have shown that increased livestock densities in a basin were associated with an increase in river nitrate concentrations (Shimura and Tabuchi Citation1997; Cheng et al. Citation2007). Indeed, Shimura and Tabuchi (Citation1997) suggested that the risk of river water and groundwater pollution is considerable when livestock waste disposal methods are inadequate, such as when livestock waste is collected and stored in heaps in the open. However, since 1999, the risk of water pollution from the inadequate disposal of livestock waste has decreased due to promulgation of the law concerning the Appropriate Treatment and Promotion of Utilization of Livestock Manure in Japan. As a result, most livestock waste is now converted into manure and applied to agricultural fields. Essentially, this means that livestock waste has been converted from both point and non-point sources of pollution to only a non-point source of pollution that may sometimes widely affect surface water and groundwater quality. Although watershed-scale studies on nutrient discharge from non-point sources into rivers have increased in recent years, few studies have examined nutrient discharge from non-point sources into groundwater (Goss and Goorahoo Citation1995; Nakanishi et al. Citation2001).
Jordan et al. (Citation1997) and Vitousek et al. (Citation1997) found a significant correlation between surplus N and river N. Furthermore, David et al. (Citation1997) observed the quantitative relationship between the N surplus in cropland, the measured NO3-N and flux through tile-drained water, and the run-off load of NO3-N in stream water in a study on the N budget in the Embarras River basin, Illinois. The surplus N included fertilizer N (input), manure N (input), absorbed N by crop (output) and precipitation N from the air (input), irrigation N (input) and N fixation (input) and denitrification (output), among others. Although ideally all of the contributing factors were considered, the values, especially nitrogen fixation N, denitrification N and absorbed N by forests and such, were variable and difficult to quantify.
Kawashima and Toda (Citation1995) estimated the NO3-N concentration in shallow groundwater using the N budget-based model for the total area of Japanese croplands. The predicted concentration was in agreement with the measured concentration. This information suggested that the N applied artificially in fertilizer and manure to croplands without being absorbed by crops themselves could be an important contributor to the N pollution in river water and shallow groundwater.
We previously developed a simple predictive model for assessing river water quality based on the land-use ratios of watersheds (Mochizuki et al. Citation2013). The aim of this study was to evaluate the impact of land use on NO3-N in shallow groundwater (G-N) and total N in river water (R-N). We applied the model to estimate G-N concentrations, and to estimate N pollution load runoff of both river runoff and groundwater. Furthermore, we investigated the relationships between the N applied to croplands but not absorbed (non-absorbed, applied nitrogen or NAA-N), G-N and R-N concentrations.
MATERIALS AND METHODS
Study area
The study area consisted of 26 watersheds (1342 km2) covering 72% of Kagawa Prefecture, located in the middle of the Seto inland area of Japan. The mean annual temperature and precipitation are 14.4°C and 1179 mm (meteorological mesh data for 1971–2000; Japan Meteorological Agency Citation2001), respectively, and the Kagawa Prefecture has a population of 1.02 million people (Statistics Japan Citation2003). Qualitative and quantitative water conservation measures are considered important because of the relatively low levels of precipitation and the intensive agriculture. Southern Kagawa is mountainous with a sandstone foundation, while the central and northern regions are underpinned by granite and andesite which stretch east and west in the Prefecture, with alluvial plains in the north. There are approximately 30 midsize rivers running from the southern to the northern Seto Inland Sea.
Shallow groundwater exists mainly in an upper alluvial layer which consists of sand-gravel and decayed gravel in Kagawa. The upper alluvial layer commonly lies within a depth of 1 m and is between 5 and 50 m thick, with a peak at the middle part in each alluvial fan. The bottoms of some alluvial fans lie in the sea, resulting in seawater intruding into the shallow groundwater in coastal areas where pumping of groundwater is too intensive (from Wada Citation1986a). We estimated the outflow of nitrate from the double-cropped paddy fields into shallow groundwater in an alluvial fan in Kagawa by analysis of the chemical properties of spring water (shallow groundwater), irrigation-canal water and river water (Yoshikawa et al. Citation2008). We found that nitrate nitrogen was stored in soil water after the fertilizer input for the second crop, and flowed swiftly through the sand-gravel layer into the shallow groundwater mainly at the first stage of irrigation of paddy fields. The saturated water permeability of the sand-gravel layers ranged from 1–2 × 10–4m sec−1 (Kurihara Citation1986; Wada Citation1986b).
Takahashi et al. (Citation2010) estimated the movements of N, phosphorus (P) and Chemical Oxygen Demand (COD) pollution loads in Kagawa Prefecture. They found that most of the N, P and COD loads generated by industrial and domestic users flowed into the sea directly after sewage treatment. The relatively small differences in the geographical characteristics of the watersheds, combined with the absence of large point sources of pollution, mean that the watersheds in Kagawa Prefecture are well suited for evaluating non-point pollution sources in rivers and groundwater.
Land-use ratios of watersheds
The 26 watersheds were delineated using a geographic information system (GIS; Arc GIS, ESRI Ltd.; ). The GIS analysis used 50-m digital elevation data (Geospatial Information Authority of Japan Citation2001) and watershed/coastal area boundary data (National Land Numerical Information, National Land Agency Citation1977a). Because delineating groundwater watersheds is difficult, these were assumed to be the same as river watersheds based on the fact that groundwater level was reported to be almost parallel to the soil surface in the alluvial planes in Kagawa (Takakuwa Citation1965, Citation1980, Wada Citation1986b). Also, the results of analysis of a public database of geotechnical information in Japan showed that the medium groundwater levels classified by geomorphologic and geologic characteristics were within the narrow range of 1–6 m (Koshigai and Marui Citation2011).
Figure 1 Study area consisting of 26 watersheds in Kagawa Prefecture. Inland solid lines are river watershed boundaries. White-colored areas surrounding inland solid lines are watersheds. The 26 named watersheds are the study areas. Gray-colored areas are coastal areas.
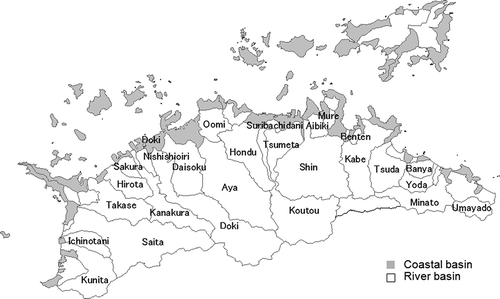
Land use in each watershed was also analyzed by GIS using cropland and forested area data (MAFF Citation2001a), as well as land-use subdivision mesh data (National Land Numerical Information, National Land Agency 1997b). Land use was divided into five categories: upland fields, paddy fields, forests, urban areas and inland waters, as shown in . The upland fields contain orchards, forests contain abandoned areas, the urban land-use category contains roads, and buildings and urban infrastructure and inland waters contain river areas, ponds and dams. Recently, the amount of abandoned agricultural land has increased due to the rice supply adjustment policy, and a decrease in the number of farmers due to aging. We therefore used the actual acreage under crop cultivation (MAFF Citation2001b) instead of registered acreage in this study.
Nitrogen concentration in shallow groundwater and rivers
The 197 G-N data measured by local governments from 2000 to 2003 on a mesh of 2 or 3 km (Kagawa Prefectural Research Institute for Environmental Sciences and Public Health 2000–2003) by sampling shallow well water were used for the analysis. The sampling depth ranged from 0.1 m to 20.0 m with an average of 5.9 m. We calculated the watershed mean G-N concentrations based on the mesh G-N concentration data, and the watershed boundary data using the GIS technique. We integrated the G-N concentration data by multiplying the ratio of the mesh area with the watershed area for all of the grids in the watersheds. R-N data which were measured once a month at each river mouth were also used (Kagawa Prefectural Research Institute for Environmental Sciences and Public Health Citation2000–2005), and the weighted-average R-N was calculated for every river and year based on continuous water-level data by Kagawa Prefecture, High-Quantity (H-Q) curve and Quantity-Total nitrogen concentration (Q-T-N) concentration curve.
Estimation of G-N specific concentration for each land-use type
We employed the model of Mochizuki et al. (Citation2013) to estimate G-N specific concentration for each land-use type. In the model, parameter ai is a specific concentration associated with land-use type i. Watershed-mean G-N concentrations were composed of the contribution of the five land-use types of upland fields, paddy fields, forests, urban areas and inland water, having respective specific concentrations. Thus, to calculate the G-N specific concentration, we used the following equation:
where C is the watershed mean G-N, ai is the G-N specific concentration for land-use type i and is showing the magnitude of i land-use contribution to watershed mean G-N, and xi is the land-use ratio for the land-use type i.
The ai were estimated as partial regression coefficients for the multiple regression analysis of the 26 watershed-mean G-N concentrations and the land-use ratios of the watersheds.
Estimation of the watershed-mean NAA-N from croplands
We focused on N discharge from areas, especially croplands, into water bodies in relation to NAA-N, which was applied to croplands via fertilizer and manure and was not absorbed by crops. Watershed mean NAA-N was estimated based on a statistical table of cropping area that had been prepared for each town for 28 main crops (MAFF 2001), application guidelines of fertilizer and manure for main crops (Kagawa Prefecture, Division for Agriculture and Fishery Administration Citation2003) and data about N uptake by crops (Nishio Citation2001). The N concentration of manure was assumed to be 1.16% based on the share of various kinds of manure in Kagawa Prefecture (Mishima and Kohyama Citation2010) and their average N concentrations (Fujiwara Citation2008).
The annual mean R-N concentrations at the river mouths and watershed mean G-N concentrations were analyzed in relation to the watershed mean NAA-N by single regression analysis.
Estimation of R-N pollution load runoff and G-N pollution load arrival for each land-use type
Pollution loads, which are used to estimate the magnitude of water pollution, were defined as follows: pollution load generation, pollution load discharge (pollution load generation × discharge ratio), pollution load arrival (pollution load discharge × arrival ratio) and pollution load runoff [pollution load arrival × (1 – purification ratio)]. Pollution load arrival is defined as the pollution load that enters water bodies, such as rivers and groundwater aquifers. Pollution load runoff is defined as pollution loads that are measured at monitoring stations after flowing in rivers or in groundwater. The N purification ratio is thought to be small in urban-area watersheds with short river lengths because N purification by denitrification and trapping by plants and animals cannot be completely achieved.
R-N pollution load runoff (to the monitoring station) was estimated by multiplying the R-N concentration and river water flow rate. Similarly, G-N pollution load arrival (to groundwater) was estimated by multiplying the G-N concentration and groundwater flow rate.
For Kagawa Prefecture, the average river flow rate was estimated to be 2134 t ha−1 y−1 (Takahashi et al. Citation2010), and the average groundwater flow rate was estimated to be 481 t ha−1 y−1 (Shimizu et al. Citation2009). R-N pollution load runoff and G-N pollution load arrival are calculated using these flow rates for each land-use type, and then discussed.
RESULTS AND DISCUSSION
Relationship between land use and water quality
The G-N concentration mesh data are shown in a. From the mesh data, watershed-mean G-N concentrations were calculated as shown in b. The land-use ratios and 6-year annual mean R-N concentrations, and watershed mean G-N concentrations, are shown in . The watershed mean G-N concentrations were used to analyze the relationship between watershed land use and groundwater quality, but not the G-N concentrations at the ends of the watersheds. The reason for this is that seawater intrusion into the shallow groundwater found in some watersheds might result in inadequate analysis, and the mesh sizes for the survey of G-N concentrations were too coarse to identify the end of the watersheds.
Table 1 Land-use ratio, total nitrogen concentration in river water (R-N) and nitrate nitrogen concentrations in shallow groundwater (G-N) for 26 watersheds.
Figure 3 Nitrate nitrogen (NO3-N) concentrations in shallow groundwater in Kagawa. (a) Mesh data of nitrate nitrogen (NO3-N) concentrations in shallow groundwater. (b) Watershed-mean nitrate nitrogen (NO3-N) concentrations in shallow groundwater.
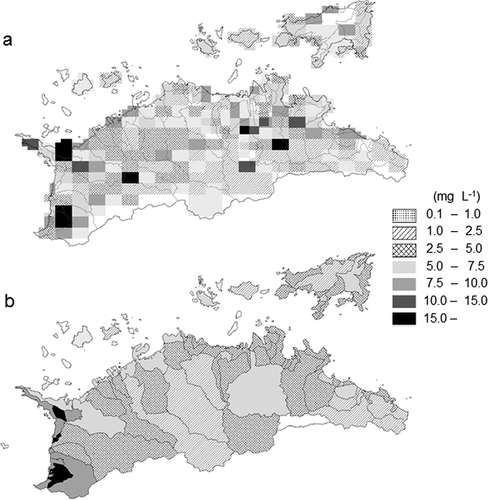
The areas of the 26 watersheds ranged from 4.4 to 137.8 km2, and the land-use ratios ranged from 0.3–10.0%, 0.4–33.5%, 0.0–92.8%, 0.0–63.4% and 1.5–16.7% for upland fields, paddy fields, forests, urban areas and inland waters, respectively.
The relationship between these land-use ratios and the watershed mean G-N concentrations is shown in . The relationship between G-N concentrations and land-use ratios was similar to that between R-N concentrations and land-use ratios (Mochizuki et al. Citation2013). The watershed mean G-N concentrations were positively correlated with the ratios for upland fields, paddy fields and urban areas, and negatively correlated with the ratio for forests. However, the correlation coefficients were smaller than those obtained for the relationships between R-N concentrations and land-use ratios.
Figure 4 Correlation between land-use ratios of watersheds and annual-mean total nitrogen concentrations in river water (R-N) and the watershed-mean nitrate nitrogen concentrations in shallow groundwater (G-N).
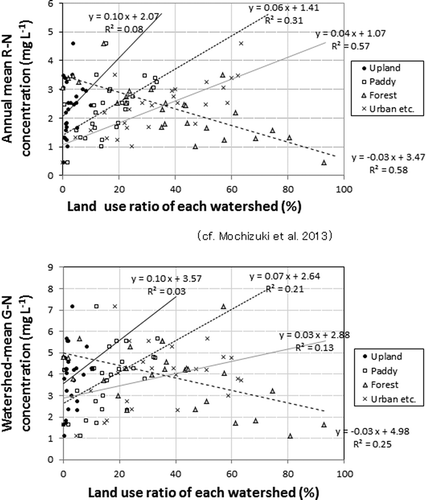
shows the relationship between cropland (upland fields + paddy fields) ratios and R-N concentrations. R-N concentrations were linearly correlated (r2 = 0.40) with cropland ratios, and the slope of the correlation was 0.065. Woli et al. (Citation2004) defined the slope of the correlation line between land-use ratio and N concentration in river water as the “impact factor.” They found that the impact factor was 0.04 for a watershed used for intensive livestock farming (livestock watershed), 0.02–0.03 for an upland field-livestock watershed and 0.005–0.015 for a paddy field–upland field watershed. The watersheds in Kagawa Prefecture are considered to be of the paddy field–upland field type. The impact factor of 0.065 gained here was several times larger than the values obtained by Woli et al. (Citation2004). The difference was considered to be due to the low rainfall in Kagawa Prefecture, similar to the findings of Hojito et al. (Citation2003), and shorter river lengths than those reported by Woli et al .(Citation2004) in their study areas.
Specific G-N concentration for each land-use type
The specific concentration, ai, for G-N was determined as a partial regression coefficient in the multiple regression analysis, and is summarized in . Here, NO3-N was substituted for T-N in groundwater because almost the entire N in groundwater is in the form of NO3-N (Yoshikawa et al. Citation2008). The land-use type “inland water” was excluded from the analysis because it was found to have no significant recognizable effect on the G-N concentration, and no validity was found in the 5th term of Eq. 1. This suggests that inland water may have both the effect of purification by denitrification and that of pollution by promoted N infiltration.
Table 2 Specific concentrations (ai) for nitrogen nitrate in shallow groundwater (G-N) and total nitrogen in river water (R-N) from particular land-use types. (*5% level significant, **1% level significant).
The ai values for G-N were 15.2, 10.3, 2.3 and 2.5 mg L−1 for upland fields, paddy fields, forests and urban land-use types, respectively. The ai values for R-N determined in the previous paper (Mochizuki et al. Citation2013) were 9.1, 3.6, 0.8 and 3.6 mg L−1 for upland fields, paddy fields, forests and urban land-use types, respectively as shown in . The values of specific G-N concentrations and their order were similar to those of specific R-N concentrations. However, the specific G-N concentrations for the paddy fields and forest land-use types were relatively larger than the specific R-N concentrations, except in the urban category.
Estimation of R-N pollution load runoff and G-N pollution load arrival for each land-use type
The R-N pollution load runoff and G-N pollution load arrival were estimated as shown in . The R-N pollution load runoff for the different land-use types was 19.3 kg ha−1 y−1 for upland fields, 7.7 kg ha−1 y−1 for paddy fields, 1.7 kg ha−1 y−1 for forests and 7.6 kg ha−1 y−1 for urban areas. Similarly, the G-N pollution load arrival for the different land-use types was 7.3 kg ha−1 y−1 for upland fields, 5.0 kg ha−1 y−1 for paddy fields, 1.1 kg ha−1 y−1 for forests and 1.2 kg ha−1 y−1 for urban areas. Total R-N pollution load runoff and G-N pollution load arrival for the different land-use types was 26.6 kg ha−1 y−1 for upland fields, 12.6 kg ha−1 y−1 for paddy fields, 2.8 kg ha−1 y−1 for forests and 8.8 kg ha−1 y−1 for urban areas, for reference, despite the ambiguity of definition of pollution loads.
Table 3 Nitrogen pollution loads estimated from specific concentration and flow rate. N: nitrogen; R-N: total nitrogen in river water; G-N: nitrate nitrogen in shallow groundwater.
The N pollution load discharge values adopted by the Ministry of Environment (MOE; JSWA Citation2008), which are the standard used in Kagawa Prefecture, are shown in . The values were 27.7 kg ha−1 y−1 for the upland fields and paddy fields, and 6.9 kg ha−1 y−1for the urban areas and forest land-use types. The standard pollution load discharge was set for planning a sewerage system based on the results of several experiments. However, the level of detail of these experiments would not be possible nowadays (JSWE Citation2012). JSWE (Citation2012) reviewed many reports of non-point pollution loads by each land use to revise them. They found that pollution load discharge from each land use ranged widely but that average values were higher than those by MOE, as shown in .
In this study, the R-N pollution load runoff from particular land-use types was estimated from R-N concentrations measured at the monitoring stations around river mouths.
The N pollution load runoff is considered to be smaller than the N pollution load discharged from particular land-use types due to a decrease in N attributed to denitrification, trapping by plants and animals, and water abstraction from rivers while flowing down (Hill Citation1996; Nagumo and Hatano Citation2000). In this study, urban pollution loads contain inaccuracies for both R-N and G-N because pollution loads from point sources could not be excluded completely, even if most of the pollution loads generated by industrial and domestic users flowed into seawater directly after sewage treatment (Takahashi et al. Citation2010).
Furthermore, the R-N and G-N pollution loads were differently defined, i.e., the former was pollution load runoff and the latter was pollution load arrival. Therefore, the values obtained in this study could not be compared directly with the average data generated by Japan Society on Water Environment (Citation2012). However, the relative trends were similar—that is, the pollution loads decreased in the order of upland, paddy fields, urban and forest.
Relations between NAA-N and R-N or G-N concentrations
Because a high contribution of cropland to N pollution in river water and groundwater was seen in , we investigated the relationship between NAA-N and R-N or G-N. shows the watershed mean NAA-N and the amount of absorbed N for 28 main crops in Kagawa Prefecture. More than half of the N input into cropland was not absorbed by crops. NAA-N tended to be large for vegetables that require large quantities of manure to ensure quality.
Figure 6 Estimated absorbed nitrogen (N) and non-absorbed, applied nitrogen (NAA-N) for various crops in Kagawa Prefecture.
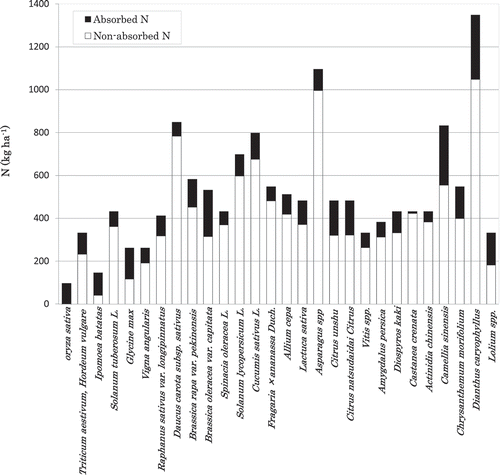
We plotted the relationship between watershed mean NAA-N and the annual weighted average R-N in Kagawa Prefecture (). Except for one small, high-density, urban watershed, a curvilinear correlation was observed between NAA-N and R-N (r2 = 0.68).
Figure 7 Relationship between watershed-mean non-absorbed, applied nitrogen (NAA-N) and annual-mean total nitrogen in river water (R-N) or watershed-mean nitrate nitrogen concentrations in shallow groundwater (G-N). (a) Correlation between watershed-mean NAA-N and annual-mean R-N. ◇ is the smallest watershed located mainly in the crowded urban land-use type. (P < 0.01 level significant except ◇). (b) Correlation between watershed-mean NAA-N and watershed-mean G-N. (P < 0.01 level significant).
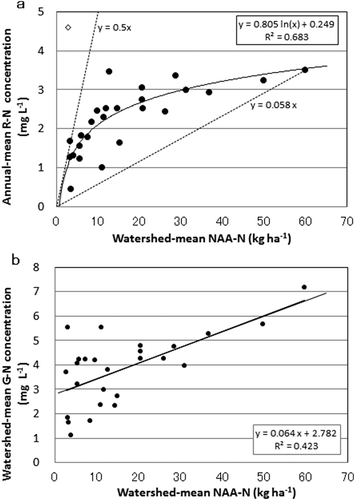
We also plotted the relationship between watershed-mean NAA-N and G-N in Kagawa Prefecture (). A weak linear correlation was observed between NAA-N and G-N (r2 = 0.42).
The plateau effect of the mean R-N concentration value seen with increasing NAA-N was considered to be due to denitrification, which appeared to occur easily in rivers (Hill Citation1996; Nagumo and Hatano Citation2000). N-rich river water tends to contain amounts of organic matter and lack oxygen around the river bottom due to the decomposition of organic matter, which leads to denitrification of R-N. However, groundwater commonly contains little organic matter due to the filtering mechanism of soil particles and the smaller population of microorganisms in the subsoil than surface soil. These conditions might suppress denitrification of G-N.
A trial calculation was conducted under a very rough hypothesis that R-N and G-N come from only NAA-N. R-N runoff to river mouths was calculated to be 2.10 kg ha−1 at NAA-N of 10 kg ha−1 (20.1%), 6.37 kg ha−1 at NAA-N of 30 kg ha−1, (21.2%) and 7.43 kg ha−1 at NAA-N of 60 kg ha−1 (12.4%). Similarly, G-N arrival to the shallow groundwater was calculated to be 1.65 kg ha−1 at NAA-N of 10 kg ha−1 (16.5%), 2.26 kg ha−1 at NAA-N of 30 kg ha−1 (7.5%) and 3.19 kg ha−1 at NAA-N of 60 kg ha−1 (5.3%). The above values are overestimates of the contribution of N from croplands to R-N and G-N. However, in this study, the high contribution of N from croplands on river and shallow groundwater quality was clarified by estimating specific concentrations and pollution loads for each land-use category. The results showed that increased N-use efficiency by crops is necessary to mitigate water pollution in river runoff and groundwater.
In this and the previous paper (Mochizuki et al. Citation2013), we propose a simple method to determine the specific concentrations for different land-use types. Using specific concentrations, R-N concentration and watershed-mean shallow G-N concentration could be roughly estimated under some scenarios with land-use change. The model has a simple framework and does not need special data. However, the model proposed here needs at least the same number of watersheds as the number of land-use types, and its applicability could be improved in cases where the loads from point sources are not large, river lengths are not too different and geographical features are similar between watersheds. When the application guidelines of fertilizer and manure are changed and farmers follow the guidelines, the specific concentration for land use should be changed. Even in such cases, R-N and G-N concentrations can be estimated roughly from the relationship between NAA-N and R-N/G-N concentrations.
CONCLUSIONS
The aim of this study was to evaluate the impact of land use on NO3-N in shallow groundwater (G-N) and total-N in river water (R-N). Both watershed mean G-N and annual mean R-N concentrations tended to increase with the ratios of upland fields, paddy fields and urban areas, but decreased with the ratios of forests in the 26 river watersheds.
The G-N and R-N specific concentrations for each land-use type showed the magnitude of the land-use contributions to G-N and R-N, and were calculated as partial regression coefficients for the multiple regression analysis of G-N or R-N concentrations and the land-use ratios. Furthermore, G-N pollution load arrival to the shallow groundwater and R-N pollution load runoffs to the river mouths were identified by multiplying G-N or R-N specific concentrations by groundwater flow rate or river flow rate.
These results showed that R-N and G-N were derived mainly from runoff and leaching from croplands. Therefore, the R-N or watershed mean G-N concentrations were positively correlated with the watershed mean non-absorbed applied nitrogen (NAA-N). These procedures were useful to roughly estimate R-N and G-N concentrations from land-use ratios or NAA-N under some scenarios with changed land use or altered guidelines for application of fertilizer and manure.
ACKNOWLEDGMENTS
The authors thank Mr. Y. Kanno of the Kagawa Prefectural Research Institute for Environmental Sciences and Public Health, H. Takano of the Okayama Prefectural Research Center for Environmental Sciences and Public Health, and Dr. S. Onodera and his staff at Hiroshima University for their helpful discussions. The authors would also like to thank Dr. Y. Koshino, the former Division Director of National Institute for Agro-Environmental Sciences, and Dr. O. Matsuda of the Research Institute for the Seto Inland Sea for their comprehensive assistance and feedback. This study was financially supported by the Research Fund for Practical Technique Development (No.1947) from the Ministry of Agriculture, Forestry and Fisheries.
REFERENCES
- Cheng H, Ouyang W, Hao F, Ren X, Yang S 2007: The non-point source pollution in livestock-breeding areas of the Heihe River basin in Yellow River. Stoch Environ. Res. Risk Assess, 21, 213–221. doi:10.1007/s00477-006-0057-2
- Cooper CM 1993: Biological effects of agriculturally derived surface water pollutants on aquatic systems-a review. J. Environ. Qual., 22, 402–408. doi:10.1080/00380768.2000.10408788
- David MB, Gentry LE, Kovacic DA, Smith KM 1997: Nitrogen balance in and export form an agricultural watershed. J. Environ. Qual., 26, 1038–1048. doi:10.2134/jeq1997.00472425002600040015x
- Fujiwara S 2008: Composting Materials, Hiryo Binran (Fertilizer Handbook), pp. 218–223. Nobunkyo, Tokyo (in Japanese).
- Geospatial Information Authority of Japan 2001: Digital MAP 25000. Japan Map Center, Tokyo (Map Image).
- Goss MJ, Goorahoo D 1995: Nitrate contamination of groundwater: measurement and prediction. Fert. Res., 42, 331–338. doi:10.1007/BF00750525
- Hill AR 1996: Nitrate removal in stream riparian zones. J. Environ. Qual., 25, 743–755. doi:10.2134/jeq1996.00472425002500040014x
- Hojito M, Ikeguchi A, Kohyama K, Shimada K, Ogino A, Mishima S, Kaku K 2003: Estimation of nitrogen loading in Japanese Prefectures and scenario testing of abatement strategies. Jpn. J. Soil Sci. Plant Nutr., 74, 467–474 (in Japanese with English summary).
- Japan Sewage Works Association (JSWA) (Design conference on sewage construction for watershed scale) 2008: Guideline for Integrated Sewage Construction for Watershed Scale, pp. 43–68. Japan sewage works association, Tokyo (in Japanese).
- Japan Society on Water Environment (JSWE) 2012: Study on Load Estimation of Non-Point Source Pollution, RFb-11T1, P1-54 Takamatsu, Kagawa (Principal Investigator: Furumi H). http://www.hokosha.jp/beta/hitokutei/seika.html (under construction at time of publication).
- Jordan TE, Correll DL, Weller DE 1997: Effect of agriculture on discharges of nutrients from coastal plain watersheds of Chesapeake Bay. J. Environ. Qual., 26, 836–848. doi:10.2134/jeq1997.00472425002600030034x
- Kagawa Prefecture, Division for Agriculture and Fishery Administration 2003: Sehi Sekkei Shishin (Guidelines of Application of Fertilizer and Manure). Division for Agriculture and Fishery Administration, Kagawa Prefecture (in Japanese).
- Kagawa Prefectural Research Institute for Environmental Sciences and Public Health 2000–2003, 2000–2005: Suishitsu Sokutei Kekka (Results of Water Quality Measurement). Kagawa Prefectural Research Institute for Environmental Sciences and Public Health, Kagawa (in Japanese).
- Kawashima S, Toda H 1995: Prediction of nitrate concentration in underground water around field. J. Appl, Syst. Stud., 11, 175–181 (in Japanese with English summary).
- Koshigai M, Marui A 2011: Estimation of water table based on geomorphologic and geologic conditions using public database of geotechnical information over Japan. J. Groundwater Hydrol., 53, 179–191. doi:10.5917/jagh.53.179 (in Japanese with English summary).
- Kumazawa K 2002: Nitrogen fertilization and nitrate pollution in groundwater in Japan: present status and measures for sustainable agriculture. Nutr. Cycl. Agroecosyst., 63, 129–137. doi:10.1023/A:1021198721003
- Kurihara G 1986: Chapter 8 Groundwater in Shikoku Section 2 Groundwater of the Regions-1, pp. 652–656. Takamatsu Plane, Editorial Committee for “ Groundwater in Japan”, Chikyu-sya, Tokyo (in Japanese).
- MAFF (Ministry of Agriculture, Forestry and Fisheries) 2001a: Report on results of 2000 world census of agriculture and faculty in Japan. MAFF, Tokyo.
- MAFF (Ministry of Agriculture, Forestry and Fisheries) 2001b: Japanese Food Information. Kagawa Prefecture (Kagawa Norin Suisan Tokei Nenpo).
- Meteorological Agency 2001: Meteorological data reference. http://www.data.jma.go.jp/obd/stats/etrn/index.php
- Mishima S, Kohyama K 2010: The database and the methodologies to estimate recent trend of nitrogen(N) and phosphate (P) flows and residual N and P in Japanese national prefectural scales and examples their application. Bull. Natl. Inst. Agro-Environ. Sci., 27, 117–139 (in Japanese with English summary).
- Mochizuki H, Takahashi H, Yoshikawa S 2013: Development of prediction model for water quality in watersheds based on proportion of area by land use. People Environ., 39(3), 2–8 (in Japanese with English summary).
- Nagumo T, Hatano R 2000: Impact of nitrogen cycling associated with production and consumption of food on nitrogen pollution of stream water. Soil Sci. Plant Nutr., 46, 325–342. doi:10.1080/00380768.2000.10408788
- Nagumo T, Woli KP, Hatano R 2004: Evaluating contributions of point and non-point sources of nitrogen pollution in stream water in a rural area of central Hokkaido. Soil Sci. Plant Nutr., 50, 109–117. doi:10.1080/00380768.2004.10408458
- Nakanishi Y, Takahira K, Shimoji K 2001: Estimation of nitrogen loading factors for groundwater by multiple regression analysis. Jpn. J. Soil Sci. Plant Nutr., 72, 365–371 (in Japanese with English summary).
- National Land Numerical Information, National Land Agency 1977a: watershed/coastal area boundary data. Japan Map Center, Tokyo.
- National Land Numerical Information, National Land Agency 1977b: Land-use subdivision mesh data. Japan Map Center, Tokyo.
- Nishio M 2001: A method to assess the risk of nitrate pollution of groundwater by the nitrogen fertilization load from the individual crop species. Jpn. J. Soil Sci. Plant Nutr., 72, 522–528 (in Japanese with English summary).
- Shimizu Y, Onodera S, Saito M 2009: Estimation of spacial variation in submarine groundwater discharges from a coastal grid 50m square, using a topographic model: an example of the application to the middle of the Seto Inland Sea. Jpn. J. Limnol., 70, 129–139. doi:10.3739/rikusui.70.129 (in Japanese with English summary).
- Shimura M, Tabuchi T 1997: The relation between cattle farming and nitrogen concentration in stream waters: research on nitrogen outflow from high stocking density area (Ⅳ). Trans. Jpn. Soc. Irrig. Drain. Reclam. Eng., 189, 375–3804. doi:10.11408/jsidre1965.1997.375 (in Japanese with English summary).
- Statistics Japan 2003: Portal Site of Official Statistics of Japan. National Statistics Center, Tokyo. http://www.e-stat.go.jp/SG1/estat/GL08020102.do?_toGL08020102_&tclassID=000001028704&cycleCode=7&requestSender=estat
- Tabuchi T, Yoshino K, Shimura M, Kuroda S, Ishikawa M, Yamaji E 1995: Relation between land use and nitrate concentration of outflow water from watersheds of agricultural and forest area. Trans. Jpn. Soc. Irrig. Drain. Reclam. Eng., 178, 129–135 (in Japanese with English Summary).
- Takahashi H, Yoshikawa S, Takano H, Sasada Y, Ninomiya S 2010: Estimation of pollution load from the Okayama and Kagawa basins flowing into the Seto Inland Sea in light of their basin characteristics. Jpn. J. Limnol., 71, 269–284. doi:10.3739/rikusui.71.269 (in Japanese with English summary).
- Takakuwa T 1965: Chapter 7 Unconfined Ground Water, Section 1 Unconfined Ground Water in Takamatsu Plane, pp. 193–212. Division of Geography, Tokyo Educational University, Aoba-bunkan, Tokyo (in Japanese).
- Takakuwa T 1980: Study on presence of groundwater in Sakaide/Marugame area. Edu. Dept. Bull. Kagawa Univ., 1(50), 83–123 (in Japanese).
- Vitousek PM, Aber JD, Howarth RW, Likens GD, Matson PA, Schindler DW, Schlesinger WH, Tilman DG 1997: Human alteration of the global nitrogen cycle: source and consequences. Ecol. Appl., 7, 737–750.
- Wada M 1986a: Chapter 8 Groundwater in Shikoku Section 1 Outline of Groundwater in Shikoku, pp. 645–652. Editorial Committee for “Groundwater in Japan”, Chikyu-sya, Tokyo (in Japanese).
- Wada M 1986b: Chapter 8 Groundwater in Shikoku Section 2 Groundwater in Regions-2.Marugame Plane, pp. 656–658. Editorial Committee for “ Groundwater in Japan”, Chikyu-sya, Tokyo (in Japanese).
- Woli KP, Nagumo T, Hatano R 2002: Evaluating impact of land use and N budgets on stream water quality in Hokkaido. Jpn. Nutr. Cycl. Agroecosyst., 63, 175–184. doi:10.1023/A:1021146707412
- Woli KP, Nagumo T, Kuramochi K, Hatano R 2004: Evaluating river water quality through land use analysis and N budget approaches in livestock farming areas. Sci.Total Environ., 329, 61–74. doi:10.1016/j.scitotenv.2004.03.006
- Yoshikawa S, Yamamoto H, Yoshida M, Ishihara A 2008: Variation of nitrate concentration of spring water in double-cropped paddy fields in Marugame plain. Jpn. J. Soil Sci. Plant Nutr., 79, 461–470 (in Japanese with English summary).