ABSTRACT
Silicon (Si) and arsenite (iAsIII) share the same pathway of uptake and translocation in rice (Oryza sativa L.), and it has been reported that Si can decrease arsenic (As) uptake by rice in hydroponic culture. Due to the competitive sorption between Si and As for the soil minerals, different extents of effects on As toxicity and uptake by rice as influenced by soil Si application were found. In order to avoid the competitive sorption between Si and As for the soil minerals, foliar applications of Si might be a more efficient alternative to reduce As uptake by rice. Therefore, the aim of this study was to investigate the effects of Si foliar and soil applications on the growth and As accumulation in rice seedlings. In this study, three application rates of Si (as sodium silicate) were applied to (1) the foliage and (2) the soil, of rice grown in two soils with different Si retention capacities and As contents. The results showed that there were no significant differences in Si concentrations in shoots among different Si foliar applications, and it had no significant effect on the growth and As accumulation in rice seedlings. In contrast, soil applications of Si caused a decrease in As accumulation in shoots grown in the tested soils, resulting from a high Si/As ratio in the soil pore water allowing enough Si to out-compete the As for uptake by roots. These results were further supported by the significant negative correlation found between Si and As in the shoots. However, a growth inhibition of rice plants was also observed due to As toxicity from the addition of excessive treatments of Si into As-contaminated soils. Therefore, the results of this study suggest that foliar applications of Si are not able to decrease As accumulation in rice seedlings, but that the application of moderate amounts of Si into As-contaminated soils could effectively reduce As uptake by rice seedlings.
1. Introduction
Arsenic (As) is widely distributed in the environment and is a well-known human carcinogenic metalloid. Human intake of As through the food chain may lead to serious cancer risks, especially in some rice (Oryza sativa L.)-producing regions of the world (Tchounwou et al. Citation2004; Mondal and Polya Citation2008). In recent years, many reports have indicated that rice consumption is the main human exposure route to As, because of the high bioavailability and mobility of As in flooding paddy soils (Williams et al. Citation2007a; Mondal and Polya Citation2008), leading to the uptake and accumulation of As by rice plants (Xu et al. Citation2008). Paddy rice is a dietary staple in Asia and, unfortunately, the accumulation of As in rice grains is about 10-fold higher than in other cereals (Williams et al. Citation2007a, Citation2007b; Su et al. Citation2010). Moreover, paddy rice grown in high As content soils results in As phytotoxicity, which in turn results in a decrease of plant growth and grain yields (Panaullah et al. Citation2009; Syu et al. Citation2015). Therefore, the reduction of As mobility and bioavailability in soils and As uptake by rice has become an important issue.
In general, the As species existing in soil solution is strongly controlled by the redox status of the soil (Meharg and Rahman Citation2003), and the amounts of inorganic As species are higher than those of methylated As species in paddy soils. Inorganic arsenite (iAsIII; as Arsenous acid, H3AsO3) is the predominant species under anaerobic conditions, and may be primarily responsible for As phytotoxicity and uptake by rice plants (Takahashi et al. Citation2004). Previous studies have demonstrated that iAsIII is taken up into the rice roots via aquaporin channels, thus sharing the silicic acid (H4SiO4) transport pathway. This is explained by arsenite and silicic acid having similar chemical properties, such as high pKa1 (pH 9.0–9.3) and molecular structures and sizes (Ma et al. Citation2008; Guo et al. Citation2009; Zhao et al. Citation2010). There are two silicic acid transporters, Lsi 1 and Lsi 2, located at the distal and proximal sides of the exodermis and endodermis plasma membranes in rice roots, respectively. The influx transporter Lsi 1 is involved in iAsIII uptake into rice roots from the rhizosphere, and the efflux transporter Lsi 2 is responsible for the iAsIII transport to the xylem and As accumulation in the shoot tissues of rice (Ma et al. Citation2008).
Silicon (Si) is a beneficial element for paddy rice, and the contents of Si in rice plants are up to 10% in dry weight (Takahashi Citation1995; Ma et al. Citation2006). It has been reported that Si can enhance the resistance of plants to biological and non-biological stresses (Ma Citation2004; Guntzer et al. Citation2012). Sun et al. (Citation2010) showed that Si can protect rice from diseases by physically strengthening cell walls. In addition, Si is known to decrease the toxicity levels in rice from several metals, e.g., aluminum (Al), cadmium (Cd), manganese (Mn) and zinc (Zn) (Rogalla and Romheld Citation2002; Shi et al. Citation2005; Liang et al. Citation2007; Song et al. Citation2011). Due to the competitive effect between Si and As for uptake and translocation in rice plants (Ma et al. Citation2008), many studies have shown that an Si supply could alleviate As phytotoxicity and reduce As accumulation in paddy rice grown in hydroponic (Guo et al. Citation2005, Citation2009; Tripathi et al. Citation2013) and pot experiments (Bogdan and Schenk Citation2008; Li et al. Citation2009; Seyfferth and Fendorf Citation2012; Fleck et al. Citation2013). For example, Li et al. (Citation2009) and Fleck et al. (Citation2013) indicated that the As concentrations in rice grains were reduced by 16 and 22%, respectively. In the study of Bogdan and Schenk (Citation2008), a negative relationship between Si concentration in soil solutions and As accumulation by rice grown in different soils was reported. However, Lee et al. (Citation2014) observed an increase in As release and phytotoxicity of rice seedlings grown in seriously As-contaminated paddy soils (190–240 mg As kg−1) after Si fertilizer application, which was due to an increase in As concentration in soil solution caused by competitive adsorption between Si and As. This last result suggests that the As accumulation in rice plants is mainly controlled by the Si/As molar ratio in soil solutions.
Due to the similarity in behavior of Si and iAsIII, they may compete against each other for adsorption sites on the surface of soil minerals. This can result in a decrease in inhibition of As uptake by rice after Si application into soils. To avoid this undesirable effect of soil applications, a foliar application of Si might be a more efficient alternative to reduce As accumulation and alleviate As toxicity in rice. Some previous studies have indicated that a foliar application of Si can alleviate Cd toxicity and accumulation in rice grains grown in Cd-contaminated soils (Liu et al. Citation2009; Wang et al. Citation2015). However, there are an insufficient number of studies to understand the effect of foliar application of Si fertilizers on As accumulation in rice plants. Therefore, the aims of this study are to investigate the effects of soil and foliar sodium silicate applications on the As toxicity and Si and As accumulation in rice seedlings grown in soils with different properties and As contents. In addition, the adverse effects of Si soil application on the growth and As accumulation of rice were reported from our previous studies. Therefore, we intend to clarify the possible control factors in this study.
2. Materials and methods
2.1 Soil collection and characterization
Two paddy soils from different soil series were used in this study. Two Guandu (Gd) topsoils (0–20 cm) that differed in As levels were collected from paddy fields in the Guandu Plain, Taipei, Taiwan (17 and 129 mg As kg−1, expressed as Gd-low (Gd-L) and Gd-high (Gd-H), respectively). In the Guandu Plain, there are about 128 ha of paddy soils that have been identified as As-contaminated areas, which result from irrigation with As-contaminated spring water (Chiang et al. Citation2010). Uncontaminated paddy soil was collected from the topsoil of a Chiwulan (Ca) soil series, located in Yilan, Taiwan.
The soil samples were air-dried, passed through a 10-mesh sieve and stored in plastic vessels at ambient temperature. The analyses of basic soil properties were as follows: soil pH was measured for a 1:1 ratio of soil to water (McLean Citation1982), texture was measured using the hydrometer method (Gee and Bauder Citation1986), crystalline and amorphous iron (Fe) contents were extracted by dithionite–citrate–bicarbonate (DCB) and ammonium oxalate (Mehra and Jackson Citation1960; Mckeague and Day Citation1966), organic carbon was determined by the Walkley–Black wet digestion method (Nelson and Sommers Citation1982), the available Si in soils was extracted by sodium acetate (Xu et al. Citation2001), and the total As in soil was determined by the nitric acid-hydrogen peroxide (HNO3-H2O2) digestion method (Meharg and Rahman Citation2003). A certified reference material of soil (RTC CRM 025–250) was used to verify the recovery for soil As analysis.
2.2 As and Si treatments
A portion of uncontaminated Ca soils was spiked with disodium hydrogen arsenate (Na2HAsO4 · 7H2O, ACS reagent, Sigma) solution, and the As-spiked soils (Ca−H) were then subjected to the cycling process of wetting (to saturation) and drying, followed by further sieving and mixing (21 d for each cycle). Three cycling processes were done at room temperature for approximately 63 d of aging time. Subsamples of As-spiked Ca soil were taken and total As contents were determined. The As concentrations of Ca−L and Ca−H were 16 and 114 mg As kg−1, respectively (). Accordingly, two As-uncontaminated/un-spiked soils (Gd−L and Ca−L) and As-contaminated/spiked soils (Gd−H and Ca−H) of Gd and Ca soil series were used in this study.
Table 1. The basic properties of the studied soils.
In this study, the Si treatments included soil and foliar applications. For soil applications, the amount of Si application was based on the Si fertilizer recommendation rate for rice cultivation from the Council of Agriculture, Taiwan. The recommended application rate was 0.1753 g Si kg−1 (about 0.75 ton silicon dioxide (SiO2) ha−1). We conducted different Si treatments by adding sodium silicate solutions (Na2O(SiO2)x · xH2O, Sigma Aldrich, reagent grade) into soils at rates of 0-, 1- and 3-fold the recommended rate (expressed as S-control, S + Si and S + 3Si). The contents of sodium ion (Na+) in soils were adjusted to equivalent levels by adding sodium chloride (NaCl) solutions to compensate for the differences resulting from Si application or As-spiking. For foliar application, 50 mL sodium silicate solutions (0, 0.1 and 0.3% Si) were sprayed on the leaves of rice seedlings (expressed as F-control, F + Si and F + 3Si). The control treatments were sprayed with the same quantity of distilled water. In order to avoid the dripping of sprayed sodium silicate solution into soils, the soil surface was covered with aluminum foil during the foliar application of Si solution. Soil and foliar Si treatments were applied on the 15th (seedling stage) and 30th (early tillering stage) days after transplantation.
2.3 Pot experiments of rice seedlings
Pot experiments were performed in the phytotron glasshouse under controlled conditions (day 25/night 20°C; relative humidity: 70–95%) and natural light. The Taikeng 9 cultivar of paddy rice (Oryza sativa L.) was used in this study because of its high quality and common cultivation in Taiwan. Rice seeds were sterilized in a solution containing 1% sodium hypochlorite (NaOCl) and one drop of Tween 20 for 30 min, washed with distilled water and then germinated in petri dishes containing moist tissue paper placed in a 37°C incubator for 2 d. After germination, rice seedlings were transferred to an As-uncontaminated soil for 12 d. Five seedlings were transplanted into each pot filled with 500 g of tested soils and grown for 50 days. On the 15th and 30th days after transplantation, sodium silicate solutions were added through soil and foliar applications as mentioned above. The water level was maintained at about 3–5 cm above the soil surface during the whole period of plant growth. The soil was supplemented with urea [CO(NH2)2], monocalcium phosphate [Ca(H2PO4)2 · H2O] and potassium chloride (KCl) at the recommended rates of 110 kg nitrogen (N) ha−1, 100 kg phosphorus oxide (P2O5) ha−1 and 180 kg potassium oxide (K2O) ha−1 as basal fertilizers, and the application of 110 kg N ha−1as a top dressing on the 15th day after transplantation. After harvesting, the rice seedlings were rinsed with tap water and then with distilled water. The roots and shoots of these samples were then separated and the biomass of rice plants and lengths of each shoot and root were measured.
2.4 Soil pore water collection and analysis
Soil pore water was collected by soil moisture samplers (Rhizosphere Research Products) inserted into the soil near the middle of the pot during the plant growth period, and the collected solutions were filtered through a 0.45-μm filter. To avoid co-precipitation of As and Fe ions and the transformation of As species, a portion of the solution was taken and preserved immediately in 5% nitric acid (HNO3) and 0.01 M phosphoric acid (H3PO4) (Daus et al. Citation2006), respectively. Moreover, in order to prevent precipitation of Si after the acidification process, another portion of the solution was diluted with deionized water and all analyses were completed within 24 h. The concentrations of Si and As (As species) were determined by inductively coupled plasma-optical emission spectrometry (ICP-OES, Optima 8000, Perkin Elmer) and high-pressure liquid chromatography-inductively coupled plasma-mass spectrometry (HPLC-ICP-MS, LC 1200 and ICP-MS 7700x, Agilent Technologies), respectively. The details of As species analysis including As species standards, column, mobile phase and HPLC conditions were the same as those described in our previous research (Syu et al. Citation2015). The pH and redox potential (Eh) in soil during flooding conditions were measured with a glass electrode and platinum electrode, respectively.
2.5 Plant digestion and analysis
The roots and shoots of rice seedlings were oven dried at 70°C for 48 h, and ground to a fine powder. Dried plant tissues (0.1–0.2 g) were digested in HNO3/H2O2 in heating blocks (Meharg and Rahman Citation2003), and the digests diluted to 50 mL with deionized water; As concentrations in digests were determined by ICP-MS. Certified reference materials of the plant sample (NSC DC73349) were used to verify the recovery of the As analysis. The total Si contents in shoots of rice seedlings were determined by microwave-NaOH/H2O2 digestion, which was a modification of procedures in Seyfferth and Fendorf (Citation2012). Dried shoot samples (0.05 g), 3 mL 50% NaOH and 2 mL 30% H2O2 were added to a Teflon vessel and mixed evenly. The vessels were sealed and digested in a microwave digestion instrument (Multiwave GO), in which the temperature was raised to 100°C within 5 min and held for an additional 5 min, then raised to 175°C within 10 min and held for an additional 20 min. After cooling, the digests were diluted to 50 mL with deionized water and Si concentrations in digests were determined by ICP-OES.
2.6 Statistical analysis
Measurements presented in this study are means (n = 3) ± standard deviations (SD). One-way analysis of variance (ANOVA) was used to test the effects of soil and foliar Si applications on biomass, plant height and As and Si accumulation in rice seedlings. To compare the differences in measurements between treatments, we used the least significant difference (LSD) test at the level of P = 0.05. ANOVA and LSD tests were performed using the SAS 9.2 software package.
3. Results
3.1 Soil properties
The basic properties of the tested soils are presented in . The soil pH of these two soils were similar (pH 5.0–5.7), and the textures of the Ca and Gd soils were silty clay and clay, respectively. The organic matter (OM), crystalline Fe, amorphous Fe and available SiO2 contents of Gd soils were higher than those of Ca soils. Based on our previous study, the Gd soils had higher retention capacities for Si and As than Ca soils (Lee et al. Citation2014). The As contents in Ca−H and Gd−H soils were higher than the farmland control standard (60 mg kg−1) of Taiwan’s Environment Protection Administration (EPA), and the contents of other heavy metals [Cd, chromium (Cr), copper (Cu), nickel (Ni), lead (Pb) and Zn] were below the soil control standard of farmland (data not shown).
3.2 Soil pore water chemistry
The results of soil pore water chemistry indicate that there were no differences in pH, Eh or the concentrations of Si and As in the tested soils among different Si treatments before the 15th day after transplantation, which is expected because there were no Si amendments during this period (–). As can be seen in , there were no significant differences in pH of the Ca and Gd soils among different Si application rates of foliar treatment. However, in soil applications, the soil pH increased rapidly after the 15th and 30th days due to the addition of sodium silicate solution (pH 11.76). The soil pH increased with Si application rates, which were as high as 8.5 and 9.5 for Ca and Gd soils treated with the +3Si application, respectively. The Eh value of Ca and Gd soils decreased with flooded time, and Si application had little effect on Eh of all tested soils (–h).
Figure 1. The (a–d) pH and (e–h) redox potential of Chiwulan (Ca) and Guandu (Gd) soil with different silicon (Si) treatments during the flooding conditions (F: foliar application; S: soil application; the addition of 0-, 1- and 3-fold recommendation rates of Si in soil application and 0, 0.1 and 0.3% Si in foliar application are expressed as control, +Si and +3Si, respectively; Si was added on the 15th and 30th days).
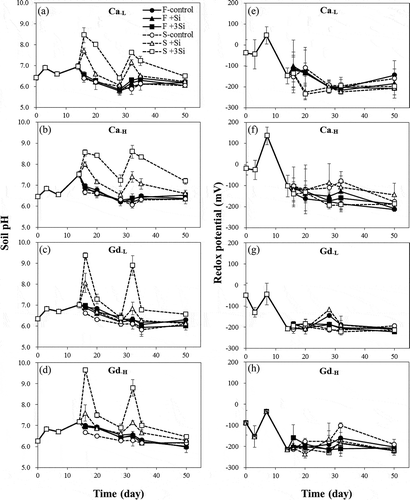
Figure 2. The changes of silicon (Si) concentrations in soil pore water with (a–d) foliar application and (e–h) soil application of Si during the flooding conditions (F: foliar application; S: soil application; the addition of 0-, 1- and 3-fold recommendation rates of Si in soil application and 0, 0.1 and 0.3% Si in foliar application are expressed as control, +Si and +3Si, respectively; Si was added on the 15th and 30th days).
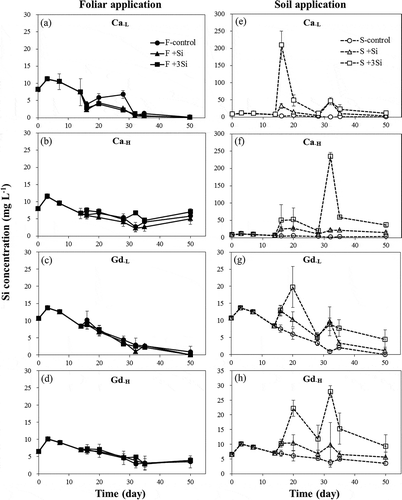
Figure 3. The changes of arsenic (As) concentrations in soil pore water with (a–d) foliar application and (e–h) soil application of silicon (Si) during flooding conditions (F: foliar application; S: soil application; the addition of 0-, 1- and 3-fold recommendation rates of Si in soil application and 0, 0.1 and 0.3% Si in foliar application are expressed as control, +Si and +3Si, respectively; Si was added on the 15th and 30th days).
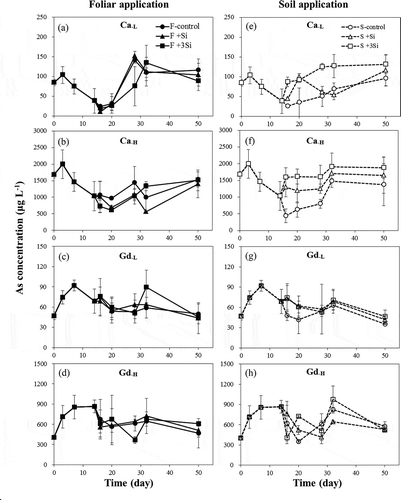
The concentrations of Si and As in soil pore water are presented in and . These figures show that the Si and As concentrations for different Si foliar application rates in the two tested soils were not clearly distinct from one another. However, Si and As concentrations showed pronounced increases with the increasing Si application rates in the soil treatments. The concentrations of Si and As in pore water of Ca soils that underwent soil Si application were higher than those of Gd soils, and the extents of increase of Si and As concentrations of Ca soils were also higher than those of Gd soils after Si application. Compared with the control treatment, the Si concentrations increased by 2.5–126-fold and 1.7–12.0-fold in Ca and Gd soils, respectively, after the addition of Si into the soils. The increases of As concentrations in Ca and Gd soils were 1.2–3.6-fold and 1.1–2.1-fold, respectively.
shows the As species in pore water of tested soils treated with +3Si foliar and soil application during flooded conditions. The results indicate that the concentrations of inorganic As species (iAsIII and iAsV) were higher than those of methylated As species (monomethylarsonic acid (MMA) and dimethylarsinic acid (DMA)). The iAsIII was the predominant As species in the pore water, and the average percentage of iAsIII was higher than 70% of the total As. This indicates that soil Si applications could enhance the release of iAsIII in pore water. The concentrations of iAsIII in soils applied with Si were 1.8- and 1.1-fold of those that received foliar application, for Ca−H and Gd−H soils, respectively. In addition, compared to the control treatment, it was observed that the iAsIII concentrations in pore water of the +3Si treatment were increased on average by 2.4- and 1.2-fold in Ca−H and Gd−H soils, respectively, during flooded conditions (data not shown).
3.3 Biomass and plant height of rice seedlings
The shoot height and biomass of rice seedlings are presented in . For all tested soils, the Si foliar treatments had no significant effects on the shoot height and biomass of rice seedlings. For soil Si treatments, the shoot height and biomass of rice seedlings grown in Ca−H and Gd−H soils decreased by 1.7–8.7 and 9.4–22.6%, respectively, with the increase in Si application rates. However, there were no significant effects on the rice seedlings grown in Ca−L and Gd−L soils, except for the shoot biomass in Ca−L soils, which increased by 22.9 and 32.4% for +Si and +3Si treatments, respectively. The shoot height and biomass of rice seedlings grown in Gd soils were higher than those in Ca soils for both foliar and soil applications. The extent of decrease in shoot height and biomass in Gd−H soils (height: −8.7%; biomass: −22.6%) was higher than in Ca−H soils (height: −4.2%; biomass: −17.6%) for the +3Si soil application. There were no significant differences in root length and biomass of rice seedlings among different Si treatments (data not shown). Moreover, the biomasses of rice shoots grown in As contaminated/spiked soils were significantly lower than those grown in As uncontaminated/un-spiked soils, but there was no obvious effect on the shoot heights of rice grown in As contaminated/spiked soils ().
Figure 5. The (a, c) shoot height and (b, d) shoot biomass of rice (Oryza sativa L.) seedlings grown in Chiwulan (Ca) and Guandu (Gd) soils with different silicon (Si) treatments. (F: foliar application; S: soil application; the addition of 0-, 1- and 3-fold recommendation rates of Si in soil application and 0, 0.1 and 0.3% Si in foliar application are expressed as control, +Si and +3Si, respectively).
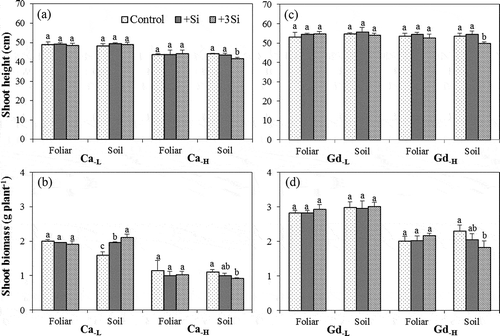
3.4 Si and As accumulation in rice seedlings
The Si and As concentrations measured in plant tissues of rice seedlings are presented in . The concentrations of Si in shoot of rice seedlings were 8.4–18.1 g kg−1 and 8.2–34.6 mg g−1 for foliar and soil treatments, respectively. The Si concentrations in shoots grown in Ca−L and Gd−L soils showed a significant increase by foliar applications of Si, but there were no significant differences while grown in Ca−H and Gd−H soils. For soil treatments, the addition of Si significantly increased Si accumulation in shoots grown in all tested soils. Moreover, the Si concentrations in shoots grown in Gd soils (8.6–34.6 g kg−1) were higher than those in Ca (8.2–29.1 g kg−1) soils treated with both foliar and soil applications. However, the extents of increase of Si concentrations in shoots grown in Ca soils (66–127%) were higher than those in Gd soils (39–106%) after the application of Si into the soils.
Table 2. The concentrations of silicon (Si) in shoot and the concentrations of arsenic (As) in roots (removing iron plaque) and shoots of rice seedlings grown in Chiwulan (Ca) and Guandu (Gd) soils with different As contents and Si treatments.
The As concentrations in roots were higher than in shoots of rice seedlings grown in low (root: 10–62 mg kg−1, shoot: 5.1–19.2 mg kg−1) and high As content soils (root: 72–636 mg kg−1, shoot: 13.7–36.5 mg kg−1) for all Si treatments. There were no significant differences in As accumulation in roots grown in tested soils treated with foliar and soil applications of Si, except for the soil treatments in Ca−L and Ca−H soils. The As concentration in roots of the S + 3Si treatment in Ca-H soil was higher than that of S-control. Instead of decreasing As uptake in roots, As accumulation in roots was enhanced in the S + 3Si treatment of Ca-H soil. The reason for this needs further investigation. The foliar application of Si also had no significant effect on the As accumulation in shoots, but the soil applications of Si into soils significantly reduced the As concentrations in shoots grown in the two tested soils with low and high contents of As. In contrast with Si concentrations in shoots, the concentrations of As in shoots grown in Ca soils were higher than those in Gd soils for all Si treatments. In addition, it was found that there was a significantly negative correlation between the concentrations of As and Si in shoots among different Si soil treatments (R2 = 0.6157, P<0.01; data not shown). The extents of decrease of As concentrations in shoots grown in Ca soils (33–53%) were higher than those in Gd soils (14–27%) for soil applications of Si.
4. Discussion
Numerous studies have reported that the application of Si fertilizers can improve rice growth and reduce As uptake and accumulation in plant tissues grown in As-containing nutrient solutions and soils (Guo et al. Citation2005, Citation2009; Bogdan and Schenk Citation2008; Li et al. Citation2009; Seyfferth and Fendorf Citation2012; Fleck et al. Citation2013; Tripathi et al. Citation2013). However, there has been a lack of studies that have investigated the effects of Si foliar applications on As toxicity and uptake by rice. In our present study, it was found that foliar applications of sodium silicate had no significant effect on the shoot height and biomass of rice seedlings regardless of the Si application rate or difference in As contents of the tested soils (). Ma et al. (Citation1989) showed that the beneficial effect of Si application on the growth of rice plants mainly occurred during the reproductive stage if the Si was fed to the plants during the earlier vegetative stages. Liu et al. (Citation2014) reported that the yields of grain and stalk of mature rice increased after foliar application of Si. In this study, a relevant finding was that there were no significant differences in As accumulation in roots and shoots among different Si foliar treatments, and that Si foliar applications did not elevate the Si concentrations in shoots (). This may be because sodium silicate could not be taken up by rice plants through foliar application, and the consequence was a lack of a significant effect on the As uptake by rice seedlings. However, the study of Liu et al. (Citation2014) indicated that the foliar application of nanoscale silica sol could reduce the As toxicity and accumulation in rice plants by lowering As-induced oxidative stress (e.g., by the increase of anti-oxidant enzymes) and the co-localization of Si and As in cell wall of shoots. These results are contradictory to those found in this study. A possible explanation for the discrepancy could be a difference between the Si materials applied or the rice cultivars used in the present and previous studies (Liu et al. Citation2014).
In the present study, we observed a decrease in Eh and increase in pH of soils under flooding conditions, which enhanced As release into pore water resulting from reductive dissolution of iron oxides and iAsV reduction (Takahashi et al. Citation2004). We also found a significant increase of As concentrations in pore water (up to 1500 μg L−1) corresponding with increased rates of sodium silicate application (–h). This was likely the result of the competition between Si and As for the adsorption sites of soil minerals, such as iron oxides and clay minerals. These results are consistent with those found in previous studies (Swedlund and Webster Citation1999; Luxton et al. Citation2008; Sharma and Kappler Citation2011; Lee et al. Citation2014). Li et al. (Citation2009) also showed that As concentrations were elevated after Si-gel application into soils, and Seyfferth and Fendorf (Citation2012) reported that the extent of As release in pore water was dependent on the solubility of Si materials. It was also found that the extents of Si and As released in pore water were higher in this study than those observed in our previous study with the same Si treatment (1-fold Si fertilizer recommendation rate; Lee et al. Citation2014). This difference could be due to the difference in application methods. In this study, the addition of sodium silicate solutions into soils during plant growth (on the 15th and 30th day after transplantation) might increase the Si availability compared with the spiking of Si into soils before pot experiments. Moreover, it was discovered that the Si and As concentrations in Ca soils were higher than those in Gd soils (–), likely due to the fact that Gd soils have higher amounts of amorphous Fe oxides (), which could enhance the Si and As retention by soils.
Under flooded conditions, iAsIII is the main species in soil pore water, and it has toxic effects on the plant growth of paddy rice (Hoffmann and Schenk Citation2011). An increase of iAsIII concentrations caused by Si application into soils was found in this study (). The decreases of shoot biomass grown in Ca−H and Gd−H soils applied with +3Si treatments were possibly due to As toxicity (). Although it indicated that plant As toxicity by Si applications was observed with increasing of As concentrations in soil pore water, it was also found that As concentrations in shoot of rice seedlings were decreased by 14–53% after the application of Si into the soils (). This may have resulted from the fact that excessive Si existed in pore water competing with iAsIII for uptake by roots, thus reducing As accumulation in rice seedlings, which is supported by the negative correlation between Si and As concentrations in shoots (R2 = 0.6157, P < 0.01; data not shown). These results also reveal that it might have an initial aggravation in As toxicity before the beneficial effects of reducing As uptake by Si fertilizing to rice when Si was applied into As-contaminated soils (Lee et al. Citation2014). Fleck et al. (Citation2013) reported that the As concentrations in straw and flag leaf were decreased by approximately 2-fold after applying about 20 ton ha−1 of SiO2 into soils. Moreover, the As concentrations in brown rice and polished rice were reduced by 23 and 22%, respectively, even though the As concentration in the pore water was increased by the Si applications. Li et al. (Citation2009) also found a decrease of As concentrations in straw and grain by 78% and 16%, respectively, after the addition of silica gel into soils at the rate of about 40 ton SiO2 ha−1. However, in our previous study, Si fertilizer application (0.75 ton SiO2 ha−1) led to As toxicity and an increase of As accumulation in rice seedlings grown in five As contaminated/spiked soils (Lee et al. Citation2014). This indicates that there are differences between the present and previous studies in the effects of soil applications of Si on the As toxicity and uptake by rice seedlings, which could be explained by Si/As molar ratios in soil pore water, as described below.
The Si/As ratios in soil pore water could be regarded as the competitive intensity of Si against As for the uptake by rice, which is dependent on the contents and solubility of Si fertilizers and the properties of soils (Seyfferth and Fendorf Citation2012; Lee et al. Citation2014). As indicated in , the Si/As ratio increased with higher soil Si application rates, and the average Si/As ratios were 230–1432 and 15–64 for low and high As content soils, respectively. The extents of increase in the Si/As ratio of Ca soils by soil Si applications were higher than those of Gd soils, due to the fact that Gd soils have a higher retention capability for As and Si. Therefore, the extents of decrease of the total uptake of As in shoots and shoot biomass in Ca−H soils were higher than those in Gd−H soils. Although Si soil application can reduce As uptake by rice at high Si/As ratios in soil pore water, it should be noted that there were significant decreases in the shoot biomass of rice seedlings grown in Ca−H and Gd−H soils applied with the +3Si treatment. This may be a result of the high As concentrations in the soil pore water, causing As toxicity (). In previous soil pot experiment studies, the Si/As ratios in soil solutions were 70–1450 and 140–730 for Li et al. (Citation2009) and Fleck et al. (Citation2013) after Si application (20–40 ton SiO2 ha−1), respectively. These Si/As ratios are similar to those of this study. The As uptake and accumulation in rice plants were also decreased in these two studies by soil application of Si. However, Lee et al. (Citation2014) reported that the Si/As ratios in As contaminated/spiked soils were less than 30 after the addition of Si at the rate of 0.75 ton SiO2 ha−1. These Si/As ratios are lower than those in the present study. Growth inhibition and an increase of As accumulation in rice seedlings were observed. It suggests that decreasing As uptake may not be expected if the Si/As molar ratio in soil pore water is less than about 30. Therefore, the Si/As ratio in soil pore water is the most important indicator in evaluating the effect on the As toxicity and As uptake by rice after soil application of Si.
Table 3. The average silicon (Si)/arsenic (As) molar ratio in soil pore water, shoot biomass and total uptake of As in shoots of rice seedlings grown in Chiwulan (Ca) and Guandu (Gd) soils with different As contents and Si treatments.
In summary, the results of this study indicate that the foliar application of sodium silicate had no significant effect on the plant growth and As accumulation in rice seedlings grown in low or high As content soils. In contrast, the application of Si at the recommended rate (+Si) into soils could effectively reduce the As accumulation in shoots of rice seedlings grown in As-contaminated/spiked soils, due to the high Si/As ratios in pore water and thus the enhancement of Si–As competition for uptake by rice. However, it was observed that the addition of an excessive amount of Si fertilizer (+3Si) could inhibit plant growth due to As toxicity. This suggests that if the amounts of Si soil applications into As-contaminated soils are kept moderate, they could effectively reduce the As uptake by rice seedlings. The rates and methods of Si fertilizer application and soil properties must be taken into account before Si application into paddy fields.
Acknowledgments
Financial support from the Ministry of Science and Technology, Executive Yuan, Taiwan (grant no. MOST 104-2313-B-002-015-MY3) is sincerely appreciated.
References
- Bogdan K, Schenk MK 2008: Arsenic in Rice (Oryza sativa L.) related to dynamics of arsenic and silicic acid in paddy soils. Environ. Sci. Technol., 42, 7885–7890. doi:10.1021/es801194q
- Chiang KY, Lin KC, Lin SC, Chang TK, Wang MK 2010: Arsenic and lead (beudantite) contamination of agricultural rice soils in the Guandu Plain of northern Taiwan. J. Hazard. Mater., 181, 1066–1071. doi:10.1016/j.jhazmat.2010.05.123
- Daus H, Weiss H, Mattusch J, Wennrich R 2006: Preservation of arsenic species in water samples using phosphoric acid—limitation and long-term stability. Talanta, 69, 430–434. doi:10.1016/j.talanta.2005.10.012
- Fleck AT, Mattusch J, Schenk MK 2013: Silicon decreases the arsenic level in rice grain by limiting arsenite transport. J. Plant Nutr. Soil Sci., 176, 785–794.
- Gee GW, Bauder JW 1986: Particle-size analysis. In Methods of Soil Analysis, Part 1, Physical and Mineralogical Methods, Ed. Klute, A, pp. 383–411. ASA and SSSA, Madison, WI.
- Guntzer F, Keller C, Meunier JD 2012: Benefits of plant silicon for crops: a review. Agron. Sustain. Dev., 32(1), 201–213. doi:10.1007/s13593-011-0039-8
- Guo W, Hou YL, Wang SG, Zhu YG 2005: Effect of silicate on the growth and arsenate uptake by rice (Oryza sativa L.) seedlings in solution culture. Plant Soil, 272, 173–181. doi:10.1007/s11104-004-4732-0
- Guo W, Zhang J, Teng M, Wang LH 2009: Arsenic uptake is suppressed in a rice mutant defective in silicon uptake. J. Plant Nutr. Soil Sci., 172, 867–874. doi:10.1002/jpln.v172:6
- Hoffmann H, Schenk MK 2011: Arsenite toxicity and uptake rate of rice (Oryza sativa L.) in vivo. Environ. Pollut., 159, 2398–2404. doi:10.1016/j.envpol.2011.06.038
- Lee CH, Huang HH, Syu CH, Lin TH, Lee DY 2014: Increase of As release and phytotoxicity to rice seedlings in As-contaminated soils by Si fertilizer application. J. Hazard. Mater., 276, 253–261. doi:10.1016/j.jhazmat.2014.05.046
- Li RY, Stroud JL, Ma JF, McGrath SP, Zhao FJ 2009: Mitigation of arsenic accumulation in rice with water management and silicon fertilization. Environ. Sci. Technol., 43, 3778–3783. doi:10.1021/es803643v
- Liang Y, Sun W, Zhu YG, Christie P 2007: Mechanisms of silicon-mediated alleviation of abiotic stresses in higher plants: a review. Environ. Pollut., 147, 422–428.
- Liu CP, Li FB, Luo CL, Liu XM, Wang SH, Liu TX, Li XD 2009: Foliar application of two silica sols reduced cadmium accumulation in rice grains. J. Hazard. Mater., 161, 1466–1472. doi:10.1016/j.jhazmat.2008.04.116
- Liu CP, Wei L, Zhang SH, Xu XH, Li FB 2014: Effects of nanoscale silica sol foliar application on arsenic uptake, distribution and oxidative damage defense in rice (Oryza sativa L.) under arsenic stress. RSC Adv., 4, 57227–57234. doi:10.1039/C4RA08496A
- Luxton TP, Eick MJ, Rimstidt DJ 2008: The role of silicate in the adsorption/desorption of arsenite on goethite. Chem. Geol., 252, 125–135. doi:10.1016/j.chemgeo.2008.01.022
- Ma JF 2004: Role of silicon in enhancing the resistance of plants to biotic and abioticstresses. Soil Sci. Plant Nutr., 50, 11–18. doi:10.1080/00380768.2004.10408447
- Ma JF, Nishimura K, Takahashi E 1989: Effect of silicon on the growth of rice plantat different growth stages. Soil Sci. Plant Nutr., 35, 347–356. doi:10.1080/00380768.1989.10434768
- Ma JF, Tamai K, Yamaji N, Mitani N, Konishi S, Katsuhara M, Ishiguro M, Murata Y, Yano M 2006: A silicon transporter in rice. Nature, 440, 688–691. doi:10.1038/nature04590
- Ma JF, Yamaji N, Mitani N, Xu XY, Su YH, McGrath SP, Zhao FJ 2008: Transporters of arsenite in rice and their role in arsenic accumulation in rice grain. Proc. Natl. Acad. Sci. U.S.A., 105, 9931–9935. doi:10.1073/pnas.0802361105
- Mckeague JA, Day JH 1966: Dithionite and oxalate extractable Fe and Al as aids in differentiating various classes of soils. Can. J. Soil Sci., 45, 49–62. doi:10.4141/cjss65-008
- McLean EO 1982: Soil pH and lime requirement. In Methods of Soil Analysis, Part 2, Chemical and Microbiological Properties, Eds. Page, AL, Miller, RH, Keeney, DR, pp. 199–223. ASA and SSSA, Madison, WI.
- Meharg AA, Rahman M 2003: Arsenic contamination of Bangladesh paddy field soils: implications for rice contribution to arsenic consumption. Environ. Sci. Technol., 37, 229–234. doi:10.1021/es0259842
- Mehra OP, Jackson ML 1960: Iron oxide removed from soils and clays by a dithionite-citrate system buffered with sodium bicarbonate. Clays Clay Miner., 7, 317–327. doi:10.1346/CCMN.1958.0070122
- Mondal D, Polya DA 2008: Rice is a major exposure route for arsenic in Chakdaha block, Nadia district, West Bengal, India: A probabilistic risk assessment. Appl. Geochem., 23, 2987–2998. doi:10.1016/j.apgeochem.2008.06.025
- Nelson DW, Sommers LE 1982: Total carbon, organic, and organic matter. In Methods of Soil Analysis, Part 2, Chemical and Microbiological Properties, Eds. Page, AL, Miller, RH, Keeney, DR, pp. 539–577. ASA and SSSA, Madison, WI.
- Panaullah GM, Alam T, Hossain MB, Loeppert RH, Lauren JG, Meisner CA, Ahmed ZU, Duxbury JM 2009: Arsenic toxicity to rice (Oryza sativa L.) in Bangladesh. Plant Soil, 317, 31–39. doi:10.1007/s11104-008-9786-y
- Rogalla H, Romheld V 2002: Role of leaf apoplast in silicon mediated manganese tolerance of Cucumis sativus L. Plant Cell Environ., 25, 549–555. doi:10.1046/j.1365-3040.2002.00835.x
- Seyfferth AL, Fendorf S 2012: Silicate mineral impacts on the uptake and storage of arsenic and plant nutrients in rice (Oryza sativa L.). Environ. Sci. Technol., 46(24), 13176–13183. doi:10.1021/es3025337
- Sharma P, Kappler A 2011: Desorption of arsenic from clay and humic acid-coated clay by dissolved phosphate and silicate. J. Contam. Hydrol., 126, 216–225. doi:10.1016/j.jconhyd.2011.08.005
- Shi X, Zhang C, Wang H, Zhang F 2005: Effect of Si on the distribution of Cd in rice seedlings. Plant Soil, 272, 53–60. doi:10.1007/s11104-004-3920-2
- Song A, Li P, Li Z, Fan F, Nikolic M, Liang Y 2011: The alleviation of zinc toxicity by silicon is related to zinc transport and antioxidative reactions in rice. Plant Soil, 344, 319–333. doi:10.1007/s11104-011-0749-3
- Su YH, McGrath SP, Zhao FJ 2010: Rice is more efficient in arsenite uptake and translocation than wheat and barley. Plant Soil, 328, 27–34. doi:10.1007/s11104-009-0074-2
- Sun WC, Zhang J, Fan QH, Xue GF, Li ZJ, Liang YC 2010: Silicon-enhanced resistance to rice blast is attributed to silicon-mediated defense resistance and its role as physical barrier. Eur. J. Plant Pathol., 128, 39–49. doi:10.1007/s10658-010-9625-x
- Swedlund PJ, Webster JG 1999: Adsorption and polymerisation of silicic acid on fer-rihydrite, and its effect on arsenic adsorption. Water Res., 33, 3413–3422. doi:10.1016/S0043-1354(99)00055-X
- Syu CH, Huang CC, Jiang PY, Lee CH, Lee DY 2015: Arsenic accumulation and speciation in rice grains influenced by arsenic phytotoxicity and rice genotypes grown in arsenic-elevated paddy soils. J. Hazard. Mater., 286, 179–186. doi:10.1016/j.jhazmat.2014.12.052
- Takahashi E 1995: Uptake mode and physiological functions of silica. Sci. Rice Plant, 2, 58–71.
- Takahashi Y, Minamikawa R, Hattori KH, Kurishima K, Kihou N, Yuita K 2004: Arsenic behavior in paddy fields during the cycle of flooded and non-flooded periods. Environ. Sci. Technol., 38, 1038–1044. doi:10.1021/es034383n
- Tchounwou BP, Centeno JA, Patlolla AK 2004: Arsenic toxicity, mutagenesis, and carcinogenesis–a health risk assessment and management approach. Mol. Cell Biochem., 255, 47–55. doi:10.1023/B:MCBI.0000007260.32981.b9
- Tripathi P, Tripathi RD, Singh RP, Dwivedi S, Goutam D, Shri M, Trivedi PK, Chakrabarty D 2013: Silicon mediates arsenic tolerance in rice (Oryza sativa L.) through lowering of arsenic uptake and improved antioxidant defence system. Ecol. Eng., 52, 96–103. doi:10.1016/j.ecoleng.2012.12.057
- Wang SH, Wang FY, Gao SC 2015: Foliar application with nano-silicon alleviates Cd toxicity in rice seedlings. Environ. Sci. Pollut. Res., 22, 2837–2845. doi:10.1007/s11356-014-3525-0
- Williams PN, Raab A, Feldmann J, Meharg AA 2007a: Market basket survey shows elevated levels of As in South Central US processed rice compared to California: consequences for human dietary exposure. Environ. Sci. Technol., 41, 2178–2183. doi:10.1021/es061489k
- Williams PN, Villada A, Deacon C, Raab A, Figuerola J, Green AJ, Feldmann J, Meharg AA 2007b: Greatly enhanced arsenic shoot assimilation in rice leads to elevated grain levels compared to wheat and barley. Environ. Sci. Technol., 41, 6854–6859. doi:10.1021/es070627i
- Xu GH, Zhan XH, Li CH, Bao SD, Liu XB, Chu TD 2001: Assessing methods of available silicon in calcareous soils. Commun. Soil Sci. Plant Anal., 32, 787–801. doi:10.1081/CSS-100103909
- Xu XY, McGrath SP, Meharg AA, Zhao FJ 2008: Growing rice aerobically markedly decreases As accumulation. Environ. Sci. Technol., 42, 5574–5579.
- Zhao FJ, McGrath SP, Meharg AA 2010: Arsenic as a food chain contaminant: mechanisms of plant uptake and metabolism and mitigation strategies. Annu. Rev. Plant Biol., 61, 535–559. doi:10.1146/annurev-arplant-042809-112152