ABSTRACT
This study evaluated effects of oats (Avena sativa L.) and grazing vetch (Vicia dasycarpa L.), bicultures, in rotation with summer maize (Zea mays), on soil organic matter fractions and activities of selected enzymes. The trial was initiated in April 2009. The treatments were 100% oat, 100% vetch, 90% oat + 10% vetch, 70% oat + 30% vetch, 50% oat + 50% vetch, and weedy fallow, in a randomized complete block design (RCBD) with three replications. Soil samples were collected in October 2011, from the 0–5 and 5–20 cm depths, and analyzed for total carbon (C), particulate organic matter (POM), water-soluble carbon (WSC), microbial biomass carbon (MBC) and activities of selected enzymes. Total C was higher in bicultures, particularly the 70% oat + 30% vetch, and 100% vetch than in 100% oats and the control. The greatest MBC, WSC, dehydrogenase, aryl-sulphatase and phosphomonoesterase activities were in the 70% oat + 30% vetch biculture, and declined where the proportion of oats or vetch was higher. Increasing proportions of vetch resulted in increases in urease and β-glucosidase activity and decrease in POM. The findings suggested that, in addition to increased maize yields, bicultures of oats and vetch also have synergistic effects on soil carbon pools and enzyme activities, with potential benefits of improved soil physical condition and nutrient cycling compared with the individual crops, under warm temperate conditions.
1. Introduction
Most agricultural soils in Southern Africa have low soil fertility (Mills and Fey Citation2003; Mandiringana et al. Citation2005). The majority of smallholder farmers in the region practice maize monoculture, usually under conventional moldboard tillage with little or no fertility replenishment (Mandiringana et al. Citation2005). Intensive tillage, short to no fallow periods, virtual absence of crop rotations, overgrazing and removal of crop residues, among other practices, exacerbate the decline of soil organic matter and fertility in these soils (Fox and Rowntree Citation2001; Laker Citation2004; Mandiringana et al. Citation2005). Consequently, maize (Zea mays L.) yields are low, averaging less than 1.0 t ha−1 under dry land systems and 3 t ha−1 under irrigation (Fanadzo Citation2007). Improvements in soil organic matter (SOM) can result in several benefits, including improved soil nutrient storage capacity, nutrient availability, biological activity, soil structure and resistance to erosion (Brady and Weil Citation2008). The need to improve organic matter content and productivity of soils has prompted promotion of alternative approaches such as conservation agriculture (CA).
The promotion of CA in the low-input smallholder farming systems is meant to improve and restore soil organic matter and fertility. While a number of entry points could be used, winter cover crops have been identified as an avenue of introducing CA on smallholder farms of the Eastern Cape (EC), South Africa. Murungu et al. (Citation2011) identified grazing vetch (Vicia dasycarpa L.) and white oats (Avena sativa L.) as candidate legume and grass cover crop species, respectively, to provide sufficient soil cover to address weed and soil fertility challenges experienced on smallholder farms in the EC. The use of maize–oat and maize–vetch rotations has shown improvements in light organic matter fractions (Dube et al. Citation2012). Maize–oat rotations have been found to produce residues that degrade more slowly, and are more persistent to provide mulch with moisture conservation and weed suppression benefits. On the other hand, residues from maize–vetch rotations break down faster, releasing nutrients for the benefit of the subsequent crop (Murungu et al. Citation2011). In low-input smallholder systems, combined benefits from these two cover crops could make a significant contribution to crop and soil productivity. Bicultures of these two winter cover crops could be important to realize these benefits. Bicultures have been reported to moderate carbon (C):nitrogen (N) ratios, resulting in intermediate decomposition and mineralization, compared to their sole crops (Sainju et al. Citation2005). Their effectiveness could depend on the proportions of each component cover crop.
Soil organic carbon (SOC) is proposed as a primary indicator of soil quality (Conteh et al. Citation1997; Reeves Citation1997), especially in the surface soil (Franzluebbers Citation2002). Returning more residues to the soil is associated with an increase in SOC concentration (Wilhelm et al. Citation2004; Dolan et al. Citation2006) and bicultures of cover crops could have a significant effect in this regard. Dube et al. (Citation2012) reported high levels of light organic matter fractions [particulate organic matter (POM) and water-soluble carbon (WSC)] in CA systems involving rotations of maize with either oat or grazing vetch. POM is the most labile fraction of soil organic matter (SOM) that is readily formed and decomposed and is an important substrate for soil mineralization processes (Cambardella and Elliot Citation1992). WSC is the most dynamic C pool in soils and forms a small proportion of the total SOM (Herbert and Bertsch Citation1995). Bicultures of oat and vetch, in rotation with maize, could result in greater improvements in organic matter and nutrient cycling through the addition of greater biomass. Muzangwa et al. (Citation2012) reported greater biomass inputs from oats and vetch in bicultures than as separate crops. The proportion of oats and grazing vetch that facilitates both accumulation of organic matter and nutrient cycling needs to be determined.
In low-external-input cropping systems like the EC smallholding farms, the mineralization of OM contributes to soil fertility. Total organic matter and its light fractions work as sources of nutrients through their function as substrates for soil microbial communities. The soil microbial biomass is an important labile pool of C, N, phosphorus (P) and sulfur (S), and fluctuations in its size and activity can significantly influence crop productivity. Microbial degradation of organic matter is facilitated by enzymes associated with specific nutrients. Analyses of the activity of specific soil enzymes indicate the potential for biochemical processes associated with nutrient cycling in the soil. While a host of enzymes could be involved in overall nutrient cycling, and measurement of absolute enzyme activities is difficult, in the soil (Nannipieri et al. Citation2012), the available assays of individual enzymes can indicate relative effects of differences in soil management.
Mukumbareza et al. (Citation2015) reported that vetch and fertilized oat cover crops, in rotation with maize, increased microbial biomass carbon (MBC) and activities of dehydrogenase, β-glucosidase, urease, and acid and alkaline phosphomonoesterases. Under the same conditions, Dube et al. (Citation2012) reported that fertilized vetch and oat cover crops, in rotation with maize, improved POM, and WSC in the soil. Could there be synergistic effects of oats and vetch in bicultures on organic matter pools and enzyme activities, when compared to monocultures of the component crops? What is the ideal oats:vetch ratio that gives the greatest improvement in soil carbon pools and enzyme activities under warm temperate conditions? This study concentrated on total C, POM, WSC and activities of dehydrogenase, β-glucosidase, urease, aryl-sulfatase, acid and alkaline phosphomonoesterase. The objective of this study was to determine effects of ratios of oat-to-vetch in bicultures on labile organic matter pools and activities of selected enzymes in a no-till irrigated maize-based CA system, when compared with their monocultures. The hypothesis was that ratios of oat and vetch cover crop bicultures would improve organic matter pools and activities of soil enzymes, more than their respective monocultures in maize-based CA systems.
2. Materials and methods
The study was carried out at the University of Fort Hare Research Farm (32°47′51″S, 27°50′55″E; altitude 535 m) on a piece of land with 0.5% slope. The climate is warm temperate with annual mean temperature of 18.1°C and average winter and summer rainfall of 167 mm and 430 mm, respectively. The site had deep alluvial soils, classified as the Oakleaf form in the South African Soil Classification System (Soil Classification Working Group Citation1991), and translated to Haplic Cambisol (Eutric) in the World Reference Base for Soil Resources (Fey Citation2010). The soil had 64.2% sand, 16.0% silt, 19.8% clay, 8.4 g kg−1 organic carbon and a pH of 6.2 (Mandiringana et al. Citation2005). These are among the most commonly cropped soils in smallholder farms of the Eastern Cape Province of South Africa. The site had been under rotations of maize and lucerne (Medicago sativa L.) in the previous five years.
2.1. Treatments and experimental design
The cover crops were first planted on 29 April, and harvested on 5 September 2009 (winter season). Five species ratios of grazing vetch (Vicia dasycarpa var. Max) and white oats (Avena sativa var. Pallinup), and a weedy fallow control, were evaluated using a randomized complete block design replicated three times. The cover crop treatments were 90% oat + 10% vetch, 70% oat + 30% vetch, 50% oat + 50% vetch, 100% oat and 100% vetch. The ratios were based on the percentage of the recommended seed rate used for sole oats (100 kg ha−1) and vetch (50 kg ha−1). Seeds of cover crops were drilled into small furrows spaced 30 cm apart in 5 m × 4 m plots. Grazing vetch was inoculated with Rhizobium leguminosarium bio var viciae inoculants with 5 × 108 rhizobial cells g−1 (Stimuplant CC, Zwavelpoort 0036, SA) at planting. Only basal fertilizer was applied to the cover crop, at planting, at 200 kg ha−1 2: 3: 4 (30 + 0.5% Zinc (Zn)) N:P:potassium (K) compound fertilizer, which supplied 13.3 kg N ha−1, 20 kg P ha−1 and 26.7 kg K ha−1. Neither weed nor pest control was done during the growth of the cover crop. Cover crop growth was terminated at the early flowering stage using a tractor-drawn roller and glyphosate (N-phosphonomethyl glycine) herbicide (360 g L−1) at a rate of 5 L ha−1, before planting maize in summer.
Maize (variety SC 701) was planted in plots previously grown to the cover crop and weedy fallow treatments at a spacing of 30 cm in row and 90 cm inter-row, targeting a population of 37,000 plants ha−1. The maize was planted on 28 October 2009 and harvested on 9 April in 2010, 24 weeks after planting. Jab planters were used to drop 2–3 seeds, which were thinned to one plant per station at 3 weeks after planting (WAP). Fertilizer application in the maize was at a rate of 60 kg N ha−1 (smallholder farmer practice in the EC) with a third of the N applied as a basal compound fertilizer with an N:P:K ratio of 2:3:4 (30 + 0.05% Zn) at planting. The remainder was applied as a top dressing of lime ammonium nitrate (LAN) (28% N) at 6 WAP. Weed control was done using Basagran (a.i: thiadiazine 480 g L−1) applied at 5 L ha−1. Overhead irrigation water was applied to all treatments, when necessary, to supplement the normal winter and summer rains. After maize harvest, the residues were retained as surface cover, before planting the next cover crop. The full experiment, including the rotations, was repeated for three cover crop and two maize cropping seasons. In 2010, the cover crops were planted on 30 April and harvested on 10th September, and the follow-on maize was planted on 31 October 2010 and harvested on 11 April 2011. The next cover crop was planted on 1 June 2011 and terminated during the last week of September, 2011. The average annual cover crop (winter 2009 and 2010) maize (summer 2009/2010 and 2010/20110) biomass inputs and maize yields (2010/2011) for the different treatments are given in . The concentrations of C, N and P, and C:N ratios of the residues from the different cover crop treatments, are presented in . The average annual C inputs from both the cover crops and maize biomass are presented in .
Table 1. Average annual cover crop maize biomass inputs across seasons and maize yields (2010/2011), as affected by the different cover crop treatments.
Table 2. Average concentrations of carbon (C), nitrogen (N) and phosphorus (P) and C:N and C:P ratios of the residues of the different cover crop treatments.
Table 3. Average annual carbon inputs as cover crop (winter 2009 and 2010) and maize biomass (summer 2009/2010 and 2010/2011), as affected by the different cover crop treatments.
2.2. Soil sampling
Soil sampling was done after three seasons of cover crops and two seasons of maize. At the time of sampling soil bulk density ranged from 1560 to 1700 kg m−3, and did not significantly vary among the treatments (Mupambwa Citation2012). The samples were collected 2 weeks after cover crop termination before maize planting in each plot, in October 2011. The samples were collected from the 0–5 cm and 5–20 cm depths using a 7-cm-diameter precision auger. Three soil sub-samples were randomly taken for each depth per plot, avoiding a 1-m boundary on all sides. Soil samples from the same depth in each plot were mixed together to make a composite. The soil was then taken to a shade, where it was sieved (2 mm). Portions of the samples were air-dried before analysis of total C, POM and WSC. The sub-samples for analysis of MBC and enzyme activities were stored in the cold room at < 4°C.
2.3. Soil analyses
Total C was analyzed using a LECO CNS analyzer (LECO Corporation Citation2003). POM was determined, after soil dispersion with 1 M sodium hexametaphosphate, following the method by Cambardella and Elliot (Citation1992). WSC was determined by the dichromate oxidation method (Witt et al. Citation2000), after extraction with cold water based on the method by Haynes and Francis (Citation1993).
Soil moisture of subsamples was adjusted to 80% of field capacity, before incubation at 37°C, for 7 days, to activate soil microorganisms. MBC was determined following the modified chloroform fumigation–extraction method (Witt et al. Citation2000). Activity of dehydrogenase in soil was determined according to Tabatabai (Citation1994), while β-glucosidase was determined using the method of Dick et al. (Citation1996). Urease activity was based on determination of urea remaining after incubation of the soil with added urea as detailed by Klose and Tabatabai (Citation2000). Aryl-sulphatase activity was analyzed based on the method of Tabatabai and Bremner (Citation1972). Activities of acid and alkaline phosphomonoesterase were based on the method of Tabatabai (Citation1994).
2.4. Data analysis
Data of soil C pools and enzyme activities were subjected to two-way analysis of variance (ANOVA) as an RCBD with three replications, to test the effects of cover crop biculture and depth, using Genstat Statistical Package, 12th edition. Where significant differences occurred, separation of means was done using the Tukey-Kramer test at 5% level of significance. Correlation analysis of the different parameters with C inputs from cover crops, maize and the combination was done using SPSS Release 16.0.
3. Results
3.1. Total C and microbial biomass C
Interaction effects of cover crop treatment and soil depth on total C and MBC were not significant (p ˃ 0.05), but both main effects were significant (). Only the 100% vetch treatment had higher total C than the weedy fallow (). The highest MBC was in the 70% oats + 30% vetch followed by the 90% oats + 10% vetch with lower levels in the 50% oats + 50% vetch, which was similar to 100% oats and 100% vetch treatments. The weedy fallow treatment had the least MBC (). The 0–5 cm depth had higher total C and MBC than the 5–20 cm depth.
Table 4. P-values from the two-way analysis of variance on soil carbon (C) pools and enzyme activities, with cover crop treatment and depth as factors.
Table 5. Total and microbial biomass carbon (C) under different cover crop treatments and depths.
3.2. Particulate organic matter and water-soluble carbon
There were significant interaction effects of cover crop biculture treatment and soil depth on POM and WSC (). The weedy fallow had the least POM (). In the cover crop treatments, the 100% vetch had the least POM, which increased with increase in the proportion of oat in the biculture, with highest in the 100% oat treatment. The 70% oat + 30% vetch treatment had similar POM levels with the 90% oat + 10% vetch treatment. The WSC was highest in the 70% oat + 30% vetch treatment, and decreased with higher proportions of vetch and oats (). The 50% oat + 50% vetch had similar WSC to the 100% vetch in the 0–5 cm depth, while the weedy fallow had the least WSC at both depths.
Table 6. Particulate organic matter (POM) and water soluble carbon (WSC) under different cover crop treatments and depth.
3.3. β-glucosidase and urease activity
There were significant interaction effects of cover crop biculture treatment and soil depth on β-glucosidase and urease activity (p < 0.001; ). At the 0–5 cm depth, β-glucosidase and urease activity was least in the weedy fallow and highest in 100% vetch, which had similar β-glucosidase activity to the 70% oats + 30% vetch. Activities of the enzymes decreased with an increase in the proportion of oats in the biculture. All biculture treatments had similar β-glucosidase activity at the 5–20 cm depth (). The 50% oats + 50% vetch treatment had similar urease activity to the 100% vetch in the 0–5 cm depth. At the 5–20 cm depth, the 100% oats had similar urease activity to the 90% oats + 10% vetch, while the 70% oats + 30% vetch had similar levels to the 50% oats + 50% vetch treatment ().
Table 7. Cover crop treatment and soil depth effects on β-glucosidase (μg PNP g−1 soil h−1) and dehydrogenase activity (μg TPF g−1 soil h−1).
3.4. Dehydrogenase and aryl-sulphatase activity
There were significant interaction effects of cover crop biculture treatment and soil depth on dehydrogenase and aryl-sulphatase activity (p < 0.001; ). The results of dehydrogenase and aryl-sulphatase activity followed a similar trend to that of WSC, with the highest activity in the 70% oat + 30% vetch treatment, declining with an increase in the proportion of oats and vetch, and the least being in the weedy fallow (). In the 0–5 cm depth, the 100% oats had higher aryl-sulphatase activity than the 50% oats + 50% vetch treatment.
Table 8. Cover crop treatment and soil depth effects on urease (μg urea g−1 soil h−1) and aryl-sulphatase activity (μg PNP g−1 soil h−1).
3.5. Acid and alkaline phosphomonoesrase activity
The interaction effects of cover crop biculture treatment and soil depth on acid- and alkaline phosphomonoesterase activity were significant (p < 0.001; ). All other treatments had greater acid and alkaline phosphomonoesterase activities than the weedy fallow, except the 50% oats + 50% vetch which had similar acid phosphomonoesterase activity at the 0–5 cm depth. The results of both acid and alkaline phosphomonoesterase activities followed the same trend as that of WSC, dehydrogenase and aryl-sulphatase activity, with the highest activity in the 70% oat + 30% vetch treatment, declining with increase in the proportion of oats or vetch, and the least being in the weedy fallow (). Also similar to aryl-sulphatase, the 100% oats had similar alkaline phosphomonoesterase activity to the 50% oats + 50% vetch treatment.
Table 9. Cover crop treatment and soil depth effects on acid and alkaline phosphatase activity (μg PNP g−1 soil h−1).
3.6. Correlations
Average annual C input from cover crops was significantly correlated with WSC (p = 0.037), aryl suphatase (p = 0.021) and alkaline phosphomonesterase (p = 0.002) activity (). The correlations of C additions by maize with total soil C (p = 0.005), β-glucosidase (p = 0.001) and urease (p = 0.009) activity were highly significant. The annual total C (cover crops and maize) input was significantly correlated with total soil C (p = 0.019), β-glucosidase (p = 0.008), urease (p = 0.024) and alkaline phosphomonoesterase (p = 0.020; ). There were no significant correlations between POM and any other parameters tested. WSC was significantly correlated with MBC (p = 0.011), and activities of dehydrogenase (p = 0.027), aryl sulphatase (p = 0.022) and acid (p = 0.037) and alkaline (p = 0.015) phosphomonoesterase ().
Table 10. Correlation coefficients (r) of soil carbon (C) pools and enzyme activity with carbon inputs.
4. Discussion
Improvement of organic matter content of degraded agricultural soils could enhance physical and chemical properties, and biological activity (Salazar et al. Citation2011), and promote productivity of the soil. Bicultures of oats and grazing vetch could be essential in this regard. In our study, the higher total soil C in the 100% vetch than in the control indicated that grazing vetch is required for improving total C in soil. Dube et al. (Citation2012) also reported improvements in soil C after four years of rotations of maize and cover crops (oat and grazing vetch) under the same soil and climatic conditions. The results of total soil C could be explained by the annual biomass and C inputs through the rotations of maize and cover crops, with maize C inputs contributing more than the cover crop C, based on the correlation analysis. Increasing maize biomass could be more desirable than cover crops to improve sequestration of soil C in systems where these crops are used in rotation, as part of conservation agriculture.
The increase in total C, urease and β-glucosidase activity in the bicultures, particularly the 100% vetch and 70% oats + 30% vetch relative to 100% oats, indicated that these cover crops could improve soil structure, nutrient- and water-holding capacities, and biological activity (Brady and Weil Citation2008), with practical benefits on fertilizer and soil water management. The increase in activity of urease and β-glucosidase with increase in the proportion of vetch in the biculture was in response to total soil C accumulation, as a result of the large quantities of residues retained and C inputs, particularly from maize residues. The higher maize biomass and C inputs were in response to greater N and P uptake in the bicultures and sole vetch, as reported by Muzangwa et al. (Citation2015) for the same trial. Mukumbareza et al. (Citation2015) also reported greater β-glucosidase and urease activity in vetch than oat cover crop treatments, and the absolute levels of activities of these enzymes were similar to those in our study. Although single enzyme activities may not explain entire nutrient cycling processes, due to the involvement of a number of enzymes in the processes and the difficulties of measurement of actual activities in soil, among other challenges (Nannipieri et al. Citation2012), they give an indication of changes. Management strategies that increase activities of individual enzymes contribute to nutrient cycling.
The increase in activity of β-glucosidase with the increasing vetch component of the biculture indicates the possibility of hydrolytic conversion of cellulose, the most important organic compound in plant residues, to glucose (Deng and Tabatabai Citation1996). Although urease hydrolyzes urea to ammonium-N (and not mineralization of organic N; Nannipieri et al. Citation2012), it is widely used to monitor changes in soil quality due to management (Chang et al. Citation2007). The high urease activity in 100% vetch and bicultures suggested greater potential for N cycling attributed to lower C:N ratio, in agreement with Garcia et al. (Citation1994) and Pascual et al. (Citation1999). The high quantities of residue inputs from the previous crops (cover crops with vetch and maize) (Muzangwa et al. Citation2012) increased total soil C and WSC, a readily available substrate, and increased MBC and the activity of enzymes.
The results of WSC could be explained by the overall biomass and C inputs, with cover crop C inputs contributing more than the maize C, based on the correlation analysis. The higher WSC and MBC, and activities of dehydrogenase, aryl-sulphatase and phosphomonoesterases, indicated that the cover crops have synergistic effects in bicultures and could both be beneficial to farmers, through potential improvements in nutrient cycling and soil physico-chemical properties. The synergistic effects could be explained by the annual biomass inputs of the cover crop bicultures, particularly the 70% oats + 30% vetch and 90% oats + 10% vetch. Increasing cover crop biomass could be more desirable than maize to improve the labile WSC, microbial biomass and nutrient cycling in systems where these crops are used in rotation, as part of conservation agriculture. From and Muzangwa et al. (Citation2012), the 70% oats + 30% vetch and the 90% oats + 10% vetch contributed the greatest dry-matter input compared to the individual cover crops and the weedy fallow. The addition of fresh organic materials from both cover crops could have provided a ready substrate for the soil microbial communities. It is essential to note that these synergistic effects also increased maize grain and biomass yield (), and the maize biomass inputs increased soil total C, and the activity of most enzymes in this study.
WSC is among the most labile pools of SOM and it serves as an important reservoir of plant nutrients since it acts as a readily decomposable substrate for soil microbial communities (Gregorich et al. Citation1994), especially in agricultural ecosystems. The strong correlation of WSC with MBC, dehydrogenase, aryl sulphatase, and phosphomonoesterase activity suggested that microbial biomass utilized water-soluble organic matter as a substrate. This view was further supported the strong positive relationship between WSC and MBC at both 0–5 and 5–10 cm depths (, ). Although Mukumbareza et al. (Citation2015) reported greater MBC in oat than in vetch cover crop treatments, there was no difference between the 100% oats and the 100% vetch in our study. A high proportion of the readily biodegradable WSC, as observed in this study, promoted the growth of soil microbial communities and associated enzymes, possibly contributing to ready nutrient release. The decrease in MBC, WSC and activities of dehydrogenase, aryl-sulphatase and phosphomonoesterase enzymes with depth could have been due to the close relationship between WSC and total soil C, which is more concentrated in surface layers (Gregorich et al. Citation1994; Carter et al. Citation2002), especially in CA systems that emphasize minimum soil disturbance. In many agricultural soils, where concentrations of labile carbon are low, the microbial biomass is often starved because it does not have enough organic C (Murphy et al. Citation1998). In agricultural soils, 85% of WSC is readily degradable (Zsolnay and Steindl Citation1991), compared to 3.8–39.9% in forest soil leachates (Boissier and Fontvieille Citation1993).
Figure 1. Microbial biomass carbon (MBC) response to water soluble carbon (WSC) at (a) 0–5 cm and (B) 5–20 cm soil depths.
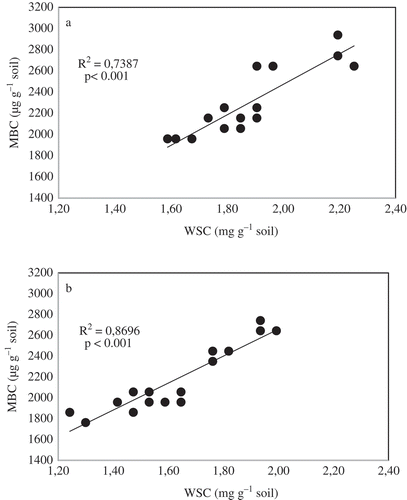
Microbial biomass C and enzyme activities have been found to respond to changes of management such as tillage, compared to physical and chemical properties (Dick Citation1994; Gregorich et al. Citation1994; Bandick and Dick Citation1999). Soil enzyme activities are affected not only by the type and chemical nature of the organic matter, but also by temperature, soil moisture and soil pH, all of which are modified as a result of retention of residues on the soil surface. Mulching, through retention of plant residues, supplies readily available substrate for microbial communities and increases enzyme activities in soil (Monreal and Bergstrom Citation2000). While the assays used only show potential (and not actual) enzyme activities in the soil (Nannipieri et al. Citation2012), they indicate the greater potential of bicultures than individual component cover crops.
Dehydrogenase contributes to the biological oxidation of SOM (Zhang et al. Citation2010), and the strong correlation of WSC, and MBC, with dehydrogenase activity could be explained by the effects of WSC as a substrate promoting growth of microbial biomass, which is associated with the dehydrogenase enzyme. Among all enzymes in the soil environment, dehydrogenase is used as an indicator of overall soil microbial activity because it occurs intra-cellularly in all living microbial cells (Zhao et al. Citation2010; Yuan and Yue Citation2012). Higher aryl-sulphatase enzyme activity under cover crops than weedy fallow was in agreement with Deng and Tabatabai (Citation1997). The greater activity of arylsulfatase, the enzyme which contributes to mineralization of ester sulphates in soil (Tabatabai Citation1994), under the 70% oat + 30% vetch treatment, reflects an increase of fungal biomass (Newell et al. Citation1987). Aryl-sulfatase has a strong correlation with ergosterol, which is almost exclusively found in fungi. Fungi have up to 42% of their S as ester sulphate, while bacteria have around 10% ester sulphate-S (Saggar et al. Citation1981). These results suggest that bicultures could result in greater accumulation of the enzyme involved in S cycling than the individual component cover crops. The strong correlation between MBC (and dehydrogenase activity) with aryl-sulphatase activity suggests that fungal biomass dominated the active microbial biomass in bicultures, where these parameters were highest.
Phosphomonoesterases particularly catalyze the hydrolysis of P-ester bonds binding P to C in OM (Nannipieri et al. Citation2012), and are known to be more concentrated in surface soil layers and the rhizosphere, where most of the fresh and less-humified OM prevails (Tabatabai Citation1994; Deng and Tabatabai Citation1997). The higher phosphomonoesterase activity in bicultures could have been in response to greater WSC as a result of higher biomass additions than oats and vetch (Muzangwa et al. Citation2012), with lower C:P than oat. This view was supported by the correlation analysis results, which showed that phosphomonoesterase activity was strongly affected by WSC. The effect of the biculture was particularly more evident for alkaline than for acid phosphomonoesterase, possibly due to the relatively high pH of the soil (Nannipieri et al. Citation2011).
Phosphorus is one of the major soil nutrients limiting crop yields in sub-Saharan Africa, where the soils are generally deficient (Barnard and du Preez Citation2004). Increased acid and alkaline phosphomonoesterase activities in the bicultures, compared to individual component crops, indicates that such bicultures could increase P cycling in soils under CA. The higher phosphomonoesterase activity in biculture could explain the higher soil-available P in biculture treatments than in the 100% oat reported by Muzangwa et al. (Citation2015) for the same trial. Large proportions of phosphorus in many soils are organically bound, and the mineralization of these portions is of agricultural and economic importance. While WSC is important for nutrient cycling, POM could also make a contribution.
Although POM was not correlated with any of the C inputs, the levels appear to be related to oat composition of the biculture. The lower total C and the higher POM content in the 100% oats suggests that the addition of vetch in the bicultures greatly increases non-POM pools, while oats contribute more to POM build-up. The higher biomass produced by oat and grazing vetch, and their bicultures, and the retention of residues on the soil surface without soil disturbance, could explain the POM build-up in the 0–5 cm depth, when compared to the weedy fallow (Franzluebbers and Arshad Citation1997). These results are in agreement with those from a long-term cover crop study by Sasal et al. (Citation2010). Although Dube et al. (Citation2012) reported no differences in POM between oat and vetch cover crops, the 100% oats had greater levels than the 100% vetch in our study. The finding could be explained by the C:N ratio, which ranged from 18.2 (100% oats) to 10.0 (100% vetch), with bicultures having C:N ratios that increased with increase in the proportion of oats (Muzangwa et al. Citation2015). The weaker or absent relationship of MBC with POM than with WSC at both depths (, ) implies that microbial activity was lower in treatments with high POM concentrations. The lower C:N and C:P ratios in vetch make it more prone to rapid decomposition, increasing MBC and the activity of enzymes, improving the potential for nutrient cycling.
Figure 2. Microbial biomass carbon (MBC) response to particulate organic matter (POM) at (A) 0–5 cm and (B) 5–20 cm soil depths.
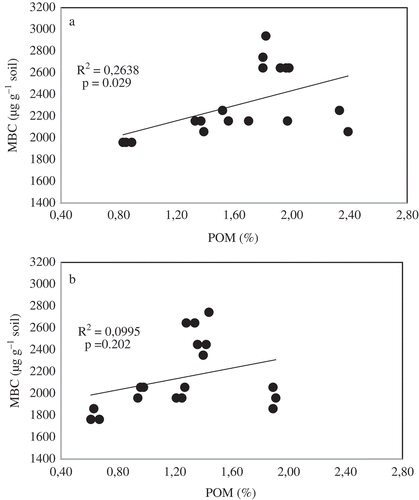
It can be concluded that, in bicultures, oats and vetch have synergistic effects on maize biomass and grain yields, soil C, WSC and MBC and activities of the selected enzymes, particularly in the 70% oat + 30% vetch ratio, while increasing the proportion of oat increased POM. Bicultures that increase the annual biomass input from the maize component of the rotation are more effective in total soil C accumulation and urease and β-glucosidase activity. Increasing the annual cover crop biomass and C input increases the WSC, MBC and activities of dehydrogenase, aryl sulphatase and acid and alkaline phosphomonoesterases. The synergistic effects of two cover crops in the 70% oats + 30% vetch biculture could contribute to soil quality and nutrient cycling, in addition to improved maize yields, in CA systems. This work was based on bicultures that received supplementary irrigation and a high level of management, and further work should test these bicultures under dry land conditions on different soil types on farmers’ fields.
Additional information
Funding
References
- Bandick AK, Dick PD 1999: Field management effects on soil enzyme activities. Soil Biol. Biochem., 31, 1471–1479. doi:10.1016/S0038-0717(99)00051-6
- Barnard RO, du Preez CC 2004: Soil fertility in South Africa: the last twenty five years. S. Afr. J. Plant Soil, 21, 301–315. doi:10.1080/02571862.2004.10635066
- Boissier JM, Fontvieille D 1993: Biodegradable dissolved organic matter in seepage waters from two forest soils. Soil Biol. Biochem., 25, 1257–1261. doi:10.1016/0038-0717(93)90222-W
- Brady NC, Weil RR 2008: The Nature and Properties of Soils, Pearson Prentice Hall, New Jersey.
- Cambardella CA, Elliot ET 1992: Particulate soil organic matter changes across a grassland cultivation sequence. Soil Sci. Am. J., 56, 777–783. doi:10.2136/sssaj1992.03615995005600030017x
- Carter MR, Sanderson JB, Ivany JA, White RP 2002: Influence of rotation and tillage on forage maize productivity, weed species and soil quality of a fine sandy loam in the cool-humid climate of Atlantic Canada. Soil Till. Res., 26, 549–559.
- Chang EH, Chung RS, Tsai YH 2007: Effect of different application rates of organic fertilizer on soil enzyme activity and microbial population. Soil Sci. Plant Nutr., 53, 132–140. doi:10.1111/j.1747-0765.2007.00122.x
- Conteh A, Blair GL, MacLeod DA, Lefroy RDB 1997: Soil organic carbon changes in cracking clay soils under cotton production as studied by carbon fractionation. Aust. J. Agric. Res., 48, 1049–1058. doi:10.1071/A96177
- Deng SP, Tabatabai MA 1996: Effect of tillage and residue management on enzyme activities in soils: II. Glycosidases. Biol. Fert. Soil, 22, 208–213. doi:10.1007/BF00382514
- Deng SP, Tabatabai MA 1997: Effect of tillage and residue management on enzyme activities in soil. III. Phosphatases and arylsulfatase. Biol. Fert. Soil, 24, 141–146. doi:10.1007/s003740050222
- Dick RP 1994: Soil enzyme activities as indicators of soil quality. In Defining Soil Quality for a Sustainable Environment, Eds. Doran, JW, Coleman, DC, Bezdicek, DF, Stewart, BA, pp. 107–124. Soil Science Society of America, Madison.
- Dick RP, Breakwell DP, Turco RF 1996: Soil enzyme activities and biodiversity measurements as integrative microbiological indicators. In Methods for Assessing Soil Quality, Eds. Doran, JW, Jones, AJ, pp. 247–271. Soil Science Society of America, Madison.
- Dolan MS, Clapp CE, Allmaras RR, Baker JM, Molina JAE 2006: Soil organic carbon and nitrogen in a Minnesota soil as related to tillage, residue and nitrogen management. Soil Till. Res., 89, 221–231. doi:10.1016/j.still.2005.07.015
- Dube E, Chiduza C, Muchaonyerwa P 2012: Conservation agriculture effects on a Haplic Cambisol after four years of maize-oat and maize-grazing vetch rotations in South Africa. Soil Till. Res., 123, 21–28. doi:10.1016/j.still.2012.02.008
- Fanadzo M 2007: Weed management by small-scale irrigation farmers – the story of Zanyokwe. SA Irrigation, 29, 20–24.
- Fey M 2010: Soils of South Africa, Cambridge University Press, Cambridge.
- Fox RC, Rowntree KM 2001: Redistribution, restitution and reform prospects for the land in the Eastern Cape Province, South Africa. Land Degrad, 58, 167–186.
- Franzluebbers AJ 2002: Soil organic matter stratification ratio as an indicator of soil quality. Soil Till. Res., 66, 95–106. doi:10.1016/S0167-1987(02)00018-1
- Franzluebbers AJ, Arshad MA 1997: Particulate organic carbon content and potential mineralisation as affected by tillage and texture. Soil Sci. Soc. Am. J., 61, 1382–1386.
- Garcia C, Hernandez T, Costa F 1994: Microbial activity in soils under Mediterranean environmental conditions. Soil Biol. Biochem., 26, 1185–1191. doi:10.1016/0038-0717(94)90142-2
- Gregorich EG, Carter MR, Angers DA, Monreal CM, Ellert BH 1994: Towards a minimum data set to assess soil organic matter quality in agricultural soils. Can. J. Soil Sci., 74, 367–385. doi:10.4141/cjss94-051
- Haynes RJ, Francis GS 1993: Changes in microbial C, soil carbohydrates composition and aggregate stability induced by growth of selected crop and forage species under field conditions. J. Soil Sci., 44, 665–675. doi:10.1111/j.1365-2389.1993.tb02331.x
- Herbert BE, Bertsch PM 1995: Characterization of dissolved and colloidal organic matter in soil solution: a review. In Carbon Forms and Functions in Forest Soils, Eds. McFee, WW, Kelly, JM, pp. 63–88. Soil Science Society of America, Gainesville, FL.
- Klose S, Tabatabai MA 2000: Urease activity of microbial biomass in soil. Biol. Fert. Soil, 6, 68–72.
- Laker MC 2004: Soils and environment; the past 25 years. S. Afr. J. Plant Soil, 21, 245–268.
- LECO Corporation 2003: Truspec Carbon/Nitrogen determinator. Leco Corporation 3000, Lakeview Avenue St Joseph, USA.
- Mandiringana OT, Mnkeni PNS, Mkile Z, Van Averbeke W, Van Ranst E, Verplancke H 2005: Mineralogy and fertility status of selected soils of the Eastern Cape Province, South Africa. Commun. Soil Sci. Plan., 36, 2431–2446. doi:10.1080/00103620500253514
- Mills AJ, Fey MV 2003: Declining soil quality in South Africa: effects on land use on soil organic matter and surface crusting. S. Afr. J. Sci., 99, 429–436.
- Monreal CM, Bergstrom DW 2000: Soil enzymatic factors expressing the influence of land use, tillage system and texture on soil biochemical quality. Can. J. Soil Sci., 80, 419–428. doi:10.4141/S99-088
- Mukumbareza C, Muchaonyerwa O, Chiduza C 2015: Effects of oats and grazing vetch cover crops and fertilisation on microbial biomass and activity after five years of rotation with maize. S. Afr. J. Plant Soil. doi:10.1080/02571862.2015.1025446
- Mupambwa HA 2012: Winter rotational cover crops effects on soil strength, aggregate stability and water conservation of a hard-setting cambisol in Eastern Cape Province, South Africa. MSc dissertation, University of Fort Hare, Alice.
- Murphy DV, Sparling GP, Fillery IRP 1998: Stratification of microbial biomass C and N and gross N mineralisation with soil depth in two contrasting Western Australian Agricultural soils. Aust. J. Soil Res., 36, 45–55. doi:10.1071/S97045
- Murungu F, Chiduza C, Muchaonyerwa P 2011: Mulch effects on soil moisture productivity in warm-temperate climate of South Africa. Soil Till. Res., 112, 58–65. doi:10.1016/j.still.2010.11.005
- Muzangwa LR, Chiduza C, Muchaonyerwa P 2012: Biomass production, weed suppression, nitrogen and phosphorus uptake in white oat (Avena sativa L.) and grazing vetch (Vicia dasycarpa L.) cover crop bicultures under an irrigated no-till system. S. Afr. J. Plant Soil, 29, 135–141. doi:10.1080/02571862.2012.741719
- Muzangwa LR, Chiduza C, Muchaonyerwa P 2015: Bicultures of oat (Avena sativa) and grazing vetch (Vicia dasycarpa) regulate residue decomposition, nitrogen and phosphorus dynamics, and weed suppression in Maize. Int. J. Agr. Biol., 17, 475–482.
- Nannipieri P, Giagnoni L, Landi L, Renella G 2011: Role of phosphatase enzymes in soil. In Phosphorus in Action, Eds. Bunemann EK, Oberson A, Frossard E, pp. 215–241. Soil Biology 26. Springer Verlag, Berlin.
- Nannipieri P, Giagnoni L, Renella G, Puglisi E, Ceccanti B, Masciandaro G, Fornasier F, Moscatelli MC, Marinari S 2012: Soil enzymology: classical and molecular approaches. Biol. Fert. Soil, 48, 743–762. doi:10.1007/s00374-012-0723-0
- Newell SY, Miller JD, Fallon RD 1987: Ergosterol content of salt marsh fungi: effect of growth conditions and mycelial age. Mycologia, 79, 688–695. doi:10.2307/3807821
- Pascual JA, Garcia C, Hernandez T 1999: Lasting microbiological and biochemical effects of the addition of municipal solid waste to an arid soil. Biol. Fert. Soil, 30, 1–6. doi:10.1007/s003740050579
- Reeves DW 1997: The role of soil organic matter in maintaining soil quality in continuous cropping systems. Soil Till. Res., 43, 131–167. doi:10.1016/S0167-1987(97)00038-X
- Saggar S, Bettany JR, Stewart WB 1981: Measurement of microbial sulphur in soil. Soil Biol. Biochem., 13, 493–498. doi:10.1016/0038-0717(81)90040-7
- Sainju UM, Whitehead WF, Singh BP 2005: Biculture legume-cereal cover crops for enhanced biomass yield and carbon and nitrogen. Agron. J., 97, 1403–1412. doi:10.2134/agronj2004.0274
- Salazar S, Sanchez L, Alvarez J, Valverde A, Galindo P, Igual J, Peix A, Santa-Regina I 2011: Correlation among soil enzyme activities under different forest system management practices. Ecol. Eng., 37, 1123–1131. doi:10.1016/j.ecoleng.2011.02.007
- Sasal MC, Castiglion MG, Wilson MG 2010: Effect of crop sequences on soil properties and run-off on natural-rainfall erosion plots under no tillage. Soil Till. Res., 108, 24–29. doi:10.1016/j.still.2010.03.010
- Soil Classification Working Group 1991: Soil classification: a taxonomic system for South Africa. Memoirs on the Agricultural Natural Resources of South Africa, no. 15. Department of Agriculture Development, Pretoria.
- Tabatabai MA 1994: Soil enzymes. In Methods of Soil Analysis Part 2: Microbiological and Biochemical Properties, Eds. Weaver, RW, Angle, JS, Bottomley, PS, pp. 775–833. Soil Science Society of America, Madison.
- Tabatabai MA, Bremner JM 1972: Assay of urease activity in soils. Soil Biol. Biochem., 4, 479–487.
- Wilhelm WW, Johnson JMF, Hatfield JL, Voorhees WB, Linden DR 2004: Crop and soil productivity response to corn residue removal: a literature review. Agron. J., 96, 1–17.
- Witt C, Gaunt JL, Galicia CC, Ottow JCG, Neue HU 2000: A rapid chloroform fumigation extraction method for measuring soil microbial biomass carbon and nitrogen in flooded rice soils. Biol. Fert. Soil, 30, 510–519.
- Yuan B, Yue D 2012: Soil microbial and enzymatic activities across a chronosequence of Chinese pine plantation development on the loess plateau of China. Pedosphere, 22, 1–12.
- Zhang N, He X, Gao Y, Li Y, Wang H, Ma D, Zhang R, Yang S 2010: Pedogenic carbonate and soil dehydrogenase activity in response to soil organic matter in Artemisia ordosica community. Pedosphere, 20, 229–235.
- Zhao B, Chen J, Zhang J, Qin S 2010: Soil microbial biomass and activity response to repeated drying-rewetting cycles along a soil fertility gradient modified by long-term fertilization management practices. Geoderma, 160, 218–224.
- Zsolnay A, Steindl H 1991: Geo-variability and biodegradability of the water extractable organic material in an agricultural soil. Soil Biol. Biochem., 23, 1077–1082.