ABSTRACT
Phosphorus (P) deficiency is one of the major constraints to crop yield worldwide, and genotypes or cultivars with high phosphate use efficiency (PUE) sustain growth when exposed to phosphate stress. Therefore, it is imperative to develop the genotypes or cultivars with high PUE. A pot experiment was conducted to evaluate the PUE among 150 barley (Hordeum vulgare L.) genotypes. Two high-tolerant and -sensitive accessions were selected. These two candidate materials were used to investigate the differences among the root morphology characteristics, antioxidant enzyme activity, inorganic phosphate (Pi) content and gene expression of HvPT5 under P-deficiency and P-sufficiency conditions. The values of these parameters were higher in the low-P-tolerant genotype than in the sensitive one. In pot experiment 1, all genotypes showed a significant difference in low-P tolerance, with variety GN121 achieving the highest tolerance, and GN42 being most sensitive. The results of this study may provide elite genetic germplasms for future work on isolation of P-related genes, and the improvement of PUE in barley.
Abbreviations: PUE: phosphate use efficiency; CAT: catalase; POD: peroxidase; SOD: superoxide dismutase; DMSO: dimethyl sulphoxide; MDA: malondialdehyde; TOPSIS: technique for order preference by similarity to an ideal solution; MCDM/MADM: multi-criteria (or attribute) decision making
KEY WORDS:
1. Introduction
Phosphorus (P), a crucial element for plant growth, is absorbed by the root system in the form of inorganic phosphate (Pi) from soil matrices (Marschner and Rimmington Citation1988; Ha and Tran Citation2014; Frydenvang et al. Citation2015). It is one of the major ingredients of compound fertilizers used throughout the world to raise the yield of crops (Okazaki et al. Citation2013; Park et al. Citation2014). Although the productivity of crops is increased significantly by 30–50% with a corresponding increase of fertilizer application rates, its manufacturing is a process of intensive energy (Stewart et al. Citation2005). In addition, a series of environmental problems have been raised as a result of the excessive application of the fertilizer all over the world (Tilman et al. Citation2002; Ju et al. Citation2007; Rosas et al. Citation2015).
An adequate supply of Pi is essential to plant growth. Nevertheless, the utilization rate of Pi in the soil is very low, with the result that plants have evolved many traits to improve their adaptability to different P environments (Vance et al. Citation2003). These modulations cover: (1) increasing the number of roots and changing their architecture; (2) improving P absorption by more P transporters; and (3) enhancing the activity of enzymes related to P utilization (Nilsson et al. Citation2010).
Specifically, phosphate assimilation relies mainly on phosphate transporters (PTs) under the condition of Pi deficiency (Mukatira et al. Citation2001). AtPT5 was reported to regulate Pi between the source and sink tissues (Nagarajan et al. Citation2011; Wu et al. Citation2013; Inoue et al. Citation2014). The expression of OsPT5 indirectly acts on Pi translocation in shoots (Jia et al. Citation2011). TaPT5 was also detectable in both roots and shoots of plants under different Pi treatments (Davies et al. Citation2002). HvPT5, one of the Hvpht1 family members, was used indirectly to evaluate the phosphate use efficiency (PUE) of barley (Hordeum vulgare L.) accessions because the same highly conservative amino acid sequence (GGDYPLSATIMSE) had been found in HvPT5 when compared with other PTs from different species (Liqin Citation2011).
Moreover, reactive oxygen species (ROS) are involved in many signal transduction pathways for nutrient stress (Shin et al. Citation2005). They are also generated and accumulated rapidly under P stress, which is considered to cause extensive oxidative damage to biological macromolecules (Wang et al. Citation2012). Antioxidative enzymes play a vital role in scavenging ROS, especially superoxide dismutase (SOD) and peroxidase (POD) (Guo et al. Citation2005; Lin et al. Citation2013; Fu et al. Citation2014; Grad et al. Citation2014). Malondialdehyde (MDA) (Ding et al. Citation2014) is the end product of lipid oxidation by ROS, and catalase (CAT) (Iannone et al. Citation2015) can transfer two electrons to protect cells from hydrogen peroxide.
Genotypes or cultivars with high nutrient use efficiency sustain growth when exposed to nutrient deficiency due to more nutrient uptake or higher utilization efficiency (Swiader et al. Citation1994). The use efficiency of a nutrient is defined as the ratio of dry biomass per unit nutrient in a particular growth stage (Good et al. Citation2004; Maia et al. Citation2011). A large variation among genotypes in PUE has been found in many crops. Some genotypes are able to promote crop growth and output in the soil with lower P levels, and they are identified as P-deficiency tolerant (Wissuwa and Ae Citation2001). Thus, it is imperative to develop the genotypes or cultivars with high PUE.
Barley (Hordeum vulgare L.) is one of the principal cereal crops all over the world (Consortium Citation2012; Nadira et al. Citation2014). It is used as forage and grain as well as a health food. As industries related to barley are developing rapidly, the importance of barley production has already been highlighted around the world. However, the planting area of barley in China has been reduced in recent years. Compared with rice (Oryza sativa L.) and wheat (Triticum aestivum L.), barley is relatively sensitive to P deficiency. Therefore, it is important to develop barley cultivars with normal growth and biomass production with less fertilizer application.
The objectives of the current research were: to assess low-P tolerance of barley accessions, and identify the accessions with high PUE; to detect the morphological and physiological traits related to low-phosphate tolerance; and to examine the expression level of the gene related to P efficiency.
2. Materials and methods
2.1. Pot experiment 1
A total of 150 barley accessions of different genetic backgrounds were used for the evaluation of low-P tolerance. The experiment was conducted from late March to early July in 2014 at Gansu Agricultural University (Lanzhou, China). The seeds were surface-sterilized in 3% NaClO (sodium hypochlorite) for 20 min, soaked in tap water for 10 min and washed 3 times with tap water to remove NaClO. The seeds were then sprouted on three layers of dampened filter paper in transparent plastic boxes (15 cm × 10 cm × 12 cm), which were placed in a phytotron (22/18°C, day/night). Five days later, six uniform seedlings of each accession, as one repetition, were selected and transplanted into a 5-L plastic pot, which was filled with sand + vermiculite (volume:volume, 1:1) and placed randomly outdoors. The Pi-sufficiency nutrient solution (+P) involved 0.5 mM KH2PO4, and the Pi-inadequate nutrient solution (-P) involved 0.05 mM KH2PO4 (potassium phosphate monobasic) and an additional 0.45 mM KCl (potassium chloride). The composition of full strength nutrient solution (Hoagland, modified) was as follows: macronutrients (mM): KNO3 (potassium nitrate) 1, Ca(NO3)2 (calcium nitrate) 2, CaCl2 (calcium chloride) 1.5, MgSO4 (magnesium sulphate) 0.5, KCl (potassium chloride) 1.8; micronutrients (μM): H3BO3 (Orthoboric acid) 1, MnSO4 (Manganese sulphate) 1, CuSO4 (cupric sulfate) 0.5, ZnSO4 (zinc sulfate) 1, (NH4)6Mo7O24 (ammonium molybdate) 0.1, Na2-EDTA (ethylenediaminetetraacetic acid disodium salt) 50, FeSO4 (ferrous sulfate) 50. The pH was adapted to 6.0 ± 0.1 with NaOH (sodium hydroxide) or HCl (chlorane) as required. The pots were irrigated with 1 L water every 7 d before the booting stage. From booting stage to mature stage, pots were watered with the same amount as before every 3 d. All agronomic managements were implemented equally as per the recommendation.
Seven days after being cultured, two P levels of 0.5 and 0.05 mM were set up for nutrient solution, respectively. The pots were placed in a completely randomized design (CRD) with three replications. The treatment lasted for the whole growth period of barley, and finally the part of plants above ground was mowed for evaluation of low-P tolerance.
2.2. Pot experiment 2 and hydroponic
Based on the results obtained in pot experiment 1, two cultivated barley genotypes, including a low-P-tolerant one (GN121) and a sensitive one (GN42) were selected. The hydroponic experiment was performed in a phytotron which was set at 20 ± 1°C, 50~70% relative humidity and a photon fluence rate of 300 μmol photons m−2 s−1 with a 16 h day/8 h night cycle. The nutrient solution and P levels were the same as those used in pot experiment 1, but 20-L containers were used here. For the evaluation of plant growth in hydroponic experiment 1, the plant materials were randomly planted in normal (0.5 mM KH2PO4) and low (0.05 mM KH2PO4) P nutrient solutions for 30 d. The roots and shoots were sampled to measure dry weight and root morphological parameters every 10 d. In order to detect the quantity of gene expression (hydroponic experiment 2), plant materials were cultured in normal-P (0.5 mM KH2PO4) and low-P (0.05 mM KH2PO4) nutrient solutions for a week, and seedlings were exposed to the above nutrient solution with 0.05 mM P, while roots were sampled at 0, 6, 24, 48 and 72 h, and frozen in liquid nitrogen immediately. Physiological indicators and the total Pi of seedlings were determined every 30 d.
2.3. Evaluation on low-P tolerance
In pot experiment 1, plant height, stem diameter, ear kernel number, spike length and total spike weight were measured from nine representative plants, and three of them were regarded as one replication. Thousand-kernel weight was calculated on the basis of 500 grains dry weight (Wang et al. Citation2013). The PUE is the ratio of shoot P accumulation to aboveground biomass (Berendse and Aerts Citation1987; Hammond et al. Citation2009).
2.4. Root modulus measurement
Morphological modulus of roots was estimated in hydroponic experiment 1. Roots were scanned with an Epson scanner (Epson, Long Beach, CA, USA). The total length, volume and surface area of roots were tested with WinRHIZO software (Quebec, QC, CAN) (Wang et al. Citation2013). The shoots and root biomass of sampled plants were separated and dried at 80°C for 72 h until they gained constant mass.
2.5. Measurement of physiological indicators and total Pi in plant samples
5% (weight/volume) trichloroacetic acid was used to extract MDA, and the analytical method was in conformity with previous research (Heath and Packer Citation1968). MDA content is regarded as one of the most crucial indicators of lipid peroxidation. Conductivity of the medium containing leaf segments was determined directly using a conductivity meter (Suntex conductivity meter, Chinese Taipei). The conductivity of the medium containing no leaf segments was used for correction.
In terms of enzyme extraction, 0.1 M sodium phosphate buffer (pH 6.8) was used to treat pre-cooled leaf tissues, with a chilled mortar and pestle. CAT activity was analyzed by testing the initial disappearance rate of H2O2 (hydrogen peroxide). The reduction in H2O2 was followed along with the fall in optical density at 240 nm, and the activity was figured using the extinction coefficient (40 mM−1 cm−1 at 240 nm) for H2O2 (Kato and Shimizu Citation1987). POD activity was estimated according to Mahan (Citation1994). The activity was computed though the extinction coefficient (22.6 mM−1 cm−1 at 470 nm). SOD was estimated on the basis of the method developed by Paoletti et al. (Citation1986).
Dimethyl sulphoxide (DMSO) was used to extract chlorophyll (Chl) according to Hiscox and Israelstam (Citation1979; see also Wang et al. Citation2013). Arnon’s (Citation1949) formula was adopted: Chla (g·l−1) = 0.0127 A663-0.00269 A645; Chlb (g·l−1) = 0.0229 A645-0.00468 A663; tot Chl (g·l−1) = 0.0202 A645 + 0.00802 A663.
To measure total Pi, the materials were dried at 80℃ for 72 h to measure the dry weight. Plant samples were then digested with concentrated H2SO4 (sulfuric acid) and H2O2 after milling for the determination of Pi content in leaves (Murphy and Riley Citation1962; Wang et al. Citation2013).
2.6. Real-time PCR analysis of HvPT5
Total RNA was isolated using a Trizol regent (Life Technologies, Cat. No. 15596–018) kit following the manufacturer’s instruction. The first-strand cDNA was synthesized according to the instruction with a PrimeScript® RT reagent Kit (TaKaRa, Dalian, China). Real-time polymerase chain reaction (PCR) was performed on a Mastercyclerw ep realplex machine (Eppendorf, Hamburg, Germany) using the SYBRw Premix Ex Taq TM II (TaKaRa, Cat. No. RR091A) (Wang et al. Citation2013) with two sets of primers: 5ʹ-GCCGTGCTTTCCCTCTATG-3ʹ and 5ʹ-GCTTCTCCTTGATGTCCCTTA-3ʹ for ACTIN gene (AY145451), and 5ʹ-TCGGGTTCCTCTACGCGG-3ʹ and 5ʹ-CGCAGCGAGCACATACAACG-3ʹ for HvPT5 (AY187021). The reactions were incubated at 95℃ for 3 min, followed by 40 cycles of 95℃ for 5 seconds, 60℃ for 60 seconds and 72℃ for 30 seconds. The quantities of relative expression for each gene were computed using the comparative ΔCt method with normalization to the internal control gene (Hébert et al. Citation2009). All quantitative PCR data showed average value (standard error) with three independent biological samples.
2.7. Statistical analysis
In this multi-criteria (or attribute) decision-making (MCDM/MADM) problem, the technique for order preference by similarity to an ideal solution (TOPSIS), one of the MCDM methods raised by Yoon and Hwang (Citation1995) was introduced to choose the hithermost from the active ideal solution and the outmost from the passive ideal solution. A normalized decision matrix was established first. The ideal and negative-ideal solutions were obtained to calculate the separation measures of each alternative after weighting the matrix. Finally, the alternatives were ranked in the order of the relative closeness to the ideal solution (Jahanshahloo et al. Citation2006; Wang and Elhag Citation2006). All of the statistical operations were processed by IBM SPSS Statistics 20.0.
3. Results
3.1. The difference in low-P tolerance among accessions
Previous research indicated that low-P condition had an obvious influence on plant height (Cai et al. Citation2012), stem diameter (Melton and Dufault Citation1991), grain number of the primary spike (Rahman and Wilson Citation1977), thousand-grain weight (Arif et al. Citation2006), spike weight (Lázaro et al. Citation2010), spike length (Afzal and Bano Citation2008) and PUE (Wang et al. Citation2010). There were significant differences among the 150 barley accessions in their response to low-P stress. The cluster analysis based on the results obtained by the TOPSIS method showed that the 150 barley accessions could be classified into two different categories (). Compared with the control, the plant height, stem diameter, grain number of the primary spike, thousand-grain weight, total spike weight, and spike length of GN121 declined by 0.86, 2.50, 4.17, 4.31, 3.79 and 1.45%, respectively, and these measurements of GN42 fell sharply by 10.37, 15.39, 31.25, 27.03, 40.74 and 51.97%, respectively (). Yet the PUE of GN121 increased by 15.52% and that of GN42 decreased by 17.41% compared with their control groups (). For these morphological indicators, the performance of GN42 decreased dramatically, but GN121 showed a slight decline except for the thousand-grain weight and PUE under the two treatments. Furthermore, the thousand-grain weight () of GN42 was more reduced than that of GN121. In addition, the TOPSIS method was used for evaluation and ranking (see Supplemental material). Thus, GN121 and GN42 were selected as low-P-tolerant and -sensitive varieties for the next study, respectively.
Figure 1. A cluster dendrogram of 150 barley (Hordeum vulgare L.) accessions based on the results obtained by the TOPSIS method.
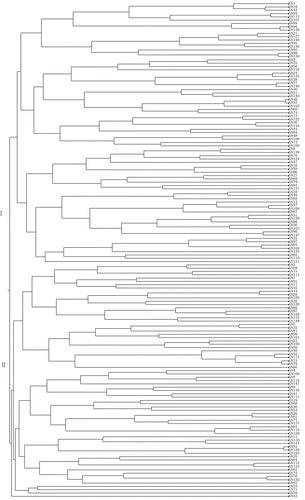
Figure 2. Economical characters of two barley (Hordeum vulgare L.) genotypes under normal and low phosphorus (P) levels after harvests (A: plant height, B: stem diameter, C: spike length, D: grain number of spike, E: total spike weight, F: thousand-grain weight, G: PUE). +P: normal P level; −P: P deficiency stress. Values are mean±standard deviation from three independent biological replicates. Lower case letters represent significant differences at the levels of P = 0.05.
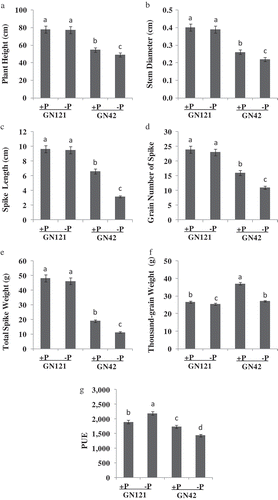
3.2. Root morphological characteristics
Root is the first organ that is mostly affected by the P-stress signal. Therefore, different P-efficiency genotypes respond differently to P stress on the first performance in the root (Niu et al. Citation2012). The greater the contact between roots and soil is, the larger the absorptive area will be, which is beneficial to developing larger root systems and crucial to P uptake (Elanchezhian et al. Citation2015). Furthermore, the absorption and utilization of P complement each other (López-Arredondo et al. Citation2014). In the hydroponic experiment 1, two cultivated barley genotypes were compared under two P levels. The results showed that their growth was obviously inhibited by the low P level, and the symptoms of P deficiency varied between genotypes. In addition, GN42 showed more severe symptoms than GN121 did. In term of root:shoot ratio, root volume, root surface area, total root length, and straw and root dry weight, GN42 showed the maximum reduction of 8.63, 37.86, 18.87, 12.37, 25.47 and 29.93% in turn under the low P level relative to normal conditions (the control), while GN121 was relatively less affected (). This indicates that the low-P tolerance of GN121 was higher than that of GN42.
Table 1. Dry weight and root morphological parameters of two barley (Hordeum vulgare L.) genotypes under normal and low phosphorus (P) levels. +P: normal P level; −P: P deficiency stress. Values are mean ± standard deviation from three independent biological replicates. Lower case letters represent significant differences at the levels of P = 0.05.
3.3. Physiological characteristics
3.3.1. MDA contents
The MDA is the production of lipid peroxide inside cells (Qiu et al. Citation2013). Its content could reflect the degrees of the lipid peroxide of organism and was enhanced under P-deficiency stress. With the extension of P-deficiency stress time, MDA contents of barley leaves varied among different genotypes, with less increase in the high-P-efficient genotype GN121 than in the low-P-efficient genotype GN42. As shown in , MDA contents of GN121 and GN42 increased most by 2.68% and 24.61% in comparison with the control after 90 d stress, respectively. So GN42 would be more sensitive to Pi than GN121 is.
Table 2. Chlorophyll and malondialdehyde (MDA) content of two barley (Hordeum vulgare L.) genotypes under normal and low phosphorus (P) levels. +P: normal P level; −P: P deficiency stress. Values are mean ± standard deviation from three independent biological replicates. Lower case letters represent significant differences at the levels of P = 0.05. FW: fresh weight.
3.3.2. Activity of SOD
The SOD activity is one of the important indicators of plant stress resistance (Oliveira et al. Citation2014), which plays a crucial role in protecting the cell against the oxygen damage. Following the extension of stress time, the SOD activity of young barley leaves continuously dropped under P-deficiency stress condition (). Also, the SOD activity of GN121 and GN42 decreased dramatically by 1.88% and 18.53% as compared with the control after 90 and 60 d stress, respectively. It was obvious that the decreased extent of the SOD activity in GN42 was more remarkable than that of GN121. Therefore, one low-P-tolerant genotype could adapt to low-P stress by keeping a higher level of the SOD activity to alleviate lesion degrees of the cell membrane.
Table 3. Protective enzymes activities of two barley (Hordeum vulgare L.) genotypes under normal and low phosphorus (P) levels. U (unit of enzyme activity): the amount of enzyme completely reacted with 1 μmol substrate during 1 min under the standard condition. +P: normal P level; −P: P deficiency stress; SOD: superoxide dismutase; POD: peroxidase; CAT: catalase. Values are mean ± standard deviation from three independent biological replicates. Lower case letters represent significant differences at the levels of P = 0.05.
3.3.3. Activity of POD
POD is also an important protective enzyme, which can protect cell membranes against lipid peroxidation (Fu et al. Citation2014). POD activity of barley young leaves continuously declined under P-deficiency stress condition (). As stress time went on, the POD activity progressively increased and then decreased when treated in different conditions, which reached peak on the 60th day. Moreover, the POD activities of GN121 and GN42 markedly declined by 1.70% and 9.71% compared with the control at 90 and 60 d stress, respectively. Consequently, the effect of low-P stress on GN42 was very remarkable, while GN121 showed a good performance.
3.3.4. Activity of CAT
CAT provides a cellular defense mechanism by scavenging O2-, which constitutes one of the primary defense systems of cells against oxidative stress (Chen et al. Citation2015). P-deficiency stress was also conducive to inhibiting the activities of CAT, which was much higher in GN121 at a given level of treatment in comparison with GN42 (). The results showed that GN121 could be more effective in scavenging the ROS. The CAT activities of GN121 and GN42 decreased by 3.42% and 29.21% more than the control at 90 d stress, respectively. As a result, the low-P stress had little impact on GN121 compared with GN42.
3.3.5. Chlorophyll content
Chlorophyll can capture the energy from the sun and assimilate CO2. A higher content of chlorophyll means stronger photosynthesis of the plant, within certain realms. A large proportion of chlorophyll a and all chlorophyll b has the ability to collect solar energy, but a few chlorophyll a in a particular condition can convert the energy of sunlight into electric energy (Melis Citation2009). From , the contents of chlorophyll a, b and a + b decreased apparently under P-deficiency stress. Besides, the chlorophyll content of GN121 was clearly higher than that of GN42. This illustrated indirectly that the luminous energy and electron transfer efficiency of GN121 were stronger than those of GN42.
3.4. Pi content
Over the length of the growing season, the total changing trend of the Pi content first increased and then decreased (Zambrosi et al. Citation2015). The Pi content of barley gradually decreased under P-deficiency stress (). Each treatment of GN42 had a significant difference (P < 0.01) compared with GN121, and the Pi content reached maximum value on the 60th day. Meanwhile, the Pi content of GN121 and GN42 decreased most, by 5.54% and 45.92% compared with the control after 60 d stress, respectively. With the treatment on the same terms, the declining degree of the P-efficiency variety was smaller than that of the P-inefficiency variety.
Figure 3. Inorganic phosphate (Pi) concentration of two barley (Hordeum vulgare L.) genotypes under normal and low P levels. +P: normal P level; −P: P deficiency stress; PC: inorganic phosphate content; DW: dry weight. Values are mean ± standard deviation from three independent biological replicates. Lower case letters represent significant differences at the levels of P = 0.05.
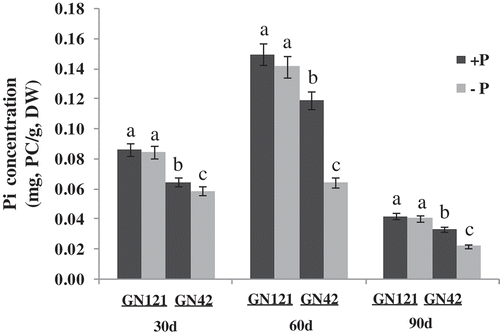
3.5. Expression of phosphate-related genes
The expression pattern of HvPT5 was investigated in the roots of two barley genotypes. It was up-regulated in GN121 under the low P level, increasing by 3.7-, 3.9-, 4.6- and 5.3-fold at 6, 24, 48 and 72 h relative to the control, respectively (). In contrast, it was down-regulated in GN42 under Pi deprivation, decreasing by 0.7-, 0.9-, 0.2- and 0.3-fold at 6, 24, 48 and 72 h relative to the control, respectively. The level of relative expression varied with stress time, and GN121 reached the largest expression peak at 72 h while the smallest expression of GN42 appeared at 48 h after exposure to low-P stress.
Figure 4. Relative expression analysis of HvPT5 in roots by qRT-PCR (quantitative reverse transcriptase polymerase chain reaction). +P: normal P level; −P: P deficiency stress. The y-axis indicates the relative expression level of HvPT5; values are mean±standard deviation from three independent biological replicates. Lower case letters represent significant differences at the levels of P = 0.05.
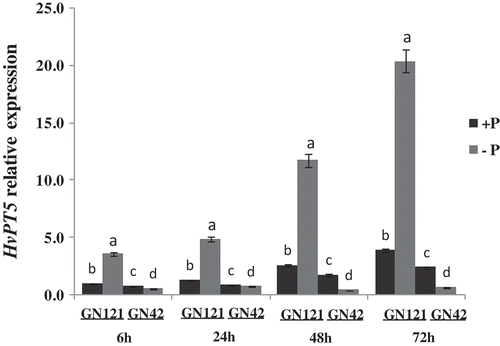
4. Discussion
Barley is one of the world’s principal cereal grains. Because of its wide adaptability, barley has evolved rich genetic variation, particularly in abiotic stress tolerance. In view of its wide distribution between latitudes 70N and 40S, and vigorous growth anywhere, it may be assumed that there are special mechanisms of nutrient uptake and utilization for ensuring normal growth under low fertility in barley, including P deficiency (Adlassnig et al. Citation2012). In this study, we identified one barley accession with high tolerance to low P.
The performance of the plants is roughly the same when they are exposed to nutrition deficiency. A recent study showed the zinc (Zn) efficiency of maize (Zea mays L.) to be related to shoot Zn content and concentration, the activity of SOD, plant height and root dry weight (Karim et al. Citation2012). Similarly, based on agronomic traits under the low-P and normal-P (control) levels, GN121 and GN42 were chosen from pot experiment 1 and used in pot experiment 2 and hydroponic for further study. Under low-P stress, GN42 showed severe P-inadequate symptoms and its economic characteristics were affected, while GN121 was less affected in both morphology and economic characteristics (; ). The result of the pot experiment was more proper and closer to that of field production systems when compared with screening at the seedling stage (Li et al. Citation2005). P deficiency may inhibit the formation of root morphology (López-Bucio et al. Citation2002). Similar to the results of previous research (Li et al. Citation2014), the structure of the root system of GN121 was much greater than that of the sensitive genotype GN42 under the low-P level in the current study ().
Under the low-P level, the Pi content of GN121 and GN42 decreased to various extents, and the content of Pi in shoots was 5.54% and 45.92% under the normal P condition, respectively. Meanwhile, the Pi content of GN121 was always higher than that of GN42 regardless of the time of P treatment, suggesting that the phosphate uptake and transportation efficiency of GN121 were higher than those of GN42. In short, the performance of GN42 was very poor among 150 accessions under P-deficiency conditions, including root system, shape indicators and gene expression. However, it could be a typical material involved in the next stage of the research. In the present study, the activities of protective enzymes (Desai et al. Citation2014) and chlorophyll content were used as important evaluation or screening indicators for the low-P-tolerance ability of barley during its whole growth phase. They differed among many barley genotypes, and decreased markedly under the low P level except the MDA content, and similar results were found in previous studies (Zhu et al. Citation2012; Zhang et al. Citation2014). This shows that plants have developed elaborate mechanisms to survive abiotic stresses.
5. Conclusions
The current study identified some barley accessions with high tolerance to low P. The Pi content, root morphological characteristics and expression of HvPT5 were significantly higher in tolerant barley accessions than in sensitive barley genotypes. All of these characteristics might be regarded as adaptations to P-deficiency environments, by changing the root morphology for P acquisition to meet their needs. This study provided basic knowledge on morphological, physiological and molecular traits of different P-efficient barley genotypes, which is required for future work on isolation of genes related to P and improvement of PUE in barley.
Acknowledgements
This work was supported by the National Basic Research Program of China [973 program, 2014CB160313]; the National Natural Science Foundation of China [nos. 31171558, 31460347]; and the China Agriculture Research System [CARS-05].
Supplementary material
Supplementary material for this article is available online from
References
- Adlassnig W, Koller‐Peroutka M, Bauer S, Koshkin E, Lendl T, Lichtscheidl IK 2012: Endocytotic uptake of nutrients in carnivorous plants. Plant J., 71, 303–313. doi:10.1111/j.1365-313X.2012.04997.x
- Afzal A, Bano A 2008: Rhizobium and phosphate solubilizing bacteria improve the yield and phosphorus uptake in wheat (Triticum aestivum). Int. J. Agric. Biol., 10, 85–88. https://www.researchgate.net/publication/228684375 (January, 2008).
- Arif M, Chohan MA, Ali S, Gul R, Khan S 2006: Response of wheat to foliar application of nutrients. Am. J. Agr Bio Sci., 1, 30–34. https://www.researchgate.net/publication/236620915 (January, 2006).
- Arnon DI 1949: Copper enzymes in isolated chloroplasts. Polyphenoloxidase in beta vulgaris. Plant Physiol., 24, 1–15. http://www.ncbi.nlm.nih.gov/pmc/articles/PMC437905/pdf/plntphys00263-0011.pdf (January, 1949).
- Berendse F, Aerts R 1987: Nitrogen-use-efficiency: a biologically meaningful definition? Funct. Ecol. doi:10.2307/2389434
- Cai H, Chu Q, Gu R et al. 2012: Identification of QTLs for plant height, ear height and grain yield in maize (Zea mays L.) in response to nitrogen and phosphorus supply. Plant Breeding., 131, 502–510. doi:10.1111/j.1439-0523.2012.01963.x
- Chen S, Ding G, Wang Z, Cai H, Xu F 2015: Proteomic and comparative genomic analysis reveals adaptability of Brassica napus to phosphorus-deficient stress. J. Proteomics., 117, 106–119. doi:10.1016/j.jprot.2015.01.012
- Consortium IBGS 2012: A physical, genetic and functional sequence assembly of the barley genome. Nature., 491, 711–716. doi:10.1038/nature11543
- Davies T, Ying J, Xu Q, Li Z, Li J, Gordon‐Weeks R 2002: Expression analysis of putative high‐affinity phosphate transporters in Chinese winter wheats. Plant Cell Environ., 25, 1325–1339. doi:10.1046/j.1365-3040.2002.00913.x
- Desai S, Naik D, Cumming JR 2014: The influence of phosphorus availability and Laccaria bicolor symbiosis on phosphate acquisition, antioxidant enzyme activity, and rhizospheric carbon flux in Populus tremuloides. Mycorrhiza, 24, 369–382. doi:10.1007/s00572-013-0548-1
- Ding Y, Feng R, Wang R, Guo J, Zheng X 2014: A dual effect of Se on Cd toxicity: evidence from plant growth, root morphology and responses of the antioxidative systems of paddy rice. Plant Soil., 375, 289–301. doi:10.1007/s11104-013-1966-8
- Elanchezhian R, Krishnapriya V, Pandey R, Rao AS, Abrol YP 2015: Physiological and molecular approaches for improving phosphorus uptake efficiency of crops. Curr. Sci. India., 108, 1271. http://www.currentscience.ac.in/cs/Volumes/108/07/1271.pdf (April, 2015)
- Frydenvang J, van Maarschalkerweerd M, Carstensen A et al. 2015: Sensitive detection of phosphorus deficiency in plants using chlorophyll a fluorescence. Plant Physiol., 169, 353–361. doi:10.1104/pp.15.00823
- Fu YQ, Yang XJ, Shen H 2014: The physiological mechanism of enhanced oxidizing capacity of rice (Oryza sativa L.) roots induced by phosphorus deficiency. Acta Physiol. Plant., 36, 179–190. doi:10.1007/s11738-013-1398-3
- Good AG, Shrawat AK, Muench DG 2004: Can less yield more? Is reducing nutrient input into the environment compatible with maintaining crop production? Trends Plant Sci., 9, 597–605. doi:10.1016/j.tplants.2004.10.008
- Grad LI, Yerbury JJ, Turner BJ et al. 2014: Intercellular propagated misfolding of wild-type Cu/Zn superoxide dismutase occurs via exosome-dependent and-independent mechanisms. P Natl. Acad. Sci. USA, 111, 3620–3625. doi:10.1073/pnas.1312245111
- Guo Z, Tan H, Zhu Z, Lu S, Zhou B 2005: Effect of intermediates on ascorbic acid and oxalate biosynthesis of rice and in relation to its stress resistance. Plant Physiol. Bioch., 43, 955–962. doi:10.1016/j.plaphy.2005.08.007
- Ha S, Tran L-S 2014: Understanding plant responses to phosphorus starvation for improvement of plant tolerance to phosphorus deficiency by biotechnological approaches. Crit. Rev. Biotechnol., 34, 16–30. doi:10.3109/07388551.2013.783549
- Hammond JP, Broadley MR, White PJ et al. 2009: Shoot yield drives phosphorus use efficiency in Brassica oleracea and correlates with root architecture traits. J. Exp. Bot., 60, 1953–1968. doi:10.1093/jxb/erp083
- Heath RL, Packer L 1968: Photoperoxidation in isolated chloroplasts: I. Kinetics and stoichiometry of fatty acid peroxidation. Arch. Biochem Biophy., 125, 189–198. doi:10.1016/0003-9861(68)90654-1
- Hébert SS, Horré K, Nicolaï L et al. 2009: MicroRNA regulation of Alzheimer’s Amyloid precursor protein expression. Neurobiol. Dis., 33, 422–428. doi:10.1016/j.nbd.2008.11.009
- Hiscox JD, Israelstam G 1979: A method for the extraction of chlorophyll from leaf tissue without maceration. Can. J. Bot., 57, 1332–1334. doi:10.1139/b79-163
- Iannone MF, Groppa MD, Benavides MP 2015: Cadmium induces different biochemical responses in wild type and catalase-deficient tobacco plants. Environ. Exp. Bot., 109, 201–211. doi:10.1016/j.envexpbot.2014.07.008
- Inoue Y, Kobae Y, Omoto E et al. 2014: The soybean mycorrhiza-inducible phosphate transporter gene, GmPT7, also shows localized expression at the tips of vein endings of senescent leaves. Plant Cell Physiol., 55, 2102–2111. doi:10.1093/pcp/pcu138
- Jahanshahloo GR, Lotfi FH, Izadikhah M 2006: An algorithmic method to extend TOPSIS for decision-making problems with interval data. Appl. Math. Comput., 175, 1375–1384. doi:10.1016/j.amc.2005.08.048
- Jia H, Ren H, Gu M et al. 2011: The phosphate transporter gene OsPht1; 8 is involved in phosphate homeostasis in rice. Plant Physiol., 156, 1164–1175. doi:10.1104/pp.111.175240
- Ju X, Kou C, Christie P, Dou Z, Zhang F 2007: Changes in the soil environment from excessive application of fertilizers and manures to two contrasting intensive cropping systems on the North China Plain. Environ. Pollut., 145, 497–506. doi:10.1016/j.envpol.2006.04.017
- Karim M, Zhang YQ, Tian D, Chen FJ, Zhang FS, Zou CQ 2012: Genotypic differences in zinc efficiency of Chinese maize evaluated in a pot experiment. J. Sci. Food Agr., 92, 2552–2559. doi:10.1002/jsfa.5672
- Kato M, Shimizu S 1987: Chlorophyll metabolism in higher plants. VII. Chlorophyll degradation in senescing tobacco leaves; phenolic-dependent peroxidative degradation. Can. J. Bot., 65, 729–735. doi:10.1139/b87-097
- Lázaro L, Abbate P, Cogliatti D, Andrade F 2010: Relationship between yield, growth and spike weight in wheat under phosphorus deficiency and shading. J. Agr Sci-Cambridge., 148, 83–93. doi:10.1017/S0021859609990402
- Li H, Ma Q, Li H, Zhang F, Rengel Z, Shen J 2014: Root morphological responses to localized nutrient supply differ among crop species with contrasting root traits. Plant Soil., 376, 151–163. doi:10.1007/s11104-013-1965-9
- Li Y, Luo A, Wang W, Yang C, Yang X 2005: [An approach to the screening index for low phosphorus tolerant rice genotype]. Ying yong sheng tai xue bao= The journal of applied ecology/Zhongguo sheng tai xue xue hui, Zhongguo ke xue yuan Shenyang ying yong sheng tai yan jiu suo zhu ban, 16, 119–124. (in Chinese).
- Lin H-J, Gao J, Zhang Z-M et al. 2013: Transcriptional responses of maize seedling root to phosphorus starvation. Mol. Biol. Rep., 40, 5359–5379. doi:10.1007/s11033-013-2636-x
- Liqin L 2011: Bioinformatics analysis of Pht1 phosphate transporter protein family in crops. Crops, 3, 006. (in Chinese). http://wenku.baidu.com/link?url=pv5e4ZsTW8XsfR39liCYeujsnO7X8LEbWruX_NeWZ4UNt2MjVIuSMw9om-_uKDbnp_BAZ8HPc46ajx_6KwrZjf4OhGdnb1LJuIQ2o12boGK (March, 2011).
- López-Arredondo DL, Leyva-González MA, González-Morales SI, López-Bucio J, Herrera-Estrella L 2014: Phosphate nutrition: improving low-phosphate tolerance in crops. Annu. Rev. Plant Biol., 65, 95–123. doi:10.1146/annurev-arplant-050213-035949
- López-Bucio J, Hernández-Abreu E, Sánchez-Calderón L, Nieto-Jacobo M, Simpson J, Herrera-Estrella L 2002: Phosphate availability alters architecture and causes changes in hormone sensitivity in the Arabidopsis root system. Plant Physiol., 129, 244–256. doi:10.1104/pp.010934
- Mahan JR 1994: Thermal dependence of glutathione reductase; thermal limitations on antioxidant protection in plants. Crop Sci., 34, 1550–1556. doi:10.2135/cropsci1994.0011183X003400060025x
- Maia C, DoVale JC, Fritsche-Neto R, Cavatte PC, Miranda GV 2011: The difference between breeding for nutrient use efficiency and for nutrient stress tolerance. Crop Breed. Appl. Biot., 11, 270–275. http://www.scielo.br/pdf/cbab/v11n3/a10v11n3.pdf (July, 2011).
- Marschner H, Rimmington G 1988: Mineral nutrition of higher plants. Plant Cell Environ., 11, 147–148. doi:10.1111/j.1365-3040.1988.tb01130.x
- Melis A 2009: Solar energy conversion efficiencies in photosynthesis: minimizing the chlorophyll antennae to maximize efficiency. Plant Sci., 177, 272–280. doi:10.1016/j.plantsci.2009.06.005
- Melton RR, Dufault RJ 1991: Nitrogen, phosphorus, and potassium fertility regimes affect tomato transplant growth. HortScience, 26, 141–142. http://hortsci.ashspublications.org/content/26/2/141.full.pdf (February, 1991).
- Mukatira UT, Liu C, Varadarajan DK, Raghothama KG 2001: Negative regulation of phosphate starvation-induced genes. Plant Physiol., 127, 1854–1862. doi:10.1104/pp.010876
- Murphy J, Riley JP 1962: A modified single solution method for the determination of phosphate in natural waters. Anal. Chim Acta., 27, 31–36. doi:10.1016/S0003-2670(00)88444-5
- Nadira UA, Ahmed IM, Zeng J et al. 2014: The changes in physiological and biochemical traits of Tibetan wild and cultivated barley in response to low phosphorus stress. Soil Sci. Plant Nutr., 60, 832–842. doi:10.1080/00380768.2014.949853
- Nagarajan VK, Jain A, Poling MD, Lewis AJ, Raghothama KG, Smith AP 2011: Arabidopsis Pht1; 5 mobilizes phosphate between source and sink organs and influences the interaction between phosphate homeostasis and ethylene signaling. Plant Physiol., 156, 1149–1163. doi:10.1104/pp.111.174805
- Nilsson L, Müller R, Nielsen TH 2010: Dissecting the plant transcriptome and the regulatory responses to phosphate deprivation. Physiol. Plantarum., 139, 129–143. doi:10.1111/j.1399-3054.2010.01356.x
- Niu YF, Chai RS, Jin GL, Wang H, Tang CX, Zhang YS 2012: Responses of root architecture development to low phosphorus availability: a review. Ann. Bot-London., mcs285. doi:10.1093/aob/mcs285
- Okazaki Y, Otsuki H, Narisawa T et al. 2013: A new class of plant lipid is essential for protection against phosphorus depletion. Nat. Commun., 4, 1510. doi:10.1038/ncomms2512
- Oliveira MT, Medeiros CD, Frosi G, Santos MG 2014: Different mechanisms drive the performance of native and invasive woody species in response to leaf phosphorus supply during periods of drought stress and recovery. Plant Physiol. Bioch., 82, 66–75. doi:10.1016/j.plaphy.2014.05.006
- Paoletti F, Aldinucci D, Mocali A, Caparrini A 1986: A sensitive spectrophotometric method for the determination of superoxide dismutase activity in tissue extracts. Anal. Biochem., 154, 536–541. doi:10.1016/0003-2697(86)90026-6
- Park BS, Seo JS, Chua N-H 2014: NITROGEN LIMITATION ADAPTATION recruits PHOSPHATE2 to target the phosphate transporter PT2 for degradation during the regulation of Arabidopsis phosphate homeostasis. Plant Cell., 26, 454–464. doi:10.1105/tpc.113.120311
- Qiu Z-B, Guo J-L, Zhang M-M, Lei M-Y, Li Z-L 2013: Nitric oxide acts as a signal molecule in microwave pretreatment induced cadmium tolerance in wheat seedlings. Acta Physiol. Plant., 35, 65–73. doi:10.1007/s11738-012-1048-1
- Rahman M, Wilson J 1977: Effect of phosphorus applied as superphosphate on rate of development and spikelet number per ear of different cultivars of wheat. Crop Pasture Sci., 28, 183–186. doi:10.1071/AR9770183
- Rosas F, Babcock BA, Hayes DJ 2015: Nitrous oxide emission reductions from cutting excessive nitrogen fertilizer applications. Clim. Change, 1–15. doi:10.1007/s10584-015-1426-y
- Shin R, Berg RH, Schachtman DP 2005: Reactive oxygen species and root hairs in Arabidopsis root response to nitrogen, phosphorus and potassium deficiency. Plant Cell Physiol., 46, 1350–1357. doi:10.1093/pcp/pci145
- Stewart W, Dibb D, Johnston A, Smyth T 2005: The contribution of commercial fertilizer nutrients to food production. Agron. J., 97, 1–6. doi:10.2134/agronj2005.0001
- Swiader J, Chyan Y, Freiji F 1994: Genotypic differences in nitrate uptake and utilization efficiency in pumpkin hybrids 1. J. Plant Nutr., 17, 1687–1699. doi:10.1080/01904169409364840
- Tilman D, Cassman KG, Matson PA, Naylor R, Polasky S 2002: Agricultural sustainability and intensive production practices. Nature, 418, 671–677. doi:10.1038/nature01014
- Vance CP, Uhde‐Stone C, Allan DL 2003: Phosphorus acquisition and use: critical adaptations by plants for securing a nonrenewable resource. New Phytol., 157, 423–447. doi:10.1046/j.1469-8137.2003.00695.x
- Wang J, Sun J, Miao J et al. 2013: A phosphate starvation response regulator Ta-PHR1 is involved in phosphate signalling and increases grain yield in wheat. Ann. Bot-London., 111, 1139–1153. doi:10.1093/aob/mct080
- Wang L, Xu C, Wang C, Wang Y 2012: Characterization of a eukaryotic translation initiation factor 5A homolog from Tamarix androssowii involved in plant abiotic stress tolerance. BMC Plant Biol., 12, 118. doi:10.1186/1471-2229-12-118
- Wang X, Shen J, Liao H 2010: Acquisition or utilization, which is more critical for enhancing phosphorus efficiency in modern crops? Plant Sci., 179, 302–306. doi:10.1016/j.plantsci.2010.06.007
- Wang Y-M, Elhag TMS 2006: Fuzzy TOPSIS method based on alpha level sets with an application to bridge risk assessment. Expert Syst. Appl., 31, 309–319. doi:10.1016/j.eswa.2005.09.040
- Wissuwa M, Ae N 2001: Genotypic variation for tolerance to phosphorus deficiency in rice and the potential for its exploitation in rice improvement. Plant Breeding., 120, 43–48. doi:10.1046/j.1439-0523.2001.00561.x
- Wu P, Shou H, Xu G, Lian X 2013: Improvement of phosphorus efficiency in rice on the basis of understanding phosphate signaling and homeostasis. Curr. Opin. Plant Biol., 16, 205–212. doi:10.1016/j.pbi.2013.03.002
- Yoon KP, Hwang C-L 1995: Multiple Attribute Decision Making: An Introduction, Sage Publications, New York.
- Zambrosi FCB, Ribeiro RV, Marchiori PER, Cantarella H, Landell MGA 2015: Sugarcane performance under phosphorus deficiency: physiological responses and genotypic variation. Plant Soil., 386, 273–283. doi:10.1007/s11104-014-2252-0
- Zhang K, Liu H, Tao P, Chen H 2014: Comparative proteomic analyses provide new insights into low phosphorus stress responses in maize leaves. PloS One., 9, e98215. doi:10.1371/journal.pone.0098215
- Zhu XF, Lei GJ, Jiang T, Liu Y, Li GX, Zheng SJ 2012: Cell wall polysaccharides are involved in P-deficiency-induced Cd exclusion in Arabidopsis thaliana. Planta., 236, 989–997. doi:10.1007/s00425-012-1652-8