ABSTRACT
Behavior of radioactive strontium (Sr2+) in contaminated soils is an important issue in relation to nuclear power plant accidents. The Sr2+ adsorption on kaolinite and its migration in a kaolinite soil were investigated because toxic effects of radioactive Sr2+ have been found to be very severe for living organisms at low Sr2+ concentrations. Adsorption isotherms of Sr2+ on kaolinite at different salt (NaCl) concentration and pH were obtained by the batch method. The calculated distribution coefficients (KD) ranged between 600 and 40,000 L kg−1, which showed a strong preference for the adsorbed phase. The results were used to evaluate the ratio (r) of penetration length of Sr2+ relative to that of water in a model kaolinite soil. When the Sr2+ solution was percolated constantly into the kaolinite soil, the penetration of Sr2+ was delayed strongly at low Sr2+ concentration due to adsorption. The Sr2+ penetration length was only 0.001–0.056 of the water penetration length at pH 6.5 (0.1–10 mmol L−1 NaCl). At pH 4.1 (1 mmol L−1 NaCl) the effect was about 17 times less than at pH 6.5 (1 mmol L−1 NaCl). Under all conditions, the Sr2+ penetration increased when the Sr2+ concentration increased due to the KD decrease. The Sr2+ isotherms could be fitted well to the Langmuir adsorption equation, which indicates that only one site type is involved in the Sr2+ adsorption.
1. Introduction
Strontium (Sr)-90 is one product of the radioactive elements of nuclear fuel materials emitting beta particles. Its half-life is 29 years, and it is harmful to human health (Fetter et al. Citation1988). During the nuclear power plant accidents in Fukushima, Japan, and Chernobyl, Ukraine, large amounts of radioactive species, including 90Sr, were released into the environment (Bondarkov and Oskolkov et al. Citation2011; Steinhauser et al. Citation2013). As the radioactive damage is serious, the environmental fate of Sr2+ must be considered, especially at low Sr2+ concentrations.
Kaolinite is one of the most ubiquitous phyllosilicates in soils, and it has next to the basal plane surface a relatively large edge surface (White and Dixon Citation2002); therefore, Sr2+ adsorption and transport in kaolinite soils deserve specific attention. Some reports consider Sr2+ adsorption on kaolinite on the basis of ion exchange (Wahlberg et al. Citation1965; Parkman et al. Citation1998; Bascetin and Atun Citation2006; Keçeli Citation2015). Sr2+ transport in quartz sand (Rod et al. Citation2010), sediment (Wallace et al. Citation2012) and porous material (Prigiobbe et al. Citation2012) were reported. The distribution coefficient provides information on both adsorption and migration of a component, and is the simplest parameter to characterize the influence of environmental factors on the fate of a pollutant. Thus, the distribution coefficient has been used to characterize Sr–kaolinite systems (Meyer Citation1979; Rafferty et al. Citation1981; Erten et al. Citation1988; Bunde et al. Citation1997; Başçetin and Atun Citation2006; Rani and Sasidhar Citation2012). The distribution coefficient of the Sr–kaolinite system under the given conditions can be determined when the adsorption isotherm of Sr2+ on kaolinite is known. Migration of Sr2+ in kaolinite soil can be conveniently characterized by using the ratio of average penetration length of Sr2+ relative to that of water. This ratio is determined by the distribution coefficient and the solid and solution content in the kaolinite soil (Bolt Citation1978). The migration of Sr2+ is retarded because of the adsorption of Sr2+ to kaolinite soil. This retardation can be quantified by using the parameter of the distribution coefficient. However, there has been little research on Sr2+ penetration length at low Sr2+ concentration compared with water penetration length.
The objectives of the present study are therefore to investigate both the characteristics of Sr2+ adsorption on kaolinite at different salt (NaCl) concentration and pH values with emphasis on low Sr2+ concentrations, and the migration of Sr2+ in a kaolinite soil under these conditions. The Sr2+ distribution coefficients were derived and used to describe the Sr2+ migration. To gain further insight into the mechanism of the adsorption, the Sr2+ adsorption results were analyzed with the Langmuir model. The Langmuir model assumes ideal adsorption behavior in a monolayer, considers the adsorption capacity as an independent parameter and neglects valence effects (Langmuir Citation1918; Rani and Sasidhar Citation2012).
2. Materials and experiments
The kaolinite from Iriki, Kagoshima, was suspended in deionized water, and the fraction with a diameter less than 2 μm was obtained by centrifugation. The obtained fraction was freeze-dried and stored in a closed container. The sample was characterized by using X-ray diffraction apparatus (RINT1200, Rigaku Group of companies, Japan); the kaolinite content was 98.1% and the alunite content was 1.9%.
Three grams of kaolinite powder was put in a 400 mL centrifuge bottle, mixed well with 300 mL of 1 mol L−1 NaCl solution and repeated 3 times to reach a complete exchange. The clay was equilibrated with the prescribed NaCl solution at 0.1, 1 or 10 mmol L−1. This process was repeated until the electrical conductance of the suspension equaled that of the prescribed NaCl solution at 0.1, 1 or 10 mmol L−1. A suitable volume of 0.01 mol L−1 hydrochloric acid (HCl) or sodium hydroxide (NaOH) solution was added to adjust the pH of the Na-kaolinite suspension to 6.5 or 4.1. The final 10 g L−1 Na-kaolinite suspension was kept for further use.
The Sr2+ adsorption experiments were conducted by the batch method using a series of polypropylene centrifuge tubes. Ten milliliters of the 10 g L−1 Na-kaolinite suspension was pipetted into the centrifuge tube. Subsequently, 20 mL of strontium chloride (SrCl2) solution containing the prescribed NaCl concentration (0.1, 1 or 10 mmol L−1) at prescribed pH (4.1 or 6.5) was added to reach an initial Sr2+ concentration in the range of 2 × 10–3 mmol L−1 to 6 × 10–2 mmol L−1. The tubes were shaken for 24 h at room temperature. The pH of the equilibrium suspension was measured and recorded. Triplicate runs were performed for all batch experiments.
The equilibrium suspensions were centrifuged and the supernatants were gathered to determine the Sr2+ concentrations by inductively coupled plasma mass spectrometry (ICP-MS: PerkinElmer SCIEX-ELAN DRC-e). The adsorbed amount of Sr2+ on the Na-kaolinite was calculated by subtracting the amount of Sr2+ in equilibrium solution from the total amount of Sr2+ added initially.
3. Modeling
3.1. Distribution coefficient
The distribution coefficient, KD (L kg−1), describes the distribution of the adsorbate over the solid phase and the solution phase that can be defined as
where QSr is the adsorbed amount of Sr2+ on kaolinite (mmol kg−1) and [Sr] is the equilibrium Sr2+ concentration in the solution (mmol L−1). The larger KD is, the stronger is the accumulation in the adsorbed phase.
3.2. Retardation and penetration length ratio
Let a certain volume of solution containing a concentration [Sr] mmol L−1 of cation Sr2+ at the prescribed NaCl concentration and pH feed to per unit area of kaolinite. The distribution ratio, RD, is a commonly used physical parameter for estimating the retardation of a dissolved pollutant in the case of a solution with pollutant that permeates in a column of soil. Because the retardation is caused by adsorption, RD is related to the partition of the contaminant between the solution and the solid (adsorbed) phase. As KD quantifies the partition, KD and RD are directly related:
where ρb (kg L−1) is the bulk density of the kaolinite soil (mass of kaolinite in the column per unit volume of kaolinite and void) and θ is the volumetric water content of the column of soil per unit soil volume in saturated condition (L L−1 or m3 m−3). For the calculations, the soil bulk density of the hypothetical kaolinite soil has to be assumed to be homogeneous and constant. As the bulk density of natural soil usually ranges from 1.0 to 1.5 kg L−1, a kaolinite soil bulk density of 1.25 kg L−1 is used in this study. From this value, and the density of pure kaolinite of 2.65 kg L−1 (Kuroda et al. Citation2003), the volume fraction of kaolinite in the soil can be obtained: 1.25/2.65 = 0.472. Consequently, the volume fraction of solution in the soil equals 0.528 when the kaolinite soil is saturated, and this implies that under these conditions has reached its maximum value of 0.528. When only the saturation condition is adopted, RD is proportional to KD because the
and ρb are constant.
In the saturated column, the average penetration length, xp, of Sr2+ fed into the soil by the Sr2+ solution is defined by the relation (Bolt Citation1978):
where VF is the feed volume of Sr2+ solution per m2 of soil (m3/m2) and θ is the saturated water content (m3/m3). VF / θ is the average penetration length (m) of water in the soil. The ratio, r, of the average penetration length of Sr2+ to that of water is thus obtained as:
The inverse of r, 1+RD, is the retardation factor which is widely used for analyses of solute transport in soils.
As KD is a positive quantity, RD is positive and this implies that r < 1 and a small r implies a strong Sr2+ retardation. For large RD (RD > 100), i.e., large KD, r is, practically speaking, inversely proportional to RD. We can also assume that θ is a constant unsaturated volumetric water content. However, in order to obtain the largest penetration length ratio, the saturated condition is adopted here.
3.3. Langmuir model
The Langmuir model was used for the adsorption of Sr2+ on kaolinite as follows:
where QSr (mmol kg−1) is the Sr2+ adsorption, QSr,m (mmol kg−1) is the maximum adsorption, KL the Langmuir adsorption constant (L mmol−1) and [Sr] (mmol L−1) is the equilibrium Sr2+ concentration. As the Langmuir model is the theoretically derived adsorption model, we use it for the theoretical consideration.
4. Results and discussion
4.1. The Sr2+ distribution coefficient
The adsorption isotherms of Sr2+ on Na-kaolinite at pH 6.5 and 4.1 and different NaCl concentrations are depicted in as a double logarithmic plot. The measured adsorption increases linearly with slope ≈ 1 at low Sr2+ concentrations, but gradually levels off at higher Sr2+concentrations. Slope ≈ 1 implies a constant adsorption affinity and KD value; the low concentration ranges for which the slope ≈ 1 is the so-called ‘Henry region’; in this range the adsorption is directly proportional to the solution concentration, which can be well explained by the Langmuir model as written in the later part. The dotted lines (slope = 1) are lines with different KD (L kg−1) as indicated (see Equation 1). The constant values of KD at low Sr2+ concentration are observed for each isotherm in . They ranged between 600 and 40,000 L kg−1. The magnitude of KD clearly depends on the solution conditions: KD strongly decreases with increasing salt concentration and decreasing pH. The KD value at low Sr2+ concentration at 0.1 mmol L−1 NaCl is about 53 times larger than that at 10 mmol L−1 NaCl. The effect of pH is only investigated at 1 mmol L−1 NaCl. KD at pH 6.5 is about 17 times larger than KD at pH 4.1. Similar effects for the kaolinite–Sr2+ system have been observed by a few researchers (Meyer Citation1979; Rani and Sasidhar Citation2012). For all solution conditions KD decreases with increasing Sr2+ concentrations at Sr2+ concentrations beyond the Henry region, but the Sr2+ concentration where KD starts to decrease depends on the solution conditions. Erten et al. (Citation1988) and Başçetin and Atun (Citation2006) also observed a decrease of KD with the increasing Sr2+ concentration for kaolinite. Concluding this section, “it is clear that pH and Na+ concentration strongly influence the adsorption of Sr2+ on kaolinite and the Sr2+ distribution coefficient”.
Figure 1. Strontium (Sr2+) adsorption isotherms on kaolinite at two pH values and three salt concentrations.
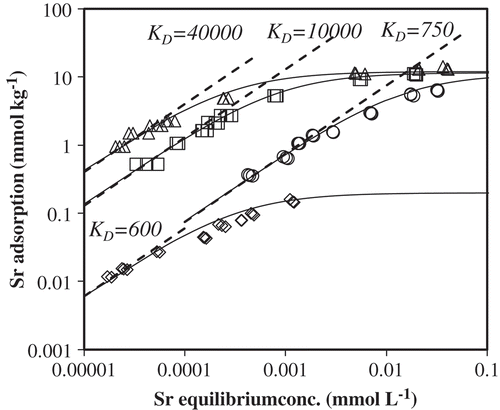
The fittings of the Sr2+ adsorption data to the Langmuir equation are presented in as double logarithmic plot (solid lines). The measured values of Sr2+ adsorbed on kaolinite are quite well fitted to the Langmuir model. The values of the adsorption affinity or Langmuir constant, KL, and the adsorption capacity, QSr,m, are collected in . The values of KL are strongly influenced by NaCl concentration because the influence of competition between Sr2+ and Na+ on KL differs with different Na+ concentration. Thus, KL obtained in this study should be interpreted as a conditional constant; QSr,m are almost identical under different NaCl concentrations.
Table 1. Langmuir isotherm parameters.
By comparing KD and KL at given solution conditions, it should be noted that KL is a constant for all Sr2+ concentrations, but that KD is only constant in the Henry region. The difference is due to the fact that Equation (5) takes into account the adsorption capacity, QSr,m. At relatively high Sr2+ concentration the probability of adsorption is decreased by the decrease of the vacant sites for Sr2+ adsorption. Equation (5) takes this decreased probability into account, while Equation (1) does not take the adsorption capacity into account. At sufficiently low Sr2+ concentration (KL[Sr] ≪ 1) Equation (5) reduces to the Henry equation, QSr = QSr,mKL[Sr], and this relation equals Equation (1) with QSr,mKL = KD, indicating that in the Henry region KL and KD are closely related.
The Sr2+ isotherms could be fitted well to the Langmuir adsorption equation, which indicates that only one site type is involved in the Sr2+ adsorption. Values of KD keeping constant at low Sr2+ concentration were observed. Therefore, even if the Sr2+ concentration is much lower than those in this experiment, the values of KD in the Henry region can be valid from the theoretical viewpoint. The value can be applicable for the 90Sr contaminated sites where the Sr2+ concentration is supposed to be very low.
4.2. The Sr2+ penetration length ratio
The average penetration length ratio r, representing the average penetration length of Sr2+ relative to that of water, is depicted in as a function of the equilibrium Sr2+ concentration of the solution fed in the kaolinite soil. The four depicted data sets correspond with the four adsorption isotherms depicted in . Since the ratio r is inversely proportional to (1 + RD) and RD is proportional to KD, the large KD values corresponding with the isotherms at low Sr2+ concentration induce small r values. As KD increased with decreasing NaCl concentration and increasing pH, the reverse trend should be observed for r and this explains the decrease of r with decreasing NaCl concentration and increasing pH observed in . The small values of r indicate considerable retardation of Sr2+ upon solution penetration in the kaolinite soil. The increase of the penetration length ratio of Sr2+ in kaolinite with increasing Sr2+ concentration is due to the decrease of KD. By considering the above trends quantitatively, the following observations can be made. The dependence of the penetration length ratio of Sr2+ in kaolinite on pH is considerable: at 1 mmol L−1 NaCl and low Sr2+ concentration, the r value at pH 4.1 (7.04 × 10–4) is 16.7 times larger than r at pH 6.5(4.22 × 10–5). It is favorable for Sr2+ adsorption at high pH, which results in a strong retardation of Sr2+ in sediments (Wallace et al. Citation2012) and iron oxides (Prigiobbe et al. Citation2012). The effect of the NaCl concentration at pH 6.5 and low Sr2+ concentration on the penetration length of Sr2+ is also large: at 0.1 mmol L−1 NaCl r equals 1 × 10–5 which is 53.3 times smaller than r at 10 mmol L−1 NaCl (5.63 × 10–4). The trend of the present results corresponds well with literature results. Wallace et al. (Citation2012) have reported that for sediments the presence of Na+ resulted in the much lower sorption of Sr2+ which caused a significant migration of Sr2+ in the sediments. Hull and Schafer (Citation2008) reported that Sr2+ transported rapidly in the vadose zone sediment at high Na+ concentration and the Sr2+ mobility decreased significantly in the absence of competing cations.
Figure 2. The penetration length ratio of Strontium (Sr2+) versus the fed solution concentration Sr2+.
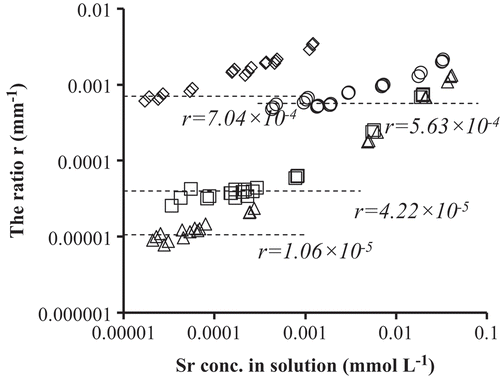
The evaluated penetration length ratios of the Sr2+ at low [Sr] are very small. The Sr2+ penetration length is about 0.001% of the water penetration length at the conditions of pH 6.5, 0.1 mmol L−1 NaCl and [Sr] < 5 × 10−8 mmol L−1. At pH 6.5, 1 mmol L−1 NaCl and [Sr] < 2 × 10−7 mmol L−1, the value is about 0.004%. At pH 6.5, 10 mmol L−1 NaCl and [Sr] < 2 × 10−6 mmol L−1, the value is 0.056%. At pH 4.1, 1 mmol L−1 NaCl and [Sr] < 2 × 10−8 mmol L−1, the value is about 0.07%. These values clearly indicate that Sr2+ is most strongly retarded by kaolinite at low NaCl concentration and relatively high pH.
The values of extremely low penetration length ratio of Sr2+ relative to that of water in kaolinite were calculated theoretically based on the distribution coefficient obtained from adsorption experiment. Although there are many complicated factors affecting the migration of Sr2+ in soils such as preferential flow, the average penetration length in the soils with uniform soil structure can be predicted.
In conclusion, because only one site type is involved in the Sr2+ adsorption in the kaolinite, the constant values of KD are valid to calculate the average penetration length of Sr2+ even at extremely low Sr2+ concentration, theoretically. The low values of average penetration length ratio show the strong retardation in kaolinite soils for Sr2+ at low Sr2+ concentration. Although the penetration ratio is a simple and useful parameter to estimate the mobility of Sr2+, direct observations of Sr2+ migration in soil are also necessary to confirm the theoretically calculated retardation.
Acknowledgments
The authors would like to express gratitude to the China Scholarship Council (CSC) for supporting Zigong Ning’s overseas study. This research was supported by Grants-in-Aid for Scientific Research [grant number 25252042] from the Japan Society for the Promotion of Science.
References
- Başçetin E, Atun G 2006: Adsorption behavior of strontium on binary mineral mixtures of Montmorillonite and Kaolinite. Appl. Radiat. Isotopes, 64 (8), 957–964. doi:10.1016/j.apradiso.2006.03.008
- Bolt GH 1978: Transport and accumulation of soluble soil components. In Soil Chemistry: A. Basic Elements, Ed. Bolt, GH, Bruggenwert, MGM, pp. 126–140. Elsevier, Amsterdam.
- Bondarkov MD, Oskolkov BY et al. 2011: Environmental radiation monitoring in the Chernobyl exclusion zone-history and results 25 years after. Health. Phys., 101, 442–485. doi:10.1097/HP.0b013e318229df28
- Bunde RL, Rosentreter JJ, Liszewski MJ, Hemming CH, Welhan J 1997: Effects of calcium and magnesiumon strontium distribution coefficients. Environ. Geol., 32 (3), 219–229. doi:10.1007/s002540050210
- Erten HN, Aksoyoglu S, Hatipoglu S, Gokturk H 1988: Sorption of cesium and strontium on montmorillonite and kaolinite. Radiochinica. Acta., 44/45 (147–151). doi:10.1524/ract.1988.4445.1.147
- Fetter S, Cheng ET, Mann FM 1988: Long-term radioactivity in fusion reactors. Fusion. Eng. Des., 6 (123), 123–130. doi:10.1016/S0920-3796(88)80098-X
- Hull LC, Schafer AL 2008: Accelerated transport of 90Sr following a release of high ionic strength solution in vadose zone sediments. J. Contam. Hydrol., 97, 135–157. doi:10.1016/j.jconhyd.2008.02.001
- Keçeli G 2015: Adsorption kinetics and equilibria of strontium onto kaolinite. Sep. Sci. Technol., 50, 72–80. doi:10.1080/01496395.2014.955206
- Kuroda Y, Nakaishi K, Adachi Y 2003: Settling velocity and structure of kaolinite floc in sodium chloride solution. Clay Sci., 12 (103–107). doi:10.11362/jcssjclayscience1960.12.103
- Langmuir I 1918: The adsorption of gases on plane surfaces of glass, mica and platinum. J. Am. Chem. Soc., 40 (9), 1361–1403. doi:10.1021/ja02242a004
- Meyer RE 1979: Systematic study of nuclide adsorption on selected geologic media. ONWI Report
- Parkman RH, Charnock JM, Livens FR et al. 1998: A study of the interaction of Sr ions in aqueous solution with the surface of calcite and kaolinite. Gechim. Cosmochim. Acta., 62 (9), 1481–1492. doi:10.1016/S0016-7037(98)00072-6
- Prigiobbe V, Hesse MA, Bryant SL 2012: Fast strontium transport induced by hydrodynamic dispersion and pH-dependent sorption. Geophy. Res. Lett., 39 (18). doi:10.1029/2012GL053146
- Rafferty P, Shiao S, Binz CM, Meyer RE 1981: Adsorption of Sr(II) on clay minerals: effects of salt concentration, loading and pH. J. Inorg. Nucl. Chem., 43, 797–805. doi:10.1016/0022-1902(81)80224-2
- Rani DR, Sasidhar P 2012: Geochemical and thermodynamic aspects of sorption of strontium on kaolinite dominated clay samples at Kalpakkam. Environ. Earth. Sci., 65 (1265–1274). doi:10.1007/s12665-011-1374-4
- Rod KA, Um W, Flury M 2010: Transport of strontium and cesium in simulated Hanford Tank waste leachate through quartz sand under saturated and unsaturated flow. Environ. Sci. Technol., 44 (21), 8089–8094. doi:10.1021/es903223x
- Steinhauser G, Schauer V, Shozugawa K, Aegerter CM 2013: Concentration of Strontium-90 at Selected Hot Spots in Japan. Plos ONE, 8 (3), e57760. doi:10.1371/journal.pone.0057760
- Wahlberg JS, Baker JH, Vernon RW, Dewar RS 1965: Exchange adsorption of strontium on clay minerals. Geol. Surv. Bull., 1140–c.http://pubs.er.usgs.gov/publication/b1140C
- Wallace SH, Shaw S, Morris K, Small JS, Fuller AJ, Burke IT 2012: Effect of groundwater pH and ionic strength on strontium sorption in aquifer sediments: implications for 90Sr mobility at contaminated nuclear sites. Appl. Geochem., 27, 1482–1491. doi:10.1016/j.apgeochem.2012.04.007
- White GN, Dixon JB 2002: Soil Mineralogy with Environmental Application: kaolin-Serpentine Minerals. Soil Science Society of America, Inc. Madison, Wisconsin, USA SSSA Book Series, no.7.