ABSTRACT
Siderite (FeCO3), a type of carbonate mineral, is very occasionally recognized as a nodule in anoxic soils and sediments. During siderite formation, elements are expected to be accumulated or excluded between siderite and bulk soil. Therefore, we verified the affinity of 40 elements for siderite found in the gley horizon of a smectite-rich paddy field in northeastern Japan from the elemental concentration of the soil and the siderite dissolved in 2.88 mol L−1 hydrochloric acid (10% HCl). The concentration ratio of the target element of the soil to the siderite was expressed by the product of the dimensionless R0 value and ratio of titanium (Ti) concentration of the soil to the siderite (Csiderite /Csoil) = R0 (Tisiderite /Tisoil). The affinity of each element for the siderite was analyzed by comparing the R0 values. In comparison with Ti used as the reference element, P, Cr, Co and Mo were accumulated (R0 > 1.0), and alkali metals, such as Li, Na and K, and chalcophile elements, such as Cu, Zn, Cd and Pb, tended to be equal or excluded from the siderite (R0 ≦1.0). Of the group 2 elements, Ca, Sr and Ba were accumulated, while Mg was excluded. The affinity of Mn for siderite exceeded that of Fe as the main component of the siderite, which reflects the fact that siderite was formed in a fresh water condition. The siderite size did not influence the elemental affinity. Observation of micromorphology using a scanning electron microscope showed that the siderite nodules were aggregations of siderite particles and clays, suggesting that the siderite grew by taking in clay around it. The rare earth element (REE) distribution pattern showed a tendency not to increase linearly but to increase with forming a concave curve at the intervals of the four elements with the increase of atomic number (i.e. tetrad effects). Although these findings are from the lower horizons of anoxic soils, the elemental affinity for siderite of this study is useful in order to elucidate the complex elemental dynamics in the anoxic environment.
1. Introduction
After soil is flooded by irrigation water or a rise in the water table, iron (Fe), manganese (Mn), nitrate (NO3−) and sulfate ion (SO42−) in the soil become reduced (Patrick and Reddy Citation1978; Kyuma Citation2004). Since the Fe concentration of typical soil is considerably higher than that of Mn, NO3− and SO42−, morphological changes in the Fe due to the redox process have a significant influence on the chemical properties of the soil (Neubauer et al. Citation2007). For the reduction from trivalent Fe [Fe(III)] to divalent Fe [Fe(II)] in the soil, a few conditions are required, i.e., (1) the presence of organic matter; (2) the absence of oxygen, NO3− and easily reducible Mn oxides; (3) the presence of anaerobic bacteria and an environment suitable for their growth (Breemen and Buurman Citation2002).
Most of the Fe(II) in the anoxic soil is in the state of an exchangeable and solid form, while the movable Fe(II) ion occurs only slightly in a soil solution (Breemen and Buurman Citation2002). However, Fe(II) ion in the soil solution accumulates at the boundary near the oxidative environment, and specific nodules and mottles are formed with specific characteristics affected by changes in the water management or water table (King et al. Citation1990; Kyuma Citation2004). Under a long-lasting anoxic condition in which the water table is continuously high, Fe(II) minerals like siderite, vivianite (Fe3(PO4)2・8H2O), pyrite (FeS2), and Fe(III)–Fe(II) mixed minerals like a magnetite and green rust are produced (Borch et al. Citation2010).
Meanwhile, in order to reveal the element dynamics in the soil, information on the elemental affinity for soil components is needed. Laboratory experiments to evaluate the elemental affinity for soil components including clay, organic matter, humic substances and oxyhydroxide have been conducted (Bunzl et al. Citation1976; Sparks Citation1999; Pandey et al. Citation2000; YoungDo and Park Citation2010). In particular, the affinity of some heavy metals (Cd, Cu, Pb and Zn) for goethite or manganese oxide produced by an oxidation process in the soil has been revealed (McKenze Citation1980; Doyle and Otte Citation1997; Zhang et al. Citation2012). However, actual anoxic soil environment is a semi-open system and the duration elapsed to present is much longer than in the laboratory experiments. Therefore, the elemental affinity for components peculiar to anoxic soil is not completely known compared to oxidative soil.
The elemental affinity between Fe(II) minerals and anoxic soil is important information for understanding the complex and unclear elemental dynamics in the anoxic environment. Morse and Luther III (Citation1999) and Keene et al. (Citation2010) reported the affinity of trace elements on iron sulfide minerals. Berner (Citation1981) deduced that siderite was formed in low sulfide conditions where Fe(II) was not consumed by the formation of pyrite. Siderite is not always found even in anoxic soil in Japan; thus, reports related to siderite are very few.
In divalent iron minerals, we focused on siderite formed in actual soil because we found soil horizons with significant amounts of siderite and the siderite may affect the elemental dynamics in this soil. The first objective of the present study was to evaluate the elemental affinity for siderite found in actual soil. The second objective was to discuss the forming environment for siderite using the physicochemical properties of the soil. Additionally, we examined the rare earth element (REE) distribution pattern, used as a tracer or probe that reflects the origin or reaction process of the REEs in the target material (Matsui and Masuda Citation1963). This distribution pattern is generally expressed by plotting the logarithms of the REE concentration ratio between the target and referential material (Leedey chondrite) againt the atomic number of REEs.
2. Materials and methods
2.1. Site description
The paddy field in which we found siderite was alluvial lowland in the Tohoku region, Japan. The site is located near a tributary of the Kitakami and about 60 km upstream from the mouth of the river. The local altitude was 33 m, the annual mean air temperature was 11.4°C (from 2008 to 2012), the highest monthly mean temperature was 24.1°C (August), the lowest monthly mean temperature was −0.7°C (January), the annual mean precipitation was 1151 mm (from 2008 to 2012), the highest monthly average precipitation was 142 mm (September), and the lowest monthly average precipitation was 35 mm (January). The zinc, Cd, As and Pb concentrations of the soil are relatively high due to a non-ferrous metal mine located upstream. The main clay mineral of the soil is smectite. The soil was classified as Gleyic–Stagnic Fluvisol according to the World Reference Base for Soil Resources (WRB) (FAO Citation2006a).
2.2. Soil and siderite samples
We conducted a soil survey in both sides of the paddy field in April 2013. Soybean (Glycine max (L) Merr.) was cultivated in the field in 2012 due to a policy of reducing the production of rice (Oryza sativa L.) although rice production had lasted for a long time in 2011 or before. The sites were about 40 m apart. Soil profiles were described according to the guideline for soil description (FAO Citation2006b). One hundred milliliter core samples and soil samples after removal of both siderite nodules and aggregates of the siderite powder were collected from each horizon. The collected soil samples were treated as fresh soil (passed through a 4-mm sieve) and fine earth (air-dried and passed through a 2-mm sieve). The siderite nodules collected from the soil profiles were washed with distilled water and air-dried.
2.3. Soil and siderite analyses
A three-phase distribution and saturated hydraulic conductivity of the 100-mL soil core samples were analyzed using a three-phase meter (DIK-M100; Daiki, Saitama, Japan) and falling-head permeameter (DIK-4050; Daiki, Saitama, Japan), respectively. The soil pH values in a suspension of fresh soil/distilled water or 1 mol L−1 potassium chloride (KCl) in the ratio of 1:2.5 were measured by the glass electrode method. The particle size distribution was determined using the pipette method (International Society of Soil Science Citation1929). The total carbon (Total C) and nitrogen (Total N) contents were determined with an NC analyzer (Sumigraph NC-80; Sumika Chemical Analysis Service, Tokyo, Japan). The cation exchange capacity (CEC) was determined using the modified ammonium acetate (1 mol L−1 and pH7.0) compulsory displacement method (Schollenberger and Simon Citation1945). The dithionite citrate-extractable Fe (Fed) and ammonium oxalate-extractable Fe (Feo) were determined as described by Mehra and Jackson (Citation1960) and Blakemore et al. (Citation1987), respectively. The exchangeable cations in the percolated ammonium acetate, obtained from the CEC determination, and the concentrations of Fe in the Fed and Feo extracts were analyzed by inductively coupled plasma optical emission spectrometry (ICP–OES) (iCAP6300; Thermo Scientific, MA, USA). The base saturation (BS) in the soil was calculated by dividing the sum of the exchangeable Ca, K, Mg and Na by CEC and multiplying the results by 100%. These measured physicochemical properties were the average of two soil profiles.
The micromorphology and chemical composition of the siderite were observed with a scanning electron microscope coupled with energy dispersive X-ray spectrometry (SEM–EDX) (SU-8000; Hitach, Tokyo, Japan). The mineralogical composition of the powdered siderite was determined with an X-ray diffractometer (Miniflex; Rigaku, Tokyo, Japan, Cupper Kα radiation (CuKα), 30kV, 15mA).
2.4. Elemental analyses
The soil samples were ground to a fine powder, while the samples of siderite nodules were divided by size (< 1 cm, 1–2 cm and > 2 cm); then 10 siderites for each size were separately powdered. The siderite hardly dissolves in water because the logarithm value of the solubility product of siderite is −10.68 (Smith and Martell Citation1976). It is necessary for the elemental analysis to dissolve the siderite using a dilute acid. The effervescence due to carbon dioxide gas when adding 2.88 mol L−1 hydrochloric acid (10% HCl) is indicative of the presence of secondary carbonate including siderite in the soil survey (FAO Citation2006b). In this study, we measured the element concentration of the soil and siderite dissolved in 10% HCl and that of soil digested using aqua regia (1/3 HNO3–HCl, v/v) to obtain the aqua regia-digestible element concentration (Chen and Ma Citation2001). Residual elements that are not released by aqua regia digestion are mostly bound to silicate minerals and are considered unimportant for estimating the mobility and behavior of elements (Niskavaara et al. Citation1997).
The fine-ground soils and powdered siderites were dissolved in 10% HCl at the ratio of 1:100. The mixture, which was stored for 1 d and centrifuged, was used for the element analysis, and the residues of the soil and siderite after the 10% HCl dissolution were washed and dried, and their weights measured. The dissolution rates of the soil and siderite in the 10% HCl were obtained by subtracting the weight of the residue after dissolution from the initial weight. For analysis of the aqua regia-digestible element concentration of soil, a fine-ground soil sample (100 mg) was placed in a sealed melting pot for pressure thermal decomposition (PTFE melting pot; FLON Industry, Tokyo), and 5 mL of aqua regia was added and heated at 150°C for 6 h. The mixture was diluted to 50 mL with ultrapure water, and filtered.
The solution after dissolution using 10% HCl and aqua regia was diluted with 0.16 mol L−1 (1%) nitric acid to a suitable level for the element analysis. The Li, Cr, Co, Cu, Zn, Sr, Y, Mo, Cd, Sn, Sb, Ba, La, Ce, Pr, Nd, Sm, Eu, Gd, Tb, Dy, Ho, Er, Tm, Yb, Lu and Pb concentrations of the solution were analyzed by inductively coupled plasma–mass spectrometry (ICP–MS) (ICPM8500; Shimadzu, Kyoto, Japan). Na, Mg, Al, Si, P, S, K, Ca, Sc, Ti, Mn, Fe and As were analyzed by ICP–OES. For the As analysis, a hydride generation accessory (HYD–10; Thermo Scientific, MA, USA) was used to introduce the solution to the ICP–OES. The element concentrations of the solution were transformed to concentrations per dry weight of the soil or siderite by multiplying the solid:liquid ratio of the soil or siderite to acid and the moisture factor. The element concentrations of the soil and siderite for each size were the average of two soil profiles.
2.5. Data calculation and analyses
When the elemental affinity between soil and siderite is discussed, it is desirable to remove the influence of the unit by nondimensionalization. When analyzing the elemental distribution between the soil and siderite, a simple distribution coefficient (Csiderite/Csoil) may be used, in which the Csiderite and Csoil are the concentrations of the target element of the siderite and soil, respectively. However, the analysis of the elemental affinity using this causes a relative dilution and enrichment of the elements since the characteristic values including density, dissolution rate to acid, content of organic matter and water differ between the soil and siderite. White (Citation1995) analyzed the element dynamics during the soil forming process using a reference element as a standard for the elemental attenuation. Titanium (Ti) and zirconium are relatively immobile in soil and are therefore used as reference elements (Stiles et al. Citation2003). We applied the method of White (Citation1995) to the elemental affinity analysis between the soil and siderite using Ti as the reference element, as will be discussed later. Using the ratio of the Ti concentration of the siderite to the soil (Tisiderite/Tisoil) in addition to the dimensionless coefficient, Ro, (Csiderite/Csoil) is represented by the following equation:
The element concentrations of the soil were the average of the Cr1 and Cr2 horizons in which the siderite nodules were found. When the R0 value is calculated from Equation (1), the above characteristic values (e.g., density, and content of organic matter and water) of each soil and siderite sample, which should be multiplied or divided by the element concentrations, are offset. We compared the dimensionless R0 value of each element as an indicator of the elemental affinity between the soil and siderite. A one-way analysis of variance (ANOVA) was used to compare the differences in R0 values among the siderite sizes with R (R Core Team 2013, ver. 2.15.3).
3. Results
3.1. Soil and siderite characteristics
lists a brief description and the characterization data of the soil. We collected soil samples from the areas of the paddy field where soybean had not been planted. Nevertheless, the soil properties might be close to those of other parts of the paddy field because the areas had been used as paddy field for a long time. The soil profile was divided into five horizons to the depth of 60 cm. A weak development of the soil structure was recognized in the Ag and Bg horizons, but not in the other horizons. The gas phase rate and saturated hydraulic conductivity had increased in the upper horizons. These results suggest that the Ag and Bg horizons are susceptible to oxidation.
Table 1. Morphological and physico-chemical properties of soil.
The soil pH(H2O) values were 5.2 in the Ag and Bg horizons, and from 5.6 to 5.9 in the Cr1 to Cr3 horizons, and decreased in the upper horizons, though the pH (KCl) values in all the horizons ranged from 3.7 to 4.1 and showed little change. The total C of the Ag horizon was the highest, while the CEC (25.2–29.5 cmolc kg−1) and BS (52.4–59.8%) did not change by a large extent in the soil profile. The Fed values were slightly higher than the Feo in the Ag to Cr2 horizons while they were lower than that in Cr3 horizon, indicating that poorly soluble Fe(II) salts are possibly formed in the Cr3 horizon.
The effervescence of carbon dioxide gas was recognized when the 10% HCl was added to the siderite nodules collected from the soil profile. The micromorphology result using SEM in shows that the collected nodules were aggregations of fine columnar or blocky particles enveloped by platy clays, and grew by taking clay around them in. Additionally, the EDX spectrum indicated that nodules consisted of a majority of Fe and small amounts of P, Ca and Mn ( and ). The XRD chart indicated that the components of the powdered nodules were mainly siderite and a small amount of quartz (). These results provide evidence that the main components of the collected nodules are undoubtedly siderite.
Figure 1. (a) Scanning electron microscope image of siderite. (b) Energy-dispersive X-ray (EDX) mapping image of iron in siderite. (c) EDX spectrum corresponding to point 1 in a; O: oxygen, Al: aluminum, Si: silicon, P: phosphorus, Ca: calcium, Mn: manganese, Fe: iron. (d) d1: X-ray diffraction (XRD) chart of powdered siderite sample; a rising baseline due to an iron-rich sample was corrected to be nearly flat to compare the peak positions with reported data. d2: XRD chart of powdered siderite reported by Brindley and Brown (Citation1980), Q: quartz.
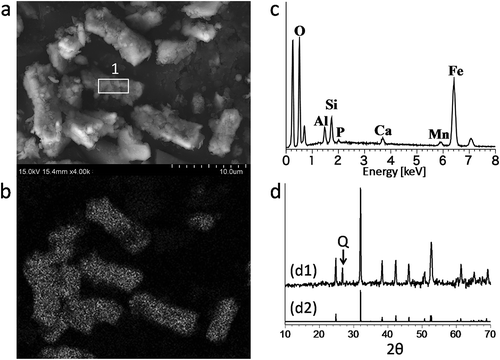
3.2. Element concentrations of soils and siderites
The aqua regia-digestible and 10% HCl soluble element concentrations of the soil are shown in . The order of the aqua regia-digestible element concentrations was Al > Fe > Ca > Mg > K > Ti > Na > Si > P > S, whereas that of the 10% HCl solubles was Fe > Al > Ca > S > Mg > Si > Zn > Na > K > P. As compared to the 10% HCl element concentration, the order of the aqua regia-digestible element concentrations of Al and Ti increased. The ratio of the 10% HCl soluble to aqua regia-digestible element concentration was less than 100% for every measured element; specifically, Ti, Cr, K, Na and Al ratios were less than 10% (). The dissolution trend of aqua regia differed from that of 10% HCl. Besides, the dissolution rates of the soil in 10% HCl in this study were 11.3–16.6% (). Chen and Ma (Citation2001) indicated that the soil digestion using microwaved aqua regia achieved 80% of the elemental recoveries on average for six major and 10 minor elements. Aqua regia, although it could not completely decompose the soils, dissolves the soils more than 10% HCl. By contrast, high Si recovery is due to precipitation of silica under highly acidic conditions in aqua regia.
Table 2. Aqua regia digestible and 2.88 mol L−1 hydrochloric acid (10% HCl) soluble element concentrations of soil, ratio of 10% HCl soluble concentration to aqua regia digestible concentration (HCl /Aqua regia), dissolution rate of soil in 10% HCl.
The dissolution rates of the siderite nodule in 10% HCl were 79.9–80.1% (), showing that the fraction of siderite dissolved in the 10% HCl was much greater than that of soil (11.3–16.6%; ). If the siderite nodules dissolved in 10% HCl were pure, Fe concentrations considering the dissolution rate resulted in the range from 385,000 to 386,000 mg kg−1 and were a little higher than those in (313,000–334,000 mg kg−1). Iron concentrations in response to the siderite sizes showed the degree of purity of the siderite nodule. Iron concentrations in decrease as the siderite size increases, suggesting that impurities like clay minerals or organic substance increase as the siderite size increases. Considering the results, the 10% HCl dissolves the fraction related to siderite more selectively than aqua regia does. Since a soil dissolution method suitable for investigating the elemental affinity only related to siderite has not yet been developed, we applied the element concentrations of the soil (Csoil) and siderite (Csiderite) dissolved in the 10% HCl for comparing the elemental affinity for siderite.
Table 3. Element concentrations and dissolution rate corresponding to siderite size dissolved in 2.88 mol L−1 hydrochloric acid (10% HCl), and element concentration ratio of siderite to soil.
Incidentally, the aqua regia-digestible and 10% HCl soluble concentrations of Ti in the soil profile were 3380–3590 and 62–72 mg kg−1, respectively (). These small variations in the concentration show that Ti seldom fluctuates in these soil profiles. Based on the data from and , the ratios of the 10% HCl soluble Ti concentration of the siderite nodule to that of soil (average of Cr1 and Cr2 horizons) were 0.78–0.82. The results indicate that Ti tends to be slightly excluded from the siderite nodule. We adapted Ti as the reference element for calculating the R0 value after White (Citation1995).
3.3. R0 values of elements
shows the R0 values calculated from Equation (1). If the R0 value is unity, the affinity of the target element between the soil and the siderite is equal to Ti; if the R0 value is zero, the target element is not transferred from the soil to the siderite nodule. The effect of siderite particle size on the R0 value of all elements was not significant (p values > 0.05, one-way ANOVA). The elements with an accumulation tendency (R0 > 1) were in the order Mn > Fe > P > Ca > Co > Cr > Mo > Y > Sc > Si > Sr > Ba, while those with the tendency from equal to exclusion (R0 ≦ 1) were in the order Sn > As > K > Al > Zn > S > Na > Mg > Li > Cu > Sb > Pb > Cd.
Figure 2. R0 values of elements corresponding to siderite size (< 1 cm, 1–2 cm and > 2 cm). Mn, Fe, P, Ca, Co, Cr, Mo, Y, Si, Sc, Sr, Ba, Sn, As, K, Al, Zn, S, Na, Mg, Li, Cu, Sb, Pb, and Cd are manganese, iron, phosphorus, calcium, cobalt, chromium, molybdenum, yttrium, silicon, scandium, strontium, barium, tin, arsenic, potassium, aluminum, zinc, sulfur, sodium, magnesium, lithium, copper, antimony, lead, and cadmium, respectively. R0 value is nondimensional parameter calculated by (Csiderite/Csoil) = R0(Tisiderite/Tisoil). Csoil and Csiderite are element concentrations of soil and siderite, and Tisoil and Tisiderite are Ti concentrations of soil and siderite, respectively. Element concentrations of soil were the average of Cr2 and Cr3 horizons. R0 values are arranged from highest to lowest. Vertical bar represents standard deviations of the means (n = 2). P values of the effect of siderite particle size on the R0 value of all elements were > 0.05 (one-way analysis of variance, ANOVA).
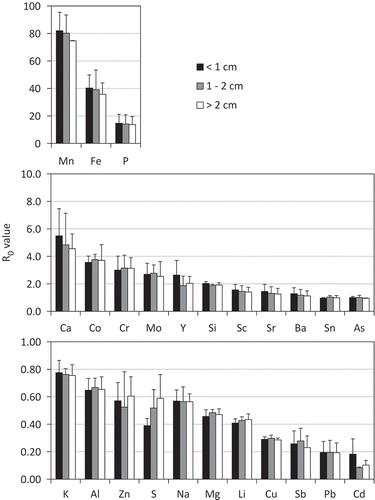
4. Discussion
4.1. Relationship between siderite formation and physicochemical properties of soil
Kyuma (Citation2004) evaluated the stable chemical form of iron from the pH–Eh diagram, taking into account the physicochemical data. When the Fe and carbon dioxide concentration were assumed to be 10–3 mol L−1, which might be realistic for the natural paddy soil, the range of the soil pH value in which siderite can be formed in the pH is between 6.0 and 10.4. In the Cr1 to Cr3 horizons where siderite nodules and the aggregation of powder were found, the soil pH(H2O) values were 5.6–5.9 () and slightly outside the postulated pH–Eh region of siderite. The soil pH values before the soil sampling were supposed to be higher and in a stable range of siderite formation because of the partial oxidation of Fe(II) ion after the soil sampling. Precise field monitoring is needed to specify the soil environment for siderite formation.
Iwasa (Citation1959) indicated that siderite was found in the gley horizon containing organic material with a soil texture ranging from loam to clay loam, but not in gley horizon with a bright color. In the Cr1 to Cr3 horizons, the total C values were more than 13 g kg−1 () and organic material was included to some extent. The soil textures ranged from clay loam to light clay, and the soil colors were not bright (); these conditions were mostly in agreement with those proposed by Iwasa (Citation1959). Such findings for siderite formation will give rise to valuable information about a carbon sink under conditions where the soil is not alkaline, as well as about the dynamics of Fe(II) in anoxic conditions.
4.2. Elemental affinity for siderite
In general, the same group elements in the periodic table have a similar chemistry. Alkali metallic elements including Li, Na and K were removed from the siderite (). However, for the group 2 elements which form carbonates with low solubilities, Ca, Sr and Ba were accumulated, while Mg was excluded from the siderite. Romanek et al. (Citation2009) formed a solid solution of calcite (CaCO3)–siderite from a solution including Fe, Ca and Mg to synthesize carbonate, and showed that Mg was removed from the crystalline structure because the presence of aqueous Ca did not facilitate the incorporation of Mg in the solid. This supports the results in this study indicating the removal tendency of Mg.
Postma (Citation1982) has shown that vivianite was detected in siderite found in sediment. The results that the R0 value of P was higher than 10 () and a small amount of P was detected in the EDX spectra () support the fact that vivianite was formed with the siderite. The R0 values can be affected by minor inclusion in the siderite.
Several studies have reported that heavy metals including Cr, Co, Cu, Zn, Mo, Cd and Pb have an affinity for iron (III) hydroxide (McKenze Citation1980; Backes et al. Citation1995; Dixit and Hering Citation2003). In this study, Cr, Co and Mo, whose R0 values were high, in the range from 2 to 4 (), accumulated in the siderite nodule and had the same tendency toward iron hydroxide. In contrast, chalcophile elements like Zn, Cu, As, Cd, Sn, Sb and Pb, whose R0 values were low, in the range from 0.1 to 1.1, showed the opposite tendency toward iron hydroxide. Kirk (Citation2004) has shown the possibility that chalcophile elements precipitate as insoluble sulfides produced by the reduction of sulfate. The solubility of sulfides of the chalcophile elements like as Zn, Cu, Cd, Sn, Sb and Pb is lower than that of Fe (Smith and Martell Citation1976). The low R0 values of the chalcophile elements imply that the insoluble sulfide was formed in the anoxic soil before these elements were incorporated into the siderite nodules. However, it is possible that the R0 values of Zn, As, Cd and Pb were underestimated due to their high concentrations of the soil affected by the metal mine located upstream.
4.3. Estimation of environment during siderite formation
The elemental affinity for the siderite differed from that for iron hydroxide as discussed above. This suggests the possibility that the elemental affinity for minerals reflects the environment, including redox during mineral formation. Therefore, we attempted to speculate regarding the environment during the siderite formation, using the R0 values.
Mozley (Citation1989) indicated that siderite collected from fluvial sediment was relatively pure and contained much Mn (more than 2% as MnCO3), while that collected from marine sediment was impure because of highly substituted Mg for Fe in the siderite lattice (up to 41% mol). The siderite we collected had high purity (81 to 86%) and contained much Mn (1.8 to 1.9% equivalent to 3.8 to 4.0% MnCO3) in addition to very low Mg concentration compared with Fe (), which implies that siderite collected in this study was formed in freshwater conditions. This is supported by the fact that the paddy field where we collected the siderite nodule is located near a river and about 60 km upstream from the mouth of the river, and the sampled site was hardly affected by changes in sea water level.
4.4. Distribution of REEs between the soil and the siderite
Although the REEs have similar chemical properties, the R0 value of Sc (1.4) differed from that of Y (2.5) (). This difference may reflect the difference in chemical properties of the REEs between the soil and the siderite. If the chemical properties for REEs (e.g., chemical bonding and coordination number) are similar between the soil and the siderite, the REE distribution ratio would also be similar for all the elements. But if not, the ratio is expected to vary with the atomic number of elements (Ohta and Kawabe Citation2000).
shows that the REEs having lower atomic numbers were incorporated into the soil, and log(Csiderite/Csoil) roughly increased as the atomic number increase. More interestingly, it has the tendency not to increase linearly but to increase with forming a concave curve at the intervals of the four elements with the increase of atomic number. These phenomena, although there are several kinds of shapes of the curve, were generically called ‘tetrad effects’, and found in the partition coefficient of REE complex forming (Peppard et al. Citation1969) and the REE abundance in geochemical sample (Kawabe et al. Citation1991).
Figure 3. Rare earth element (REE) distribution pattern of siderite–soil system.
CSiderite: 2.88 mol L−1 hydrochloric acid (HCl) soluble element concentration of siderite, Csoil: 2.88 mol L−1 HCl soluble element concentration of soil. La, Ce, Pr, Nd, Pm, Sm, Eu, Gd, Tb, Dy, Ho, Er, Tm, Tb, and Lu are lanthanum, cerium, praseodymium, neodymium, promethium, samarium, europium, gadolinium, terbium, dysprosium, holmium, erbium, thulium, ytterbium, and lutetium, respectively. Promethium of atomic number 61 does not exist in nature because Pm does not have a stable isotope. The curve between Tm and Tb was passed through their intermediate point. Vertical bar represents ranges of values (n = 2).
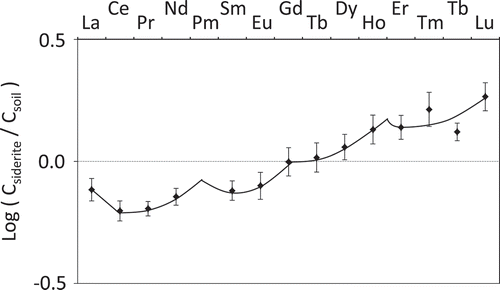
5. Conclusion
The concentration ratio of the target element of siderite to the soil was expressed by the product of the dimensionless R0 value and ratio of Ti concentration of siderite to the soil; then the affinity of each element for the siderite was analyzed by comparing the R0 value. Based on a comparison with the elemental affinity for iron oxyhydroxide previously reported, the affinity of Cr, Co and Mo by the siderite had the same accumulation tendency, while chalcophile elements like Cu, Zn, Cd and Pb had the opposite, exclusionary tendency. The environment during the siderite formation deduced from the elemental affinity indicated that the siderite we collected was probably formed in freshwater and anoxic conditions. The REE distribution pattern showed the tetrad effects to increase with forming a concave curve at the intervals of four elements with the increase of atomic weight.
References
- Backes CA, McLaren RG, Rate AW, Swift RS 1995: Kinetics of cadmium and cobalt desorption from iron and manganese oxides. Soil Sci. Soc. Am. J., 59, 778–785. doi:10.2136/sssaj1995.03615995005900030021x
- Berner R 1981: A new geochemical classification of sedimentary environments. J. Sediment. Petrol., 51, 359–365.
- Blakemore LC, Searle PL, Darly BK 1987: Extractable iron, aluminum and silicon. In Methods for Chemical Analysis of Soils, New Zealand Soil Bureau Scientific Report 10A, Ed. Blakemore L, pp. 71–76. DISR, Lower Hutt.
- Borch T, Kretzschmar R, Kappler A, Cappellen PV, Ginder–Vogel M, Voegelin A, Campbell K 2010: Biogeochemical redox processes and their impact on contaminant dynamics. Environ. Sci. Technol., 44, 15–23. doi:10.1021/es9026248
- Breemen NV, Buurman P 2002: Soil chemical processes. In Soil Formation. Eds NV Breemen and P Buurman, pp. 41–82. Kluwer Academic Publishers, Dordrecht.
- Brindley GW, Brown G 1980: Carbonates. In Crystal Structures of Clay Minerals and Their X–Ray Identification. Eds GW Brindley and G Brown, pp. 389–390. Mineralogical Society, London.
- Bunzl K, Schmidt W, Sansoni B 1976: Kinetics of iron ex-change in soil organic matter, IV. Adsorption and de-sorption of Pb2+, Cu2+, Cd2+, Zn2+, and Ca2+ by peat. J. Soil Sci., 27, 32–41. doi:10.1111/j.1365-2389.1976.tb01972.x
- Chen M, Ma LQ 2001: Comparison of three aqua regia digestion methods for twenty florida soils. Soil Sci. Soc. Am. J., 65, 491–499. doi:10.2136/sssaj2001.652491x
- Dixit S, Hering JG 2003: Comparison of arsenic(V) and arsenic(III) sorption onto iron oxide minerals: implications for arsenic mobility. Environ. Sci. Technol., 37, 4182–4189. doi:10.1021/es030309t
- Doyle MO, Otte ML 1997: Organism–induced accumulation of iron, zinc and arsenic in wetland soils. Environ. Pollut., 96, 1–11. doi:10.1016/S0269-7491(97)00014-6
- FAO 2006a: World Reference Base for Soil Resources 2006, pp. 11–100. FAO, Rome.
- FAO 2006b: Guideline for Soil Description, fourth edition. pp. 1–97. FAO, Rome.
- International Society of Soil Science 1929: Minutes of the first commission meetings. In International Congress of Soil Science, pp. 215–220. International Society of Soil Science, Washington, D. C.
- Iwasa Y 1959: On the siderite concretion in paddy soils. Pedologist, 3, 53–58. (in Japanese).
- Kawabe I, Kitahara Y, Naito K 1991: Non-chondritic yttrium/holmium ratio and lanthanide tetrad effect observed in pre-Cenozoic limestones. Geochem. J., 25, 31–44. doi:10.2343/geochemj.25.31
- Keene AF, Johnston SG, Bush RT, Burton ED, Sullivan LA 2010: Reactive trace element enrichment in a highly modified, tidally inundated aced sulfate soil wetland: east Trinity, Australia. Mar. Pollut. Bull., 60, 620–626. doi:10.1016/j.marpolbul.2010.02.006
- King HB, Torrance JK, Bowen LH, Wang C 1990: Iron concretions in a typic Dystrochrept in Taiwan. Soil Sci. Soc. Am. J., 54, 462–468. doi:10.2136/sssaj1990.03615995005400020029x
- Kirk G 2004: The Biogeochemistry of Submerged Soils, pp. 1–258. John Wiley & Sons, Chichester.
- Kyuma K 2004: Fundamental chemical reactions in sub-merged paddy soils. In Paddy Soil Science. Ed K Kyuma, pp. 60–81. Kyoto University Press, Kyoto.
- Matsui Y, Masuda A 1963: On the variation in relative abundances of rare earth elements among meteorites, igneous rocks and sediments. Geochim. Cosmochim. Acta, 27, 547–549. doi:10.1016/0016-7037(63)90087-5
- McKenze RM 1980: The adsorption of lead and other heavy metals on oxides of manganese and iron. Aust. J. Soil Res., 18, 61–73. doi:10.1071/SR9800061
- Mehra OP, Jackson ML 1960: Iron oxide removal from soils and clays by a dithionite–citrate system buffered with sodium bicarbonate. Clays Clay Miner., 7, 317–327. doi:10.1346/CCMN.1958.0070122
- Morse JW, Luther III GW 1999: Chemical influences on trace metal–sulfide interactions in anoxic sediments. Geochim. Cosmochim. Acta, 63, 3373–3378. doi:10.1016/S0016-7037(99)00258-6
- Mozley PS 1989: Relation between depositional environment and the elemental composition of early diagenetic siderite. Geology, 17, 704–706. doi:10.1130/0091-7613(1989)017<0704:RBDEAT>2.3.CO;2
- Murano H, Takata Y, Isoi T 2015: Origin of the soil texture classification system used in Japan. Soil Sci. Plant Nutr., 61, 688–697. doi:10.1080/00380768.2014.998594
- Neubauer SC, Emerson D, Megonigal JP 2007: Microbial oxidation and reduction of iron in the root zone and influences on metal mobility. In Biophysico–Chemical Processes of Heavy Metals and Metalloids in Soil Environments, Eds. Violante A, Huang PM, Gadd GM, pp. 339–372. John Wiley & Sons, Hoboken.
- Niskavaara H, Reimann C, Chekushin V, Kashulina G 1997: Seasonal variability of total and easily leachable element contents in topsoils (0-5 cm) from eight catchments in the European arctic (Finland, Norway, and Russia). Environ. Pollut., 96, 261–274. doi:10.1016/S0269-7491(97)00031-6
- Ohta A, Kawabe I 2000: Theoretical study of tetrad effects observed in REE distribution coefficients between marine Fe–Mn deposit and deep seawater, and in REE(III) –carbonate complexation constants. Geochem. J., 34, 455–473. doi:10.2343/geochemj.34.455
- Pandey AK, Pandey SD, Misra V 2000: Stability constants of metal–humic acid complexes and its role in environ-mental detoxification. Ecotoxicol. Environ. Safety, 47, 195–200. doi:10.1006/eesa.2000.1947
- Patrick WH, Reddy CN 1978: Chemical changes in rice soils. In Soils and Rice, Ed. IRRI, pp. 361–379. IRRI, Los Banos, Philippines.
- Peppard DF, Mson GW, Lewey S 1969: A tetrad effect in the liquid-liquid extraction ordering of lanthanides (III). J. Inorg. Nucl. Chem., 31, 2271–2272. doi:10.1016/0022-1902(69)90044-X
- Postma D 1982: Pyrite and siderite formation in brackish and freshwater swamp sediments. Am. J. Sci., 282, 1151–1183. doi:10.2475/ajs.282.8.1151
- R Core Team 2002: A language and environment for statistical computing. R Foundation for Statistical Computing. Vienna.
- Romanek CS, Jimenez-Lopez C, Navorro AR, Sanchez-Roman M, Sahai N, Coleman M 2009: Inorganic synthesis of Fe-Ca-Mg carbonates at low temperature. Geochim. Cosmochim. Acta, 73, 5361–5376. doi:10.1016/j.gca.2009.05.065
- Schollenberger CJ, Simon RH 1945: Determination of exchange capacity and exchangeable bases in soil-ammonium acetate method. Soil Sci., 59, 13–24. doi:10.1097/00010694-194501000-00004
- Smith RM, Martell AE 1976: Inorganic ligands. In Critical Stability Constants. Eds RM Smith and AE Martell., Volume 4: inorganic Complexes, pp. 1–130. Plenum Press, New York.
- Sparks DL 1999: Kinetics of reactions in pure and mixed systems. In Soil Physical Chemistry, Eds. Sparks DL, pp. 83–178. CRC Press, Boca Raton.
- Stiles CA, Mora CI, Driese SG 2003: Pedogenic processes and domain boundaries in a vertisol climosequence: evidence from titanium and zirconium distribution and morphology. Geoderma, 116, 279–299. doi:10.1016/S0016-7061(03)00105-8
- White AF 1995: Chapter 9 Chemical weathering rates of silicate minerals in soils. In Chemical Weathering Rates of Silicate Minerals. Reviews in Mineralogy Volume 31, Eds. White AF, Brantley SL, pp. 407–462. Mineralogical Society of America, Washington, D.C.
- YoungDo N, Park HI 2010: A study on adsorption of Pb, Cu, Zn and Cd onto natural clay. Int. J. Environ. Res., 5, 413–424.
- Zhang C, Ge Y, Yao H, Chen X, Hu M 2012: Iron oxidation-reduction and its impacts on cadmium bioavailability in paddy soils: a review. Front. Environ. Sci. Eng., 6, 509–517. doi:10.1007/s11783-012-0394-y