ABSTRACT
Root colonization by arbuscular mycorrhizal (AM) fungi has traditionally been analyzed by microscopy. However, this method is time consuming and it is often difficult to distinguish between AM and non-AM fungi. In this study, we analyzed the fatty acid profiles in soybean roots colonized by AM fungi to determine if specific fatty acids derived from AM fungi can be used as markers for the intensity of the AM fungal colonization. The wild-type Enrei and hypernodulating Kanto100 soybean cultivars were inoculated with an AM fungus (Gigaspora rosea) alone or with Bradyrhizobium diazoefficiens, which nodulates soybean roots. Fatty acids 20:1ω9, 20:4ω6, and 20:5ω3 were specifically detected in the lateral roots of AM fungus-inoculated and dual-inoculated soybean plants. In the second lateral roots, the percentage of AM-specific fatty acids (i.e., 20:1ω9, 20:4ω6, and 20:5ω3) derived from AM fungi was closely correlated with the intensity of the AM fungal colonization. We propose that the AM-specific fatty acids represent useful markers for estimating the degree of AM fungal colonization. The percentage of AM-specific fatty acids was more than twofold higher in the second lateral roots than in the first lateral roots. Thus, the degree of AM fungal colonization is probably twofold higher in the second lateral roots than in the first lateral roots.
1. Introduction
Arbuscular mycorrhizal (AM) fungi in the subphylum Glomeromycotina form mutualistic associations with more than 80% of the examined vascular plant species, including many crop plants. The hyphae of AM fungi colonize the root cortex and form highly branched, bush-like structures known as arbuscules within host cells. Colonization of plant roots by AM fungi significantly improves plant mineral nutrition, increases plant growth, and enhances tolerance against pathogens, drought, and other environmental stresses (Augé Citation2001; Porcel et al. Citation2003; Smith and Read Citation2008). Root colonization by AM fungi has traditionally been determined based on a microscopic analysis of root tissue stained with trypan blue (Rajapakse and Miller Citation1994). However, this method is time consuming and it is often difficult to distinguish between AM and non-AM fungi. Moreover, observing the mycorrhization of thick roots with a large diameter, such as the first lateral root of soybean, is often problematic, because trypan blue method often produces high background staining of cortical cells or parts of the stele. The use of biochemical markers for estimating root colonization by AM fungi may overcome some of these drawbacks.
Two important types of lipids found in plants and AM fungi are phospholipids (membrane constituents) and neutral lipids (energy storage in eukaryotes). These lipid types contain fatty acids connected to a glycerol backbone. Fatty acids derived from the phospholipids (i.e., PLFAs, which are mainly localized in hyphal cells) and neutral lipids (which are mainly localized in spores and vesicles) of AM fungi are potentially useful for detecting or identifying AM fungi (Graham et al. Citation1995; Bentivenga and Morton Citation1996; Jansa et al. Citation1999; Olsson Citation1999). Some fatty acids are specific markers for AM fungi. In the case of Glomus species, fatty acids 16:1ω5, 18:1ω9, 20:3, 20:4, and 20:5 have been detected in AM fungal spores and the roots of plants colonized by AM fungi (Beilby and Kidby Citation1980; Jabaji-Hare Citation1988; Pacovsky and Fuller Citation1988; Pacovsky Citation1989; Graham et al. Citation1995; Jansa et al. Citation1999; Madan et al. Citation2002). Additionally, Olsson et al. (Citation1995, Citation1997, Citation1998) estimated the biomass of the external hyphae of several Glomus species using the PLFA 16:1ω5. Furthermore, fatty acids 18:1ω9 and 20:1ω9 have been identified as specific markers in the spores of some Gigaspora species (Bentivenga and Morton Citation1994; Bentivenga and Morton Citation1996; Graham et al. Citation1995; Madan et al. Citation2002). Graham et al. (Citation1995) reported that sudan grass roots colonized by Gigaspora rosea contain fatty acid 20:1ω9, but nonmycorrhizal roots do not. Moreover, Sakamoto et al. (Citation2004) suggested that PLFA 20:1ω9 may be a useful marker for quantifying the external hyphae of G. rosea.
In this study, we analyzed the fatty acids in soybean roots colonized by G. rosea to test whether specific fatty acids derived from AM fungi are useful markers for determining the degree of root colonization by AM fungi. Globally, soybean is one of the most important crops for production of protein, oil, and biofuel resources. More production of soybean is needed to meet the increasing demands of the world market. Soybean roots are well known to establish dual symbiosis with nodule-inducing rhizobia (bacteria in the Rhizobiaceae) and AM fungi to improve the nitrogen and phosphorus nutrition of the plant. During the dual symbiosis, each microbial symbiont has been shown to affect the activity of the other. The existing nodules systemically suppress subsequent nodulation in other parts of the roots. This feedback control has been named the autoregulation of nodulation (Caetano-Anollés and Gresshoff Citation1991). Reciprocal grafting experiments have revealed that some forms of autoregulation are systemically regulated by the shoot (Delves et al. Citation1986). Shrihari et al. (Citation2000) have reported that hypernodulating mutants of soybean, defective in the autoregulation, have higher intensity of AM fungal colonization than the respective wild types. Sakamoto and Nohara (Citation2009), using a reciprocal grafting technique, demonstrated that the shoot of soybean negatively controls AM fungal colonization as well as root nodulation. Moreover, Sakamoto et al. (Citation2013) indicated that root nodulation suppressed AM fungal colonization in the root of soybean and concluded that autoregulation of the host plant, initiated by nodulation, was involved in this phenomenon. Based on the previous results, the presence of autoregulation or root nodulation may affect the level of fatty acids in AM fungi within soybean roots. Therefore, we used hypernodulating soybean mutant cv. Kanto100, defective in the autoregulation, along with wild-type soybean cv. Enrei, and designed the experiment using not only plants inoculated with AM fungus alone but also plants together with rhizobium in this study. Additionally, we compared the root colonization by AM fungus in the first lateral roots with that in the second lateral roots based on the AM-specific fatty acids. As we stated above, it is often difficult to observe the mycorrhization of thick roots such as the first lateral root using trypan blue method, since the background by trypan blue staining is quite high. We analyzed AM-specific fatty acids in the first lateral root to solve this problem in this study.
2. Materials and methods
2.1. Biological materials
We used two soybean (Glycine max (L.) Merr.) cultivars, namely wild-type Enrei and its hypernodulating mutant, Kanto100. Kanto100 was selected from a cross between Enrei and En6500 (Takahashi et al. Citation2005). En6500 was generated by ethyl methanesulfonate-induced mutagenesis of Enrei (Akao and Kouchi Citation1992). Kanto100 and En6500 exhibit a hypernodulating phenotype due to the presence of a stop codon (AAA to TAA) near the transmembrane domain-encoding sequence of the NTS1/GmNARK gene, which is associated with autoregulatory activities (Nishimura et al. Citation2002; Arai et al. Citation2005). However, in field trials, the soybean yield of Kanto100 was higher than that of En6500, and similar to that of Enrei. Kanto 100 and Enrei seeds were kindly provided by the NARO Institute of Crop Science, Tsukuba, Japan. Both cultivars were inoculated with G. rosea MAFF520062 (Ministry of Agriculture, Forestry and Fisheries of Japan) and Bradyrhizobium diazoefficiens USDA110 (USDA Rhizobium culture collection), which nodulates soybean roots. Gigaspora species are commonly found in soybean fields (Schenck and Kinloch Citation1980; Khalil et al. Citation1992; Isoi Citation1997).
2.2. Plant growth conditions
Enrei and Kanto100 seeds were immersed in 70% (v/v) ethanol for 12 min and washed once with sterile distilled water. The seeds were surface-sterilized with 10% (v/v) H2O2 for 3 min and washed five times with sterile distilled water. Soybean seeds were then germinated in an incubator at 25°C and then transferred to plastic pots (12 cm diameter, 15 cm height) filled with sterilized river sand and loam soil (3:2). Enrei and Kanto100 plants were divided into three groups, with one serving as a noninoculated control. The other two groups were inoculated with G. rosea alone or together with B. diazoefficiens. Pot cultures of G. rosea were obtained after mass multiplying on soybean plants grown in sterilized sand. The AM fungal inoculum (i.e., 200 spores) was applied to each pot. Meanwhile, B. diazoefficiens was grown in a liquid yeast-mannitol medium for 7 days at 30°C, after which a 1-mL aliquot of the rhizobial suspension (i.e., 1 × 108 cells mL−1) was applied under each soybean seed during sowing. The inoculated soybean plants were grown in a glasshouse under natural conditions (maximum day temperature: 26–31°C; minimum night temperature: 16–21°C; and day length: 14–16 h) and were watered regularly. Each pot was treated with the following chemicals: 285 mg NH4NO3, 95.5 mg K2HPO4, 49.6 mg K2SO4, 262 mg CaCl2·2H2O, 245 mg MgSO4·7H2O, 43.9 mg Fe-EDTA·3H2O, 1.43 mg MnSO4·5H2O, 0.25 mg ZnSO4·7H2O, 0.25 mg CuSO4·5H2O, 0.25 mg H3BO3, 0.06 mg Na2MoO4·2H2O, and 0.03 mg CoCl2·6H2O. Soybean plants were harvested at 6 weeks (flowering stage) and 9 weeks (formation of green pods) after sowing. The plants were carefully uprooted and the roots were washed with tap water. The lateral roots were collected and divided into the first and second lateral roots (including branched roots).
2.3. AM fungal colonization
Second lateral roots were cut into 1-cm segments, cleared, and then stained with trypan blue following the method described by Rajapakse and Miller (Citation1994). Ninety stained root samples were randomly selected and observed under a light microscope to determine the intensity of the AM fungal colonization (Trouvelot et al. Citation1986).
2.4. Measurement of fatty acids in the root
Root fatty acid profiles were analyzed using an automated MIDI system (MIDI Inc., Newark, DE, USA) as described by Sakamoto et al. (Citation2005). Lateral root samples were freeze-dried and ground to a fine powder. Approximately 75 mg powder was placed in a screw-capped glass tube with 1.5 mL 3.75 M NaOH prepared in methanol. The samples were mixed, heated in a 100°C water bath for 5 min, mixed again, and then heated for an additional 25 min. After cooling in running water, the samples were methylated with 3 mL 3.6 M HCl prepared in methanol, mixed, and placed in an 80°C water bath for 10 min. After cooling, the solutions were treated with 1.88 mL 1:1 hexane:methyl-tert butyl ether and then rotated for 10 min. The liquid organic phase was transferred to a clean tube and washed once with 4.5 mL 0.3 M NaOH. The extract was then evaporated under a stream of N2 in a fume hood. The residue was resuspended in 0.5 mL 1:1 hexane:methyl-tert butyl ether prior to being analyzed by gas chromatography. The fatty acid methyl esters (FAMEs) were separated using the 6890N gas chromatograph system (Agilent Technologies Inc., Palo Alto, CA, USA) with the automated procedure controlled by the MIDI program (Sherlock Microbial Identification System ver. 4.5). The gas chromatograph was equipped with a 25 m × 0.2 mm fused silica capillary column (Ultra2). The temperature was increased from 170 to 260°C at 5°C min−1. Helium was used as the carrier gas. The proportion of the total FAME content (%) represented by each identified FAME peak was calculated using the MIDI program.
2.5. Statistical analysis
The relationship between the specific fatty acids derived from AM fungi and the intensity of the AM fungal colonization was assessed by Pearson’s correlation coefficient test. Differences in the intensity of the AM fungal colonization and the percentage of the specific fatty acids derived from AM fungi in soybean lateral roots among treatments underwent a one-way analysis of variance. If significant differences were detected, multiple comparisons of means were completed in a Tukey–Kramer test. The percentage data were arcsine transformed and analyzed. The SPSS Statistics 24.0 program (IBM Co., Armonk, NY, USA) was used for all statistical analyses.
3. Results and discussion
We observed that 76.8% and 86.7% of the second lateral roots of Enrei plants inoculated with G. rosea alone were colonized by the AM fungus at 6 and 9 weeks after sowing (WAS), respectively (). These percentages were comparable to those of similarly inoculated Kanto100 plants (). In contrast, at 6 and 9 WAS, the intensity of the AM fungal colonization of Enrei roots inoculated with B. diazoefficiens and G. rosea was 29.0% and 20.7% lower, respectively, than the corresponding percentages for Kanto100 plants inoculated with the two symbionts. Additionally, the mycorrhizal intensities in Kanto100 plants were similar regardless of whether plants were inoculated with G. rosea alone or combined with B. diazoefficiens. However, at 6 and 9 WAS, the mycorrhizal intensities were 27.9% and 15.0% lower, respectively, in Enrei plants inoculated with the two symbionts compared with the plants treated with G. rosea alone. These results suggest that rhizobial nodulation suppresses AM fungal colonization, partly via the nodulation-induced autoregulation of the host plant (Sakamoto et al. Citation2013).
Table 1. The intensity of AM fungal colonization in the second lateral root of soybeans at 6 and 9 weeks after sowing (%, n = 5).
Twenty-seven fatty acid types were detected in the first lateral roots of soybean plants at 6 and 9 WAS (). The same fatty acids were detected in the second lateral roots (). In all treated soybean plants, the dominant fatty acids were 16:0, 18:2ω6,9/a18:0, 18:1ω9, 19:1ω8t, and 18:0 2OH, which are derived from plant cells. In the AM fungus-inoculated and dual-inoculated samples, fatty acids 20:1ω9, 20:4ω6, and 20:5ω3 were detected in both lateral roots. These observations imply that fatty acids 20:1ω9, 20:4ω6, and 20:5ω3 can be derived from soybean plants inoculated with G. rosea. Previous studies confirmed that fatty acid 20:1ω9 is present in the spores of Gigaspora species and in plant roots associated with these species (Bentivenga and Morton Citation1994; Bentivenga and Morton Citation1996; Graham et al. Citation1995; Madan et al. Citation2002; Sakamoto et al. Citation2004). Fatty acid 20:4 has also been detected in the spores of Gigaspora species (Bentivenga and Morton Citation1994; Bentivenga and Morton Citation1996; Madan et al. Citation2002).
Table 2. Fatty acid profiles in the first lateral root of soybeans at 6 and 9 weeks after sowing (%, n = 5).
Table 3. Fatty acid profiles in the second lateral root of soybeans at 6 and 9 weeks after sowing (%, n = 5).
In the second lateral roots of soybean, we examined the relationship between the percentage of AM-specific fatty acids (i.e., 20:1ω9, 20:4ω6, and 20:5ω3) derived from G. rosea and the intensity of AM fungal colonization. We compared the correlation between 20:1ω9 alone and AM fungal intensity with that between the total of three fatty acids and AM fungal intensity. The correlation coefficients were 0.751 (P < 0.01, n = 40) and 0.838 (P < 0.01, n = 40), respectively. This result indicates that the total of three fatty acids derived from G. rosea is closely related to the degree of root colonization by AM fungus ().
Figure 1. Correlation between the percentage of specific fatty acids derived from the arbuscular mycorrhizal (AM) fungus, Gigaspora rosea, and the intensity of the AM fungal colonization in the second lateral soybean roots. AM-specific fatty acids: the total of 20:1ω9, 20:4ω6, and 20:5ω3.
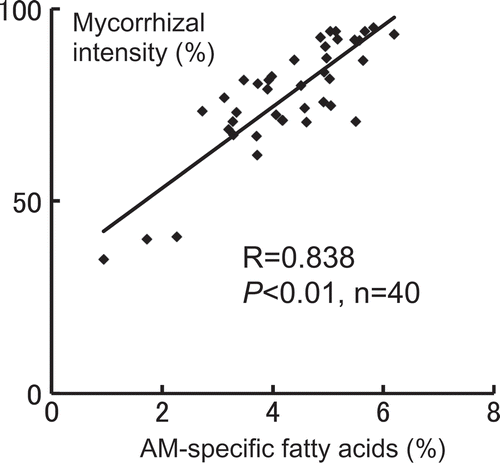
In all treatments, the percentage of AM-specific fatty acids in the first and second lateral roots of soybean plants increased with increasing cultivation duration (a and b). However, the percentage of AM-specific fatty acids was more than twofold higher in the second lateral roots than in the first lateral roots. Consequently, we speculate that the degree of root colonization by G. rosea was twofold higher in the second lateral roots than in the first lateral roots.
Figure 2. Changes in the percentage of specific fatty acids derived from arbuscular mycorrhizal (AM) fungus, Gigaspora rosea, in the first lateral roots (a) and the second lateral roots (b) of soybean plants at 6 and 9 weeks after sowing (%, n = 5). Enrei AM: Enrei with AM fungus alone; Enrei RAM: Enrei with rhizobium and AM fungus; Kanto100 AM: Kanto100 with AM fungus alone; Kanto100 RAM: Kanto100 with rhizobium and AM fungus; AM-specific fatty acids: the total of 20:1ω9, 20:4ω6, and 20:5ω3. For each sampling time point, different letters indicate significant differences as assessed by the Tukey–Kramer test (P < 0.05) after a one-way ANOVA. The absence of letters for a given sampling time point indicates no significant difference between the corresponding means.
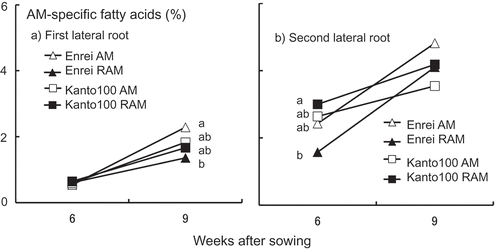
Based on the results obtained above, we suggest that the AM-specific fatty acids (i.e., 20:1ω9, 20:4ω6, and 20:5ω3) derived from G. rosea is useful markers for estimating the degree of root colonization by AM fungi. So far, it has been difficult to determine the degree of root colonization by AM fungi within the thick root, such as the first lateral root of soybean, using trypan blue staining method. We succeeded in solving this problem by use of AM-specific fatty acids, a biochemical marker, in this study.
The chitin and ergosterol have been used as such a marker for estimating root colonization by fungi. Each marker, however, has some problems for AM fungi. Both AM fungi and non-AM fungi within plant roots has chitin in their cell wall. Therefore, the root colonization by AM fungi based on the chitin determination may be overestimated (Slyvia Citation1994; Olsson Citation1999). Moreover, AM fungi contain little or no detectable ergosterol (Fontaine et al. Citation2001; Olsson et al. Citation2003). However, as shown in this study, the use of AM-specific fatty acids allows the accurate estimation of AM fungal colonization within the host root. In addition, the development of MIDI system provides a high-throughput analysis of the fatty acids.
Recently, Kobae and Ohtomo (Citation2016) established a WGA (wheat germ agglutinin)-HRP (horseradish peroxidase)-DAB (3,3ʹ-diaminobenzidine) staining method for the bright-field imaging of AM fungal mycelia within roots of soybean and other plant species. In this method, WGA-conjugated HRP, which specifically targets N-acetylglucosamine polymers and detects AM fungal cell walls, penetrated the cortical layers of 10% potassium hydroxide-treated host roots and stained AM fungal mycelia in the presence of DAB and hydrogen peroxide. This staining method provides high contrast and low background compared to that by trypan blue staining method and allows the recognition of symbiotic structure of AM fungi under low microscopic magnification. However, the cost of commercially available WGA-HRP and DAB reagents is very high. Authors stated that the cost reduction of WGA-HRP is a future challenge. Probably it takes more time for the WGA-HRP-DAB method to spread out widely.
In this study, we evaluated the efficacy of AM-specific fatty acids as a biochemical marker by use of G. rosea, which has three AM-specific fatty acids (20:1ω9, 20:4ω6, and 20:5ω3). Additional studies to examine the case of host roots colonizing the AM fungi with other specific fatty acids, e.g., 16:1ω5, are required for the future. Moreover, further investigation on the case of the host roots colonizing the various AM fungi simultaneously is needed in order to adapt the use of AM-specific fatty acids to the field study.
Acknowledgments
We thank Dr. Ryo Yamamoto (National Agriculture and Food Research Organization, Japan) for providing the soybean seeds.
Disclosure statement
The authors have no conflicts of interest to declare.
Additional information
Funding
References
- Akao S, Kouchi H 1992: A supernodulating mutant isolated from soybean cultivar Enrei. Soil Sci. Plant Nutr., 38, 183–187. doi:10.1080/00380768.1992.10416966.
- Arai M, Hayashi M, Takahashi M, Shimada S, Harada K 2005: Expression and sequence analysis of systemic regulation gene for symbiosis, NTS1/GmNARK in supernodulating soybean cultivar, Sakukei 4. Breed Sci., 55, 147–152. doi:10.1270/jsbbs.55.147.
- Augé RM 2001: Water relations, drought and vesicular-arbuscular mycorrhizal symbiosis. Mycorrhiza., 11, 3–42. doi:10.1007/s005720100097.
- Beilby JP, Kidby DK 1980: Sterol composition of ungerminated and germinated spores of the vesicular-arbuscular mycorrhizal fungus. Glomus Caledonius. Lipids., 15, 375–378. doi:10.1007/BF02533554.
- Bentivenga SP, Morton JB 1994: Stability and heritability of fatty acid methyl ester profiles of glomalean endomycorrhizal fungi. Mycol. Res., 98, 1419–1426. doi:10.1016/S0953-7562(09)81073-3.
- Bentivenga SP, Morton JB 1996: Congruence of fatty acid methyl ester profiles and morphological characters of arbuscular mycorrhizal fungi in Gigasporaceae. Proc. Natl. Acad. Sci. USA., 93, 5659–5662. doi:10.1073/pnas.93.11.5659.
- Caetano-Anollés G, Gresshoff PM 1991: Plant genetic control of nodulation. Ann. Rev. Microbiol., 45, 345–382. doi:10.1146/annurev.mi.45.100191.002021.
- Delves AC, Mathews A, Day DA, Carter AS, Carroll BJ, Gresshoff PM 1986: Regulation of the soybean-rhizobium nodule symbiosis by shoot and root factors. Plant Physiol., 82, 588–590. doi:10.1104/pp.82.2.588.
- Fontaine J, Grandmougin-Ferjani A, Hartmann M, Sancholle M 2001: Sterol biosynthesis by the arbuscular mycorrhizal fungus Glomus intraradices. Lipids, 36, 1357–1363. doi:10.1007/s11745-001-0852-z.
- Graham JH, Hodge NC, Morton JB 1995: Fatty acid methyl ester profiles for characterization of glomalean fungi and their endomycorrhizae. Appl. Environ. Microbiol., 61, 58–64.
- Isoi T 1997: Comparison of arbuscular mycorrhizal fungal flora under different cropping systems in a Light-colored Andosol of Japan. Soil Microorganisms, 50, 61–64.
- Jabaji-Hare S 1988: Lipid and fatty acid profiles of some vesicular-arbuscular mycorrhizal fungi: contribution to taxonomy. Mycologia., 80, 622–629. doi:10.2307/3807710.
- Jansa J, Gryndler M, Matucha M 1999: Comparison of the lipid profiles of arbuscular mycorrhizal (AM) fungi and soil saprophytic fungi. Symbiosis, 26, 247–264.
- Khalil S, Loynachan TE, McNabb HS 1992: Colonization of soybean by mycorrhizal fungi and spore populations in Iowa soils. Agron. J., 84, 832–836. doi:10.2134/agronj1992.00021962008400050014x.
- Kobae Y, Ohtomo R 2016: An improved method for bright-field imaging of arbuscular mycorrhizal fungi in plant roots. Soil Sci. Plant Nutr., 62, 27–30. doi:10.1080/00380768.2015.1106923.
- Madan R, Pankhurst C, Hawke B, Smith S 2002: Use of fatty acids for identification of AM fungi and estimation of the biomass of AM spores in soil. Soil Biol. Biochem., 34, 125–128. doi:10.1016/S0038-0717(01)00151-1.
- Nishimura R, Hayashi M, Wu G-J et al. 2002: HAR1 mediates systemic regulation of symbiotic organ development. Nature, 420, 426–429. doi:10.1038/nature01231.
- Olsson PA 1999: Signature fatty acids provide tools for determination of the distribution and interactions of mycorrhizal fungi in soil. FEMS Microbiol. Ecol., 29, 303–310. doi:10.1111/j.1574-6941.1999.tb00621.x.
- Olsson PA, Bååth E, Jakobsen I 1997: Phosphorus effects on the mycelium and storage structures of an arbuscular mycorrhizal fungus as studied in the soil and roots by analysis of fatty acid signatures. Appl. Environ. Microbiol., 63, 3531–3538.
- Olsson PA, Bååth E, Jakobsen I, Söderström B 1995: The use of phospholipids and neutral lipid fatty acids to estimate biomass of arbuscular mycorrhizal fungi in soil. Mycol. Res., 99, 623–629. doi:10.1016/S0953-7562(09)80723-5.
- Olsson PA, Francis R, Read DJ, Söderström B 1998: Growth of arbuscular mycorrhizal mycelium in calcareous dune sand and its interaction with other soil microorganisms as estimated by measurement of specific fatty acids. Plant Soil., 201, 9–16. doi:10.1023/A:1004379404220.
- Olsson PA, Larsson L, Bago B, Wallander H, Van Aarle IM 2003: Ergosterol and fatty acids for biomass estimation of mycorrhizal fungi. New Phytol., 159, 7–10. doi:10.1046/j.1469-8137.2003.00810.x.
- Pacovsky RS 1989: Metabolic differences in Zea-Glomus-Azospirillum symbioses. Soil Biol. Biochem.., 21, 953–960. doi:10.1016/0038-0717(89)90087-4.
- Pacovsky RS, Fuller G 1988: Mineral and lipid composition of Glycine-Glomus-Bradyrhizobium symbioses. Physiol. Plant., 72, 733–746. doi:10.1111/j.1399-3054.1988.tb06373.x.
- Porcel R, Barea JM, Ruiz-Lozano JM 2003: Antioxidant activities in mycorrhizal soybean plants under drought stress and their possible relationship to the process of nodule senescence. New Phytol., 157, 135–143. doi:10.1046/j.1469-8137.2003.00658.x.
- Rajapakse S, Miller JC 1994: Methods for studying vesicular-arbuscular mycorrhizal root colonization and related root physical properties. In Techniques for Mycorrhizal Research, Norris JR, Read D, Varma AK eds, 761–776. Academic Press, London.
- Sakamoto K, Iijima T, Higuchi R 2004: Use of specific phospholipid fatty acids for identifying and quantifying the external hyphae of the arbuscular mycorrhizal fungus Gigaspora rosea. Soil Biol. Biochem., 36, 1827–1834. doi:10.1016/j.soilbio.2004.04.037.
- Sakamoto K, Nohara Y 2009: Soybean (Glycine max [L.] Merr.) shoots systemically control arbuscule formation in mycorrhizal symbiosis. Soil Sci. Plant Nutr., 55, 252–257. doi:10.1111/j.1747-0765.2009.00358.x.
- Sakamoto K, Ogiwara N, Kaji T 2013: Involvement of autoregulation in the interaction between rhizobial nodulation and AM fungal colonization in soybean roots. Biol. Fertil. Soils., 49, 1141–1152. doi:10.1007/s00374-013-0804-8.
- Sakamoto K, Shishido M, Tsukui M, Iwamoto I 2005: Use of fatty acid profiles analyzed by MIDI system for detecting crop-root colonization of arbuscular mycorrhizal fungi. Jpn. J. Soil Sci. Plant Nutr., 76, 317–320. in Japanese.
- Schenck NC, Kinloch RA 1980: Incidence of mycorrhizal fungi on six field crops in monoculture on a newly cleared woodland site. Mycologia., 72, 445–456. doi:10.2307/3759518.
- Shrihari PC, Sakamoto K, Inubushi K, Akao S 2000: Interaction between supernodulating or non-nodulating mutants of soybean and two arbuscular mycorrhizal fungi. Mycorrhiza., 10, 101–106. doi:10.1007/s005720000064.
- Slyvia DM 1994: Quantification of external hyphae of vesicular-arbuscular mycorrhizal fungi. In Techniques for Mycorrhizal Research, Norris JR, Read D, Varma AK eds., 513–525. Academic Press, London.
- Smith SE, Read DJ 2008: Mycorrhizal Symbiosis, 3rd Edn., Academic Press, London.
- Takahashi M, Shimada S, Nakayama N, Arihara J 2005: Characteristics of nodulation and nitrogen fixation in the improved supernodulating soybean (Glycine max (L.) Merr.) cultivar ‘Sakukei 4ʹ. Plant Prod. Sci., 8, 405–411. doi:10.1626/pps.8.405.
- Trouvelot A, Kough JL, Gianinazzi-Pearson V 1986: Mesure du taux de mycorhization VA d`un systéme radiculaire. Recherche de méthodes d`estimation ayant une signification fonctionnelle. In Physiological and Genetical Aspects of Mycorrhizae, Gianinazzi-Pearson V, Gianinazzi S eds, 217–221. INRA Press, Paris.