ABSTRACT
Pruning is a regular management practice performed in tea fields, but its effects on soil properties and microbial community structures have not been well explored. Effects of tea pruned material, tea extracts, and tea polyphenols application on soil properties and microbial characteristics, including pH, soil enzyme activities, culturable microbial population, and bacterial community, were investigated in a pot-incubation experiment lasting for 10 weeks. Results indicated that returning of tea pruned material or its extracts significantly increased the soil pH as compared to control. The soil invertase and urease activities, as well as the culturable microbial population treated with pruned material were much higher than those in control, while polyphenol oxidase activity was similar among different treatments. Denaturing gradient gel electrophoresis analysis of bacterial community indicated that the Shannon’s diversity index (H) of all nine treatments receiving additives slightly decreased as compared to control. Pruning returning specifically induced several beneficial bacterial species, including Brevibacterium sp. and Burkholderia sp. In conclusion, pruning returning alleviated soil acidification, induced soil enzyme activities, stimulated culturable microbial population, and reshaped the microbial community. This research suggests that this management could be applied as an alternative agricultural practice in tea cultivation.
1. Introduction
As an important economic crop, tea (Camellia sinensis L.) is widely planted in acidic soil in the tropical and sub-tropical zones of China (Xue et al. Citation2006). In the tea cultivation, pruning is a common management practice performed to maintain the tea bushes in an elite condition for plucking, enhance the production by increasing branching, improve the tea quality, and suppress the incidence of pests and diseases (Yilmaz et al. Citation2004; Maudu et al. Citation2010). The pruned material, including branches and leaf, contains considerable quantities of nutrients; thus returning of the pruned material to the field soil was found to improve soil physical properties, organic matter, and availability of nutrients (Weeraratna et al. Citation1977). While some studies showed that the decomposition of the accumulated pruning and litter would produce allelopathic substances (phenolic compounds, alkaloids and flavonoids), which might restrain the microbial activity (Souto et al. Citation2000). However, the influence of tea pruned material on the soil properties and soil microbial characteristics has not been well explored.
Soil microbes are very important participators in nutrient cycling, soil organic matter decomposition and other relevant functions (Bastida et al. Citation2006). Soil microbial activity is regularly used as an indicator of soil quality and fertility that is mainly reflected by the community function (e.g., soil enzyme), which could be significantly and differently affected by organic and inorganic amendments (Parham et al. Citation2003). It is known that soil microbial community is sensitive to the changes in soil chemical properties (Tokuda and Hayatsu Citation2002). Lime application has been reported to cause a significant increase in pH of acidic tea orchard soils, and stimulated the BIOLOG functional diversity indexes, the structural diversity index of soil microbial community, and the soil-bacterial phospholipid fatty acid content (Xue et al. Citation2010). A long-term study indicated that the both the soil pH and bacterial genetic diversity index in tea orchard soils were lower than those that in wasteland (Zhao et al. Citation2012).
Based on the close relationship to soil biology, ease of measurement, and rapid response to changes of soil management, soil enzymes have also been suggested as potential indicators of soil quality (Bandick and Dick Citation1999; Karasawa et al. Citation2015). Especially, extracellular enzymes play important roles in litter decomposition and nutrient cycling in forest ecosystems (Sinsabaugh et al. Citation1994). Invertase regulates C cycling by catalyzing sucrose hydrolysis, which is usually used as an indicator to evaluate the soil capability of decomposing complex organic compounds (e.g., litter) into subunits that can be assimilated by microorganisms or plants (Hu et al. Citation2010). Urease, which catalyzes urea hydrolysis, is very important in the N-cycle, and is often used to evaluate the ability of nitrogen supply (Andrews et al. Citation1989; Mukumbareza et al. Citation2016). Wang et al. (Citation2011) reported that all forms of nitrogen fertilization induced the soil enzyme activities during the litter decomposition. Another study revealed that the soil enzyme activities increased significantly in mixed leaf litter treatments (Hu et al. Citation2006). In a word, soil enzymatic activities and microbial population size are closely correlated and revealed relevant response to environmental factors (Zaman et al. Citation1999).
In consequence of the limited information regarding influence of tea pruning returning on soil environment, including soil physical/chemical properties and microbial communities/functions, this study was performed to evaluate the effects of tea pruned material, its water extracts, and tea polyphenols (the important chemical in tea pruned material that are known to significantly affect the soil microbial activity) application on soil pH and microbial characteristics [soil enzyme activities, culturable microbial population assessed by plate counting, and bacterial community structure by denaturing gradient gel electrophoresis (DGGE)]. A scientific hypothesis is proposed in this paper that tea pruning returning is helpful for alleviation of soil acidification and stimulation of soil microbial activity, suggesting that it could be applied as a substantial management strategy in tea cultivation.
2. Materials and methods
2.1. Soil collection and physicochemical properties
The present study was performed in Jiangsu province, China, where the famous Chinese green tea, Nanjing Yuhua, is produced. The area is characterized by a subtropical monsoon climate with a mean annual temperature of 15.4°C and mean annual precipitation of about 1200 mm, with four distinct seasons. The soil used for experiments (depth of 0–20 cm) was collected from a tea field of Nanjing Agricultural University (latitude N 32°03ʹ, longitude E 118°50ʹ), where tea plants have been planted for 10 years. After passing through a 2-mm sieve to remove plant roots, leaves, stones, and other particles, the soil was homogenized before pre-incubation (Wang et al. Citation2011). The soil belonged to Acrisol (WRB Citation2014) and had a physical clay (<0.01 mm) content of approximately 40%. The physical and chemical properties of the soil are: total organic carbon 18.70 g kg−1, total nitrogen 1.05 g kg−1, total phosphorus 322.80 mg kg−1, cation exchange capacity 196.73 mmol kg−1, and pH 3.40.
2.2. Experimental design
The soil samples were adjusted to 60% water-holding capacity and pre-incubated at room temperature (25°C) for two weeks before the study. Pruned material, the mixture of mature leaves and twigs, was collected from tea field. The tea polyphenols content of the pruned material was 9.54%, as determined by the ferric tartrate colorimetric method (Turkmen et al. Citation2006). Briefly, 3 g of tea pruned material was infused with 450 mL of freshly boiled water and heated in a water-bath for 45 min. 1 mL of tea extract was transferred into a 25 mL volumetric flask for reaction with 5 mL dyeing solution (0.1% ferrous sulfate and 0.5% potassium sodium tartrate tetrahydrate), 4 mL distilled water and 15 mL buffer (0.067 M Na2HPO4, 0.067 M KH2PO4, pH 7.5). The absorbance of reaction solution was monitored at 540 nm in a 1 cm light-path cell by a spectrophotometer. The concentrated water extracts of the pruned material were obtained by boiling for 20 min, filtration, and rotary evaporation. The tea polyphenols content of the concentrated water extracts of the pruned material was 3.05%. In this study, nine treatments were set up and divided into three groups. Group A (Pruning): Soil was supplemented with pruned material at the rates of 5 (A1), 10 (A2), and 20 (A3) g kg−1, corresponding to the levels of approximately 3, 6, and 12 years of litter fall input in a normal green tea field (Ruan et al. Citation2004). The polyphenol concentration in the pruned material was determined as 9.54%, and the polyphenol inputs were calculated as approximately 0.48, 0.95, and 1.91 g kg−1 soil, for A1, A2, and A3, respectively. Group B (Water extract): Soil was supplemented with the concentrated water extracts of the equal pruned material described earlier at the rate of 16.67 (B1, i.e., extracts of 5 g pruned material, the same below), 33.33 (B2), and 66.67 (B3) mL kg−1, respectively, which was corresponding to the same input of those of A1, A2, and A3, respectively. The polyphenol inputs were calculated as approximately 0.51, 1.02, and 2.03 g kg−1 soil, for B1, B2, and B3, respectively. Group C (Tea polyphenols): Soil was supplemented with tea polyphenols with the final concentrations of 0.48 (C1), 0.96 (C2), and 1.92 (C3) g kg−1, corresponding to the same input of those of A1, A2, and A3, respectively. The tea polyphenols used here were purchased from Melting Point Food Science & Technology Ltd. (Nanjing, China), and the tea polyphenols content was more than 98%. This product was extracted from natural tea, which contained eight kinds of catechin monomer that approximately account for more than 70% of the compound. The soil without any supplementation was set up as the negative control. Each treatment included three replications, and each pot contained 2 kg of soil. All pots, covered by a polyethylene film to maintain the moisture, but allow gas exchange, were incubated at the room temperature for 10 weeks. During the incubation period, the soil moisture content was monitored and adjusted to 60% water-holding capacity by adding distilled water. Samples were collected from each pot as replicates at 0, 2, 4, 6, and 10 weeks after incubation for pH determination, soil enzyme assays and culturable microbial population investigation. After the 10-weeks incubation, the soil microbial communities were assessed by DGGE.
2.3. pH determination
Soil pH was determined by a glass electrode (1:2.5 soil-to-water ratio) after shaking the collected soil samples for 30 min to attain equilibrium (Dick et al. Citation2000).
2.4. Assessment of invertase, urease, and polyphenol-oxidase (PPO) activity
The activities of three important soil enzymes, including invertase (EC 3.2.1.26) and urease (EC 3.5.1.5) participated in C/N cycle, as well as polyphenol-oxidase (PPO; EC 1.10.3.1) that involved in oxidation of polyphenol substrates, were subsequently determined following the previous reported protocols (Perucci et al. Citation2000; Hu et al. Citation2006; Pan and Yu Citation2011) for evaluation of soil microbial activity in response to different treatments. Invertase activity was assessed using sucrose as the substrate. Two gram of soil, 15 mL 8% sucrose solution, 5 mL phosphate buffer (pH 5.5), and 5 drops of toluene were added to a 50-mL flask. After uniform mixing, the suspension was incubated at 37°C for 24 h; then 1 mL filtrate of this suspension was added to a 50-mL flask and heated for 5 min with 3 mL 3, 5-dinitrosalicylic acid. The color was measured by colorimetric assay at 508 nm, and the enzyme activity was expressed as mg glucose g−1 soil 24 h−1 (Hu et al. Citation2006). For urease activity assessment, 5 g of soil and 1 mL toluene was mixed in a 50-mL flask for a 15-min incubation, then 10 mL of 10% urea (w/v) and 20 mL citrate buffer (pH 6.7) were added and mixed uniformly. After incubation for another 24 h at 37°C, 3 mL filtrate of the mixture was used for the measurement. The color was determined colorimetrically at 578 nm and the activity is expressed as mg NH3–N g−1 soil 24 h−1 (Pan and Yu Citation2011). For PPO (EC 1.10.3.1), 1 g soil and 10 mL 1% pyrogallol solution was added to a 150-mL; after incubated at 30°C for 2 h, 4 mL citrate-phosphate buffer (pH 4.5) and 35 mL ether were added into the flask, and extracted for 30 min. The color was determined colorimetrically at 430 nm and the activity was expressed as micromoles of catechol oxidized 10 min−1 g−1 soil (Perucci et al. Citation2000).
2.5. Investigation of the population of culturable soil bacteria and fungi by plate counting
Ten grams of the collected soil was separately placed in 90 mL sterile distilled water and shaken for 30 min to make the soil suspension. Colony forming unit (CFU) counts were obtained by serial dilutions of the soil suspensions on beef-protein medium (beef extract 3.0 g, peptone 10.0 g, sodium chloride 5.0 g, agar 18.0 g, 1000 mL distilled water, pH 7.0–7.2) sterilized at 121°C for 20 min, for bacteria and on Rose Bengal medium (peptone 5.0 g, glucose 10.0 g, KH2PO4 1.0 g, MgSO4 · 7H2O 0.5 g, rose bengal 0.03 g, agar 20.0 g, 1000 mL distilled water and 0.1 g chloramphenicol after sterilization) at 115°C for 30 min (Pan and Yu Citation2011) for fungi. Plates were incubated in dark at 28°C for fungi and 30°C for bacteria. The population of bacteria was counted after 2–4 days and fungi after 5–7 days of incubation, respectively. Four replicates were performed for each treatment.
2.6. PCR-DGGE analysis of the soil bacterial community
As the culturable microbes only represent a small population of whole soil microorganisms, PCR-DGGE was further performed for investigating the bacterial community affected by different treatments.
2.6.1. DNA extraction and PCR conditions
DNA was extracted from 0.3 g soil samples using the UltraClean soil DNA isolation kit (M-BIO gen) according to the manufacturer’s instructions. The extracted DNA was eluted into 50 µL sterilized double distilled water and stored at −20°C.
The bacterial 16S rRNA genes containing the V6-V8 region were amplified by using primers GC-968F:5ʹ-AACGCGAAGAACCTTAC-3ʹand 1401R: 5ʹ-GCGTGTGTACAAGACCC-3ʹ. A40-bp GC-clamp was added to the 5ʹ end of the primer 968F to obtain efficient separation in the DGGE profile (Su et al. Citation2008). The PCR reaction mixtures contained: 5.0 µL 10 × PCR buffer, 5.0 µl MgCl2 (2.5 mM), 5.0 µL dNTP mixture (2.5 mM in total), 1.0 µL each primer (10 PM), 0.3 µL Taq polymerase (5U Taq), and H2O in the total volume of 50 µL. PCR reaction was performed using the following cycle conditions: 94°C for 4 min, followed by 30 cycles each consisting of 94°C for 30 s, 59°C for 30 s, and 72°C for 45 s, then 72°C for 10 min.
2.6.2. DGGE analysis and DNA sequencing
The DGGE was performed with a Dcode Universal Mutation Detection System (BioRad Lab, LA, USA). In detail, 40 µL of the PCR production with GC-clamp and 4 µL of the loading buffer were loaded onto an 8% (w/v) acrylamide gels (acrylamide/bis solution, 37.5:1). The denaturants concentration (100% denaturing gel contains 7 M urea and 40% (v/v) deionized formamide) ranged from 45 to 65%. Electrophoresis was performed at 60°C for 14 h at 80 V in a DGGE chamber containing approximately 7 L of TAE buffer (0.04 M Tris-base, 0.02 M sodium acetate, and 1 mM EDTA; pH 8.0) (Xu et al. Citation2006). A total of 27 dominant DGGE bands were carefully excised and the DNA from each band was eluted in 10 μL sterilized double distilled water over night at 4°C. Then 1 µL eluted DNA was used as a template for PCR re-amplification with the same primers (without the GC clamp) and program as described earlier. The amplified DNA was cloned into Escherichia coli JM109 using the pMD18-T vector system (TaKaRa, Dalian, China) as described by the manufacturer. Clones with correct inserts that confirmed by gel electrophoresis were further sequenced (Zhao et al. Citation2012).
2.6.3. DGGE profile analysis
Analysis of the DGGE banding patterns were performed in the Quantity One (Quantity One 4.6.3, Bio-Rad). The diversity of bacterial community was assessed by Richness (S), Shannon index (H), and Evenness (EH) as described previously (Smit et al. Citation2001; Ge et al. Citation2008). Cluster analysis was performed with the un-weighted pair group method (UPGMA) to study the general patterns of bacterial similarity, as based on the band profiles from DGGE bands to create a similarity dendrogram between samples (Zhao et al. Citation2012).
2.6.4. Sequence analysis and phylogenetic tree construction
The DNA sequences obtained were searched against in the NCBI GenBank database using the BLAST program. The phylogenetic tree including all the DNA sequences, as well as the most similar GenBank sequences to the soil 16S rRNA gene clones, were constructed using the MEGA version 5 (Ge et al. Citation2008). Bootstrap values of 80% or greater were used for defining well-supported clusters of the nucleotide sequences in the phylogenetic tree.
2.7. Statistical analysis
Differences among the treatments were calculated and statistically analyzed using the analysis of variance (ANOVA) and Duncan’s multiple range tests (P < 0.05). The SPSS version 19.0 (SPSS Inc., Chicago, IL) was used for the data analysis.
The nucleotide sequences of the DGGE bands are available in the NCBI database (Accession no. KJ718890-KJ718921).
3. Results
3.1. Effects of different treatments on soil pH
During the 10-weeks of incubation period, the soil pH values kept increasing in all nine treatments receiving additives as compared to control. Especially, three pruned material treatments in group A increased the soil pH by 0.22–0.44 pH units ( and S1). While for the group C (tea polyphenols), only the pH in the soil treated with the lowest concentration of tea polyphenols (C1) was significantly higher than that in the control soil after 10-weeks of incubation.
Table 1. The effects of different treatments on soil pH, invertase activity, urease activity, and culturable microbial population at different sampling time during the incubation.
3.2. Effects of different treatments on invertase, urease, and polyphenol-oxidase (PPO) activities
All the nine treatments that received additives significantly stimulated the invertase activity compared to control, and the soil invertase activity reached its peak value after 2 weeks of incubation (Table S2). At the end of the incubation, the invertase activity levels in A2, A3, B1, and B3 treatments was 27.3%, 62.1%, 22.9%, and 26.1% higher than that of control, respectively. The invertase activity in group C (tea polyphenol) was similar to control ().
The soil urease activity showed some decrease after 6 weeks of incubation and recovered to the initial level at the end of the incubation (Table S3). After 10 weeks of incubation, the urease activity in A2 and A3 treatments only showed a significant increase of 12.9% and 14.0% compared to control, respectively. In contrast, the enzyme activity in group B (water extracts) and C (tea polyphenols) were found to be much lower than that in control, and the decrease degrees in B1, B2, B3, C1, C2, and C3 treatments were 13.6%, 22.7%, 23.5%, 9.8%, 15.4%, and 28.7%, respectively ().
The soil PPO activity in most treatments kept roughly stable with some moderate decrease during the incubation period. After 2-weeks of incubation, the PPO activity in treatment C3 (the treatment with highest concentrations of tea polyphenol) was significantly higher than those in all other treatments. During the incubation process of 2–6 weeks, most of the treatments in group A (pruning returning) and group B (water extracts) showed an increase in the enzyme activities compared to control. At the end of the experiment, the activity levels were similar among different treatments, except that in treatment A3 that revealed a significant increase compared to control (Table S4).
3.3. Effects of different treatments on microbial community
3.3.1. Plate counting of culturable soil microbial population
The bacteria populations revealed a fast increase at the beginning of the incubation period and then decreased after 6 weeks of incubation (Table S5). The population size in group A (pruning returning treatment) was significantly higher than that in control during the whole incubation period, and the population size increased with the increase of pruned material. The largest bacterial population was obtained in treatment A3, which was 2.38 times larger than that of control. The bacterial density in group B (extracts) was higher than that of control during first 4-weeks of incubation and then decreased sharply. After 10 weeks’ incubation, the population size in group B was decreased by 12.8%, 25.6%, and 44.4% in B1, B2, and B3 treatments compared to control, respectively. The group C (tea polyphenol treatment) significantly reduced the cultured bacteria population compared to control, and the lowest bacteria density was recorded in treatment C3, which was 23.9% lower than that in control ().
The trend of population size of the culturable fungi was similar to that of bacteria with some exceptions (Table S5). The group A, as well as treatment B2 and B3 revealed a significant 0.91–2.97 times increase in the fungal density compared to control after 10-weeks of incubation; while the culturable fungi population in treatment B1 and group C showed non-significant differences with that in the control ().
3.3.2. DGGE patterns of bacterial community
Totally, 27 dominant DGGE bands were detected and their intensities were determined by Quantity One software (). In general, the bands distribution was similar among different treatments, but the bacterial diversity in all nine treatments that received additives, were significantly lower than that in control, as revealed by the richness (number of the bands in each lane) and Shannon index (). However, the color depths of the bands in all treatments, especially in group A, were more intense than that in control, suggesting a larger bacterial population size. The UPGMA cluster analysis based on DGGE profiles suggest that the sampling locations could be divided into two groups. Group 1 contained control, treatment group A, and treatment B1, and group 2 included treatment B2, B3, and group C ().
Table 2. Diversity properties of soil bacteria using DGGE bands pattern data associated with different treatments.
Figure 1. DGGE fingerprint profiles of PCR products targeting 16S rRNA gene. Lane CK to C3 represents different treatments after 10 weeks incubation (in a denaturing 45–65% gradient gel). Group A (Pruning): Soil was supplemented with pruned materials with the amounts of 5 (A1), 10 (A2), and 20 (A3) g kg−1; Group B (Water extract): Soil was supplemented with the concentrated water extracts of the pruning described above with amounts of 16.67 (B1), 33.33 (B2), and 66.67 (B3) mL kg−1; Group C (Tea polyphenols): Soil was supplemented with tea polyphenols with the final concentration of 0.48 (C1), 0.96 (C2), and 1.92 (C3) g kg−1; CK: control.
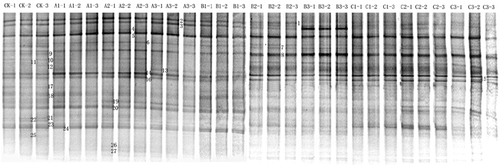
Figure 2. Dendogram showing clustering of bacterial DGGE profiles. UPGMA cluster analysis of DGGE profiles based on band intensities. The scale represents percent similarity. #1–#3 represents CK treatments; #4–#12 represents A treatments; #13–#21 represents B treatments; #21–#30 represents C treatments. For abbreviations of the samples, please see the legend for .
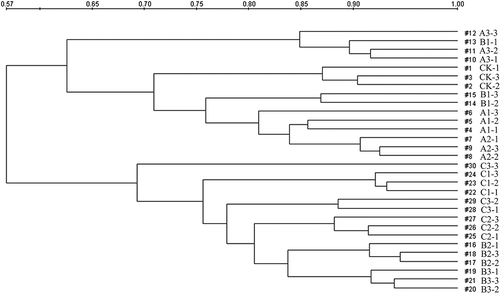
For further investigation of bacterial species, the DGGE dominant bands were re-amplified and sequenced, based on which a phylogenetic tree was constructed shown in . According to the relationships between these sequences, 27 dominant bands were grouped into 10 branches with different levels of relationship as: Bacteroidetes (band 21), beta-Proteobacterium (band 26), gamma-Proteobacterium (bands 2, 4, 6, 7, and 13), alpha Proteobacterium (band 19–1), Firmicutes (band 27–2), Actinobacteria-I (bands 3, 12, 22, and 27–1), Actinobacteria-II (bands 10 and 14), Verrucomicrobia (band 9), Uncultured bacterium (bands 11, 23, and 25), and Chloroflexi (bands 1, 5, 8, 15–1, 15–2, 16, 17, 18, 19–2, 20–1, 20–2, 24). Thoroughly, some bands (bands 2, 3, 5, 6, 8, 14, 16, 18, 20, 23, 24) that showed highest sequence homology to Gamma proteobacterium, Actinobacteria, Chloroflexi, and uncultured bacterium present in all treatments, but revealing some different depths. The bands 9, 17, and 25, only detected in control, were identified as those specific ones of uncultured bacteria. For group A of pruned material treatment, bands 7 and 12, identified as uncultured bacterium, both disappeared; while some bands (11, 22, 26, and 27), identified as those of uncultured bacteria, Brevibacterium sp., Burkholderia sp, and Staphylococcus epidermidis, respectively, were particularly detected in group A. Similarly, band 22 could only be found in group B and C.
Figure 3. Neighbor-joining phylogenetic tree representing the phylogenetic relationship of the 16S rDNA PCR-DGGE bands to the most closely related identified sequences downloaded from NCBI by BLAST searches. The tree was constructed based on the V6–V8 region of the 16 rRNA genes. A bootstrap analysis was performed with 1,000 repetitions and values greater than 50% are shown.
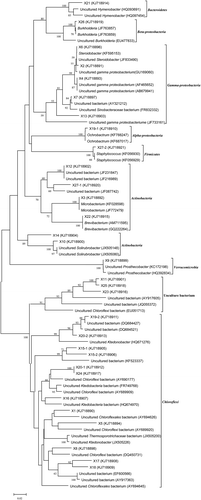
4. Discussion
The polyphenol concentrations in the pruned tea material was 9.54%, while that in the water extracts was about 3.05% (16.67 mL water for extraction of the tea polyphenol from 5 g pruned material), indicating that the tea polyphenol concentration in water extracts was approximately same as that in the pruned material. Also assuredly, the protocol used in this experiment for phenolic extract couldn’t represent the natural chemical composition in the pruned materials, and it was performed as a ‘pruned material water extracts’ treatment for the comparison of its effects on the soil characters and microbial community with ‘pruned material’ and ‘polyphenols (equal concentration with those in pruned material)’.
4.1. Alleviation of soil acidification by tea pruning returning
It is widely believed that the cultivation of tea plants can result in soil acidification, probably because root secretion of organic acids and release of H+ caused by uptake of aluminum (Al) (Zhao et al. Citation2012). Some researchers speculated that the tea pruned material, which accumulates large amounts of Al, would accelerate the soil acidification (Li et al. Citation2010; Wang et al. Citation2010b; Wang et al. Citation2013). However, Wang et al. (Citation2009) reported that incorporation of leguminous crop residues could be applied as an alternative for raising the pH of tea garden soils, as the soil pH was increased from 4.33 to 5.02 after 65 days of incubation. Another study also highlighted the increase of soil pH during litter decomposition (Haynes and Mokolobate Citation2001). Results obtained in this study showed that returning of tea pruning or its extracts significantly increased soil pH ( and S1), which was consistent with these findings, and the probable reasons could be: (i) Release of the tea alkali and caffeine from tea leaf (Pan et al. Citation2003). (ii) Release of the basic cations from the plant residues, which was reported to be directly correlated with the increase of soil pH (Xu et al. Citation2006). (iii) The oxidation of organic anions during decomposition of leaf material could consumed the protons, thus increased the soil pH (Yan and Schubert Citation2000).
4.2. Pruning returning activated soil enzyme activity but not water extracts or tea polyphenols
As the tea polyphenols in pruned material are known to be toxic to soil microbes (Pandey and Palni Citation1996), it has been proposed that the pruned material would restrain the soil microbial activity. Soil enzymes produced by soil microbes are usually used as indicator of microbial activity and soil quality. In this study, the activities of invertase and urease in pruned material treatment were significantly higher than that in control, especially in high application amount (, S2, and S3). Previous work reported that application of maize straw could yield maximal invertase activity (Kandeler et al. Citation1999), and urease activity was positive correlated with N fertilization in most cases during litter decomposition (Wang et al. Citation2010a). Here, we speculated that the induced activities were attributed to the stimulated microbial activity caused by increased soil pH, which could then improve the soil C/N cycle and fertility (Hu et al. Citation2006; Aciego Pietri and Brookes Citation2008). The fact that the average enzyme activities were decreased in an order of group A, B, and C could be attributed to the easily diffused antibiotic compounds (e.g., tea polyphenols) in group B and C; the potential nutrients in the tea pruned material could also play a role but this hypotheses still need further investigation (Weeraratna et al. Citation1977).
PPO, including peroxidases, laccase, etc., are the key enzymes involved in the plant residue degradation (Alarcón-Gutiérrez et al. Citation2009). In this study, the significant increase of PPO activity in treatment C3 after 2-weeks of incubation suggested high concentrations of tea polyphenols might induce the enzyme activity (Table S4). On the other hand, tea pruned returning also up-regulated the PPO activity during the first 6 weeks of incubation, as compared to control, which was consistent with the report that PPO activity increased along with the degradation of plant residues and lignin (Fioretto et al. Citation2007). In general, the PPO plays an important role in degradation and oxidation of both the lignin and tea polyphenols during the incubation period, but the activity and detailed roles of different kinds of PPOs involved in the process need further exploration.
4.3. Pruning returning induced soil culturable microbial population
The highest abundances of both culturable bacteria and fungi in Group A as compared with other treatments implicated the coincide between culturable microbial density and their activity, which might be attributed to the ameliorative soil acidification (). Similarly, a previous study revealed that the application of plant residues, including hay and wheat straw, would increase the enumeration of culturable bacteria and fungi (Shahsavari et al. Citation2013). Moreover, the rise of the culturable microbial population at the initial of the incubation in groups A and B but not in group C or control suggest that the pruned material and its extracts probably provide available nutrients for the soil microbes (Weeraratna et al. Citation1977), and the following decrease of microbial population might be due to consumption of the nutrients and release of the antimicrobial substances (Table S5). However, additional measurement is needed to confirm this hypothesis.
4.4. Reshaping of bacterial community in response to pruning returning as evaluated by DGGE
Because of the serious limitation on studying microbial community by culture-dependent plate counting, DGGE analysis was further performed for the comprehensive investigation of the microbial community. The finding that Proteobacteria, Actinobacteria, and Firmicutes were the dominant phylum detected in the soil () was in agreement with the previous studies (Xue et al. Citation2006; Zhao et al. Citation2012). It was reported that application of straw residue or soil amendments (pea straw mixed) could increase the soil bacterial and fungal diversity (Shahsavari et al. Citation2013; Yuan et al. Citation2013). However, in this study, the Shannon’s diversity index in all treatments was lower than control (), suggesting that some compounds in the pruned material and water extracts, such as soluble polyphenols or tannin in litter material, might slightly suppress the microbial community diversity (Kraus et al. Citation2003; Zhao et al. Citation2012). Further sequence analysis indicated that most of the disappeared bands in treatments (9, 17, 25) or that only disappeared in group A (7, 12) were identified to show highest homology to uncultured bacterium and Chloroflexi (), of which the functions were unknown or unconsidered (Bulgarelli et al. Citation2012).
On the other hand, bands 22 and 26, which were identified as the specific ones of Brevibacterium sp. and Burkholderia sp., respectively, were particularly detected in group A. Brevibacterium sp. is a Gram-positive (Halgasova et al. Citation2002), rod-shaped bacteria, which could be involved in organic material decomposition (Lu et al. Citation2003), production of siderophores (Noordman et al. Citation2006), and suppression of plant diseases (Kati et al. Citation2008). The genus Burkholderia belongs to the beta-Proteobacteria class (Bontemps et al. Citation2010), and is one of numerous species of plant growth-promoting bacteria, which could both promote plant growth and repress soil-borne fungal pathogens (Saleem et al. Citation2007). Burkholderia could adapt to infertility and acidity and reveals the potential of N-fixation on intractable soils (Garau et al. Citation2009).
In general, all treatments slightly repressed the soil microbial diversity and reshaped the community, which might be due to the release of tea polyphenol or other antibacterial compounds. However, the major disappeared species were those with unknown or unconsidered functions. Some beneficial bacterial species could also be detected in group A. In addition, the tea pruned material treatment, but not group B and C stimulated the cultured microbial population and enzyme activity, implicating that some antibacterial compounds, such as polyphenols released more slowly, and their repressive effects on microbes could be counteracted by the ameliorative soil pH.
5. Conclusion
Application of pruned tea material, water extracts and tea polyphenols all increased the soil pH as compared with control. Pruning returning significantly stimulated the activities of soil invertase and urease, and soil culturable microbial populations when comparing to other treatments. PCR-DGGE analysis indicated that pruning application reshaped the bacterial community, as induced several beneficial species and eliminated uncultured microbes. The activation of soil enzyme activity and culturable microbial population, as well as the positive regulation on bacterial community by pruning returning, could be attributed to increased soil pH and probably slow release of the antibiotic compounds. Based on these findings, it could be suggested that the pruned tea material could be applied as an alternative strategy in tea cropping for alleviation of soil acidification and activation of microbial activity.
Acknowledgements
This study was supported by the earmarked fund for Modern Agro-industry Technology Research System of China (CARS-23), Science and Technology Plan of Jiangsu Province of China (BE2013426), and Suzhou engineering research center for the modern ecological tea industry of China (SZGD201067).
Additional information
Funding
References
- Aciego Pietri JC, Brookes PC 2008: Relationships between soil pH and microbial properties in a UK arable soil. Soil Biol. Biochem., 40, 1856–1861. doi:10.1016/j.soilbio.2008.03.020
- Alarcón-Gutiérrez E, Floch C, Augur C, Petit JL, Ziarelli F, Criquet S 2009: Spatial variations of chemical composition, microbial functional diversity, and enzyme activities in a Mediterranean litter (Quercus ilex L.) profile. Pedobiologia, 52, 387–399. doi:10.1016/j.pedobi.2009.01.002
- Andrews RK, Blakeley RL, Zerner B 1989: Urease: a Ni (II) metalloenzyme. In The Bioinorganic Chemistry of Nickel, V.C.H. Publishers, New York, pp. 141–166.
- Bandick AK, Dick RP 1999: Field management effects on soil enzyme activities. Soil Biol. Biochem., 31, 1471–1479. doi:10.1016/S0038-0717(99)00051-6
- Bastida F, Luis Moreno J, Teresa H, García C 2006: Microbiological degradation index of soils in a semiarid climate. Soil Biol. Biochem., 38, 3463–3473. doi:10.1016/j.soilbio.2006.06.001
- Bontemps C, Elliott GN, Simon MF et al. 2010: Burkholderia species are ancient symbionts of legumes. Mol. Ecol., 19, 44–52. doi:10.1111/j.1365-294X.2009.04458.x
- Bulgarelli D, Rott M, Schlaeppi K et al. 2012: Revealing structure and assembly cues for Arabidopsis root-inhabiting bacterial microbiota. Nature, 488, 91–95. doi:10.1038/nature11336
- Dick WA, Cheng L, Wang P 2000: Soil acid and alkaline phosphatase activity as pH adjustment indicators. Soil Biol. Biochem., 32, 1915–1919. doi:10.1016/S0038-0717(00)00166-8
- Fioretto A, Papa S, Pellegrino A, Fuggi A 2007: Decomposition dynamics of Myrtus communis and Quercus ilex leaf litter: Mass loss, microbial activity and quality change. Appl. Soil Ecol., 36, 32–40. doi:10.1016/j.apsoil.2006.11.006
- Garau G, Yates RJ, Deiana P, Howieson JG 2009: Novel strains of nodulating Burkholderia have a role in nitrogen fixation with papilionoid herbaceous legumes adapted to acid, infertile soils. Soil Biol. Biochem., 41, 125–134. doi:10.1016/j.soilbio.2008.10.011
- Ge Y, Zhang J, Zhang L, Yang M, He J 2008: Long-term fertilization regimes affect bacterial community structure and diversity of an agricultural soil in northern China. J. Soil. Sediment., 8, 43–50. doi:10.1065/jss2008.01.270
- Halgasova N, Bukovska G, Ugorcakova J, Timko J, Kormanec J 2002: The Brevibacterium flavum sigma factor SigB has a role in the environmental stress response. FEMS Microbiol. Lett., 216, 77–84. doi:10.1111/j.1574-6968.2002.tb11418.x
- Haynes RJ, Mokolobate MS 2001: Amelioration of Al toxicity and P deficiency in acid soils by additions of organic residues: a critical review of the phenomenon and the meachnisms involved. Nutr. Cycl. Agroecosys., 59, 47–63. doi:10.1023/A:1009823600950
- Hu J, Lin X, Wang J, Dai J, Chen R, Zhang J, Wong MH 2010: Microbial functional diversity, metabolic quotient, and invertase activity of a sandy loam soil as affected by long-term application of organic amendment and mineral fertilizer. J. Soil. Sediment., 11, 271–280. doi:10.1007/s11368-010-0308-1
- Hu Y, Wang S, Zeng D 2006: Effects of single Chinese fir and mixed leaf litters on soil chemical, microbial properties and soil enzyme activities. Plant Soil, 282, 379–386. doi:10.1007/s11104-006-0004-5
- Kandeler E, Luxhoi J, Tscherko D, Magid J 1999: Xylanase, invertase and protease at the soil–litter interface of a loamy sand. Soil Biol. Biochem., 31, 1171–1179. doi:10.1016/S0038-0717(99)00035-8
- Karasawa T, Takebe M, Sato F, Komada M, Nagaoka K, Takenaka M, Urashima Y, Nishimura S, Takahashi S, Kato N 2015: Trends of lettuce and carrot yields and soil enzyme activities during transition from conventional to organic farming in an Andosol. Soil Sci. Plant Nutr., 61, 295–311. doi:10.1080/00380768.2014.985577
- Kati H, Yilmaz H, Demirbag Z 2008: Isolation and identification of bacteria from Thaumetopoea pityocampa Den. and Schiff. (Lep., Thaumetopoeidae) and determination of their biocontrol potential. World J. Microb. Biot., 24, 3005–3015. doi:10.1007/s11274-008-9845-9
- Kraus TE, Dahlgren RA, Zasoski RJ 2003: Tannins in nutrient dynamics of forest ecosystems - a review. Plant Soil, 256, 41–66. doi:10.1023/A:1026206511084
- Li J, Wang N, Xu R, Tiwari D 2010: Potential of industrial byproducts in ameliorating acidity and aluminum toxicity of soils under tea plantation. Pedosphere, 20, 645–654. doi:10.1016/S1002-0160(10)60054-9
- Lu J, Sanchez S, Hofacre C, Maurer JJ, Harmon BG, Lee MD 2003: Evaluation of broiler litter with reference to the microbial composition as assessed by using 16S rRNA and functional gene markers. Appl. Environ. Microbiol., 69, 901–908. doi:10.1128/AEM.69.2.901-908.2003
- Maudu M, Mudau FN, Mariga IK 2010: The effect of pruning on growth and chemical composition of cultivated bush tea (Athrixia phylicoides D.C). J. Med. Plants Res., 4, 2353–2358.
- Mukumbareza C, Muchaonyerwa P, Chiduza C 2016: Bicultures of oat (Avena sativa L.) and grazing vetch (Vicia dasycarpa L.) cover crops increase contents of carbon pools and activities of selected enzymes in a loam soil under warm temperate conditions. Soil Sci. Plant Nutr., 62, 447–455. doi:10.1080/00380768.2016.1206833
- Noordman W, Reissbrodt R, Bongers R, Rademaker J, Bockelmann W, Smit G 2006: Growth stimulation of Brevibacterium sp. by siderophores. J. Appl. Microbiol., 101, 637–646. doi:10.1111/jam.2006.101.issue-3
- Pan J, Yu L 2011: Effects of Cd or/and Pb on soil enzyme activities and microbial community structure. Ecol. Eng., 37, 1889–1894. doi:10.1016/j.ecoleng.2011.07.002
- Pan X, Niu G, Liu H 2003: Microwave-assisted extraction of tea polyphenols and tea caffeine from green tea leaves. Chem. Eng. Process., 42, 129–133. doi:10.1016/S0255-2701(02)00037-5
- Pandey A, Palni LMS 1996: The rhizosphere effect of tea on soil microbes in a Himalayan monsoonal location. Biol. Fert. Soils, 21, 131–137. doi:10.1007/BF00335924
- Parham JA, Deng SP, Da HN, Sun HY, Raun WR 2003: Long-term cattle manure application in soil. II. Effect on soil microbial populations and community structure. Biol. Fert. Soils, 38, 209–215. doi:10.1007/s00374-003-0657-7
- Perucci P, Casucci C, Dumontet S 2000: An improved method to evaluate the o-diphenol oxidase activity of soil. Soil Biol. Biochem., 32, 1927–1933. doi:10.1016/S0038-0717(00)00168-1
- Ruan J, Ma L, Shi Y, Zhang F 2004: Effects of litter incorporation and nitrogen fertilization on the contents of extractable aluminium in the rhizosphere soil of tea plant (Camallia sinensis (L.) O. Kuntze). Plant Soil, 263, 283–296. doi:10.1023/B:PLSO.0000047744.44940.96
- Saleem M, Arshad M, Hussain S, Bhatti AS 2007: Perspective of plant growth promoting rhizobacteria (PGPR) containing ACC deaminase in stress agriculture. J. Ind. Microb. Biot., 34, 635–648. doi:10.1007/s10295-007-0240-6
- Shahsavari E, Adetutu EM, Anderson PA, Ball AS 2013: Plant residues—a low cost, effective bioremediation treatment for petrogenic hydrocarbon-contaminated soil. Sci Total Environ., 443, 766–774. doi:10.1016/j.scitotenv.2012.11.029
- Sinsabaugh R, Moorhead D, Linkins A 1994: The enzymic basis of plant litter decomposition: emergence of an ecological process. Appl. Soil Ecol., 1, 97–111. doi:10.1016/0929-1393(94)90030-2
- Smit E, Leeflang P, Gommans S, Van Den Broek J, Van Mil S, Wernars K 2001: Diversity and seasonal fluctuations of the dominant members of the bacterial soil community in a wheat field as determined by cultivation and molecular methods. Appl. Environ. Microbiol., 67, 2284–2291. doi:10.1128/AEM.67.5.2284-2291.2001
- Souto XC, Chiapusio G, Pellissier F 2000: Relationships between phenolics and soil microorganisms in spruce forests: significance for natural regeneration 2000: relationships between phenolics and soil microorganisms in spruce forests: significance for natural regeneration. J. Chem. Ecol., 26, 2025–2034. doi:10.1023/A:1005504029243
- Su Y, Yao W, Perez-Gutierrez ON, Smidt H, Zhu W 2008: 16S ribosomal RNA-based methods to monitor changes in the hindgut bacterial community of piglets after oral administration of Lactobacillus sobrius S1. Anaerobe, 14, 78–86. doi:10.1016/j.anaerobe.2007.12.004
- Tokuda S, Hayatsu M 2002: Soil microbial biomass and fluorescein diacetate hydrolytic activity in Japanese acidic tea field soils. Soil Sci. Plant Nutr., 48, 865–869. doi:10.1080/00380768.2002.10408713
- Turkmen N, Sari F, Velioglu YS 2006: Effects of extraction solvents on concentration and antioxidant activity of black and black mate tea polyphenols determined by ferrous tartrate and Folin–ciocalteu methods. Food Chem., 99, 835–841. doi:10.1016/j.foodchem.2005.08.034
- Wang C, Feng X, Guo P, Han G, Tian X 2010a: Response of degradative enzymes to N fertilization during litter decomposition in a subtropical forest through a microcosm experiment. Ecol. Res., 25, 1121–1128. doi:10.1007/s11284-010-0737-8
- Wang C, Han G, Jia Y, Feng X, Guo P, Tian X 2011: Response of litter decomposition and related soil enzyme activities to different forms of nitrogen fertilization in a subtropical forest. Ecol. Res., 26, 505–513. doi:10.1007/s11284-011-0805-8
- Wang H, Xu R, Wang N, Li X 2010b: Soil acidification of alfisols as influenced by tea cultivation in Eastern China. Pedosphere, 20, 799–806. doi:10.1016/S1002-0160(10)60070-7
- Wang L, Yang X, Rachel K, Wang Y, Tong D, Ye M, Jiang X 2013: Combined use of alkaline slag and rapeseed cake to ameliorate soil acidity in an acid tea garden soil. Pedosphere, 23, 177–184. doi:10.1016/S1002-0160(13)60005-3
- Wang N, Li J, Xu R 2009: Use of agricultural by-products to study the pH effects in an acid tea garden soil. Soil Use Manage., 25, 128–132. doi:10.1111/j.1475-2743.2009.00203.x
- Weeraratna C, Watson M, Wettasingha D 1977: Effect of mineralization of tea prunings on some soil characteristics. Plant Soil, 46, 93–99. doi:10.1007/BF00693116
- WRB. 2014. IWG 2014: world reference base for soil resources 2014. International soil classification system for naming soils and creating legends for soil maps. World Soil Resources Reports No. 106. FAO, Rome.
- Xu J, Tang C, Chen Z 2006: The role of plant residues in pH change of acid soils differing in initial pH. Soil Biol. Biochem., 38, 709–719. doi:10.1016/j.soilbio.2005.06.022
- Xue D, Huang X, Yao H, Huang C 2010: Effect of lime application on microbial community in acidic tea orchard soils in comparison with those in wasteland and forest soils. J. Environ. Sci-China, 22, 1253–1260. doi:10.1016/S1001-0742(09)60246-1
- Xue D, Yao H, Huang C 2006: Microbial biomass, N mineralization and nitrification, enzyme activities, and microbial community diversity in tea orchard soils. Plant Soil, 288, 319–331. doi:10.1007/s11104-006-9123-2
- Yan F, Schubert S 2000: Soil pH changes after application of plant shoot materials of faba bean and wheat. Plant Soil, 220, 279–287. doi:10.1023/A:1004712518406
- Yilmaz G, Kandemir N, Kinalioglu K 2004: Effects of different pruning intervals on fresh shoot yield and some quality properties of Tea (Camellia sinensis (L.) O. Kuntze) in Turkey. Pakistan J. Biol. Sci., 7, 1208–1212. doi:10.3923/pjbs.2004.1208.1212
- Yuan H, Ge T, Zhou P, Liu S, Roberts P, Zhu H, Zou Z, Tong C, Wu J 2013: Soil microbial biomass and bacterial and fungal community structures responses to long-term fertilization in paddy soils. J. Soil. Sediment., 13, 877–886. doi:10.1007/s11368-013-0664-8
- Zaman M, Di H, Cameron K, Frampton C 1999: Gross nitrogen mineralization and nitrification rates and their relationships to enzyme activities and the soil microbial biomass in soils treated with dairy shed effluent and ammonium fertilizer at different water potentials. Biol. Ferti. Soils, 29, 178–186. doi:10.1007/s003740050542
- Zhao J, Wu X, Nie C, Wu T, Dai W, Liu H, Yang R 2012: Analysis of unculturable bacterial communities in tea orchard soils based on nested PCR-DGGE. World J. Microb. Biot., 28, 1967–1979. doi:10.1007/s11274-011-0999-5