ABSTRACT
Methane is one of the greenhouse gases emitted from paddy soil ecosystems and may induce global warming and climate change; therefore, mitigation options are urgently required to establish mitigation technology to reduce methane emission without affecting rice production. Methane is produced by a balance between oxidizing agents (such as iron) and reducing agents (easily decomposable soil organic matter), according to the so-called Takai theory. To evaluate options for mitigating methane production potential and to examine the applicability of the Takai theory in Southeast Asian paddy soils, 23 soil samples were collected from Thailand, Indonesia, Philippines, and Vietnam. These soil samples were anaerobically incubated to measure their methane production potential and examined to see whether their chemical properties, such as the ferrous, total iron, and organic matter contents, were correlated. We found a significant negative correlation between the methane production potential and the total iron content, and a positive correlation between the methane production potential and the hexose content, as an index for a soil’s easily decomposable organic matter content. The methane–C/CO2–C production ratio was also positively correlated with the mineralizable nitrogen/ferrous contents ratio, which indicated that the Takai theory, established for Japanese paddy soils, is also useful in Southeast Asian paddy soils and that the soil’s iron content is important to estimate the methane production potential.
1. Introduction
Methane (CH4) is one of the greenhouse gases (GHGs) emitted from paddy soils and other ecosystems. Together with other GHGs, such as carbon dioxide (CO2) and nitrous oxide, it may induce global warming and climate change (Lal and Kimble Citation1995; IPCC Citation2001). Among anthropogenic GHGs, methane is estimated to account for 14% of the CO2-equivalent emissions; rice paddy emits 60 Mt C year−1, particularly from tropical Asia (IPCC Citation2007). Therefore, mitigation options are urgently required to reduce emission of these GHGs without reducing rice production. In paddy soil, the predominant process producing methane under flooded conditions is anaerobic decomposition (Takai Citation1970). It is therefore important that the soil properties controlling methane production in paddy soil are identified. Takai and Kamura (Citation1961) investigated the biochemical processes by which soil organic matter is microbially decomposed and which reduction reactions take place in submerged paddy soil; they established that methane production is determined by the balance between reducing (easily decomposable soil organic matter) and oxidizing (ferrous and manganese) agents, which has been called the Takai theory (Takai Citation1970). Asami and Takai (Citation1970) also showed that adding free iron oxide to submerged soil significantly reduces methane production. By using samples taken from long-term fertilizer experiments, Inubushi et al. (Citation1984) examined the relation between the reduction process and the decomposition of organic matter in seven types of Japanese paddy soils and found that the decomposition of soil organic matter was quantitatively and predominantly associated with iron reduction and methane production. Inubushi et al. (Citation1990) reported that relationship between methane production and free iron oxide contents in 13 Japanese paddy soils was significantly negative. Watanabe and Kimura (Citation1999) also investigated methane emission in pot experiments with eight Japanese soils and found a significant negative correlation between methane emission and quantity of amorphous Fe(III) in the soils.
One of the possible mitigation methods to reduce methane emissions from paddy fields is to apply iron-containing materials, such as slag. Some incubation studies (Yoshiba et al. Citation1996; Inubushi et al. Citation1997) and pot experiments (Furukawa and Inubushi Citation2002, Citation2004) were performed to apply slag materials to Japanese paddy soils; therefore, it was possible to reduce both the production and emission of methane. The extent to which methane production is suppressed by the addition of iron-containing material depends on the original level of iron content in the soil. Furukawa and Inubushi (Citation2004) examined the effect of applying slag from revolving furnaces on methane emission from two paddy soils with different iron levels; they found that methane emission could be suppressed by such application only in the soil with a low iron level. Few studies have investigated the relation between methane emissions and soil properties in Southeast Asia (a major rice-producing area); however, it is unclear whether methane emissions can be reduced by supplementing with iron-containing materials. Therefore, to evaluate methane production in Southeast Asian paddy fields and the feasibility of mitigation options, 23 soil samples were collected from Thailand, Indonesia, Philippines, and Vietnam; these soil samples were anaerobically incubated to measure their methane production potential. The correlation between chemical properties of the soils, such as iron and organic matter contents, was examined.
2. Materials and methods
2.1. Soil samples and incubation
shows the locations where the 23 soil samples were collected from typical paddy fields in Thailand, Indonesia, Philippines, and Vietnam. We collected four replicate soil samples from each site and incubated and/or analyzed them separately; the averages of the results are given, along with their standard deviations. Samples were air-dried and sieved (<2 mm), then 5 g of each sample with four replicates was incubated in 25-mL test tubes with 5 mL distilled water under flooded conditions and under a N2 gas headspace and sealed to keep them anaerobically at 30°C for 8 weeks.
Table 1. Soil sampling locations, and chemical properties.
2.2. Gas and soil chemical analyses
Soil pH (H2O) of the air-dried samples was determined using electrodes (Horiba D-52, Horiba, Kyoto, Japan). The total carbon (C) and nitrogen (N) contents were determined by the combustion method (CN Corder Yanaco MT-700, Yannco Technical Science, Tokyo, Japan). The hexose content of air-dried soil was determined by hot-water extraction and colorimetry (Lu et al. Citation1998). The total iron and free iron oxide contents of air-dried soils were determined by extraction with hydroxyl amine and dithionite, respectively, and determined by colorimetry (Asami and Kumada Citation1959; JSSSPN Citation1986).
At the end of the incubation, mineralizable N was extracted with KCl and determined by the nitroprusside method (Anderson and Ingram Citation1989; Carole and Scarigelli Citation1971), and the ferrous iron content was extracted with acetate buffer and determined by colorimetry (Kumada and Asami Citation1958). Methane and CO2 concentrations in the headspace were taken by syringe and determined with a gas chromatograph (GC 14B, Shimadzu Corporation, Kyoto, Japan) equipped with a flame ionization detector and a thermal conductivity detector, respectively (Inubushi et al. Citation1997). Dissolved methane and CO2 concentrations in the liquid phase were estimated according to Cheng et al. (Citation2005).
2.3. Statistical analysis
The mean comparison of the soil properties was performed at 5% level of probability using the least significant difference test. Correlation between the production of methane and CO2 and the soils’ chemical properties were examined by Pearson’s correlation analysis using the XLSTAT statistical software program at 5% or 1% levels of significance.
3. Results
3.1. Chemical properties of examined soils
The chemical properties of the examined soils () were a slightly acidic pH (average of 5.1; range 3.5–7.7) and relatively high C [13.2 g kg−1 d.s. (dry soil) on average; range, 0.1–46.7 g kg−1 d.s.] and N (1.2 g kg−1 d.s. on average; range 0.04–3.2 g kg−1 d.s.) contents, compared with paddy soils from 10 tropical Asian countries (Kyuma Citation1985). The reason is that some of our examined soils, such as the sample from Sungairungas, Indonesia, contained peaty materials, although some others, such as that from Soc Trang, Vietnam, were sandy soils. The mean hexose content was 681 mg kg−1 d.s. (approximately 5.2% of total C; range, 215–1808 mg kg−1 d.s.). The total iron (22.4 g kg−1 d.s. on average; range, 1.8–66.8 g kg−1 d.s.) and free-iron oxide (8.0 g kg−1 d.s. on average; range, 0.8–26.6 g kg−1 d.s.) were in the same range as reported by Kyuma (Citation1985).
3.2. Nitrogen mineralization, ferrous and gas productions during incubation
The mineralizable N contents after 8 weeks of anaerobic incubation varied widely in the range 62–326 mg/kg d.s., although on average it was 153 mg/kg d.s. (approximately 1.26% of the total N) (). Inubushi et al. (Citation1985) compared N mineralization in 13 Japanese paddy soils in long-term fertilizer experiments with four Philippine paddy soils; they found that the ratio of easily mineralizable N to total N was similar for both groups, i.e., 1.84 ± 1.03% and 1.36 ± 1.90% (average ± standard deviation) for the Japanese and Philippine soils, respectively.
The mean ferrous production was 3.66 g kg−1 d.s. (range, 0.5–11.1 g kg−1 d.s.; approximately 16.3% of the total iron and 45.8% of the free iron contents).
Methane production varied widely from almost 0 to 260 mg C/kg d.s. (). The ratio of methane production to total C was calculated as 0.92% on average. CO2 production also varied widely from almost 45 to 370 mg C kg−1 d.s., with an average ± standard deviation of 160 ± 98 mg C kg−1 d.s. The ratio of CO2 production to total C was calculated as 1.2% on average.
3.3. Correlation between CH4 and CO2 production and chemical properties of the soil
A significant positive correlation was found between methane production and hexose content, between CO2 production and hexose content, and between CO2 production and mineralizable N content, whereas methane production potential and soil total iron were negatively correlated (, .
Table 2. Correlation between the production of CH4 and CO2 and the soil’s chemical properties.
4. Discussion
Yagi and Minami (Citation1990) showed that the emission of methane from four Japanese paddy soils was positively correlated with the readily mineralizable C content, which was determined as the sum of CO2 and methane production during 28 days incubation at 25°C. Watanabe and Kimura (Citation1999) also arrived at a similar conclusion. Hexose is known as one of the easily decomposable C components in soil, suggesting that hexose can be used as a possible index for CO2 and methane production, as indicated in . However, the ratio of CO2 and methane as products of soil C decomposition is still unclear.
Using 16 Japanese paddy soils in long-term fertilizer experiments, Inubushi et al. (Citation1984) examined the relation between reduction process and organic matter decomposition at 12 weeks of incubation; the decomposition of soil organic matter was quantitatively associated with iron reduction and methane production. Among the reduction reactions in paddy soil, iron reduction accounted for 66–84% of the decomposition of soil organic matter in the early period of incubation, as indicated in the reaction below.
Based on this formula, it is assumed that methane production can be estimated by the balance between easily decomposable organic matter (reducing agents) and iron (oxidizing agent) contents, as described by the Takai theory. Mineralizable N (Min-N) is a well-known index for the easily decomposable organic matter in paddy soils. A positive correlation was observed between methane production potentials (Y) and (Min-N) minus (1/4*total Fe) (X) (Fig. S).
Cheng et al. (Citation2007) reported that methane production (PCH4) in nine types of rice soils in Japan incubated for 16 weeks could be simplified to the balance of available N (Nav) and total iron as PCH4 = 1.59*Nav – 2.46*(total Fe)/1000. Such a formula can also be described for Southeast Asian paddy soils, taking the linear regression line in Fig. S as
when two outliers, the Suphamburi and Maligaya soils, are excluded. Only these two soils produced ferrous iron at >10 g kg−1. In addition, they may contain more fresh organic matter than other soils in which methane production could not be estimated from the total iron and N mineralization index because N was immobilized with fresh organic matter. If preincubation is conducted before the incubation, such exceptional results may be avoided. The factors in the above two formulae were different for Japanese (Cheng et al. Citation2007) and Southeast Asian soils (this study), which may be partly due to the different methods used to estimate the parameters in the formulae.
The ratio of methane–C/CO2–C production (Y) varied from almost 0 to 0.8; it was also positively correlated with the ratio of min-N content/Fe content (X) (), suggesting that, like the Takai theory, it may be useful for estimating soil iron content as an important index to gauge the reduction of methane production in Southeast Asian paddy soils. Tsutsuki and Ponnamperuma (Citation1987) investigated methane and CO2 production in three anaerobically incubated Philippine paddy soils at 20°C and 35°C for 8 weeks with or without organic matter amendments and found that the ratio of methane/CO2 production varied from 0.002 to 0.78, which was narrower than that obtained in our study. However, the ratio became wider at higher temperatures, even without changes in the organic matter. They also showed that the ratio increased upon enrichment with organic matter, i.e., to 0.06–1.1, after addition of rice straw and green manure. Wassmann et al. (Citation1998) investigated the methane production capacity in 11 Philippine paddy soils anaerobically incubated at 25°C, 30°C, and 35°C for 8 weeks, and found that methane production was significantly correlated with organic C and N contents, but not with active iron content. They also reported that supplementing with acetate strongly enhanced methane production; the explanation for this observation is that acetate is one of the important substrates for methane production (Takai Citation1970). Amended organic matter and also plant debris, such as root exudates, can be a substrate for the combined production of methane and hydrogen; therefore, adding these can increase the methane/CO2 ratio.
Figure 3. Relation between Min-N/Fe (X) and CH–C/CO2–C (Y). The linear regression line was calculated with all soils.
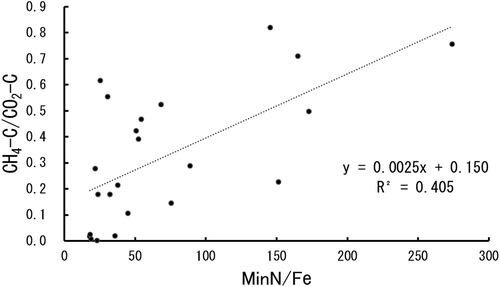
The suppression of methane production by adding iron-containing material depends on the original level of the soil’s iron content. Furukawa and Inubushi (Citation2004) examined two paddy soils with different iron levels, 18.5 and 28.5 g Fe kg−1, and the effect of adding slag from revolving furnaces on methane emission was found to be a decrease of 25–50% only in soils with a low iron level. When comparing these levels with those found in the Southeast Asian paddy soils examined in this study (), about half of the paddy soils examined can be expected to show an effect of addition of iron-containing material on reducing methane emission, although differences in climate and ecosystem should be considered. Further study is needed to find appropriate target paddy soils with low iron contents and the appropriate application rate of iron-containing materials to achieve reduction of methane emission as well as to avoid side effects on other reactions such as iron toxicity in the paddy soil–rice ecosystem.
Acknowledgments
This international study was conducted as joint research by Chiba University, Nippon Steel & Sumitomo Metal Corporation, and Sumitomo Corporation. We acknowledge the following scientists and the colleagues in their universities/institutes for helping with our soil sampling and classification: Suphachai Amkha (Kasetsart University, Thailand), Amnat Chidthaisong (King Mongkut University, Thailand), Patcharee Saenjian (Khon Kaen University, Thailand), Abdul Hadi (Lambung Mangkurat University, Indonesia), Iswandi Anas (Bogor Agricultural University, Indonesia), Prihasto Setyanto (Indonesian Agency for Agricultural Research and Development Research Station for Agricultural Environment Preservation, Jakenan, Indonesia), Asis Constancio (Phil Rice, Philippines), Pham Quang Ha (Vietnam Academy of Agricultural Sciences, Vietnam), Nguyen Huu Thanh (Hanoi University of Agriculture, Vietnam), Phan Thi Cong (Institute of Agricultural Science for Southern Vietnam, Vietnam), and Tran Kim Tinh (Can Tho University, Vietnam).
References
- Anderson JM, Ingram JSI 1989: Colorimetric determination of ammonium. In A Handbook of Methods, Tropical Soil Biology and Fertility, ed. ISSS, 42–43. CAB International, Wallingford.
- Asami T, Kumada K 1959: A new method for determining free iron in paddy soils. Soil Sci. Plant Nutr., 5, 141–146. 10.1080/00380768.1959.10430907
- Asami T, Takai Y 1970: Behavior of free iron oxide in paddy soil (Part 4). J. Sci. Soil Manure Jpn., 41, 48–55. (in Japanese).
- Carole RS, Scarigelli FP 1971: Colorimetric determination of nitrate after hydrazine reduction to nitrite. Microchem. J., 16, 657–672. 10.1016/0026-265X(71)90059-2
- Cheng W, Yagi K, Akiyama H, Nichimura S, Sudo S, Fumoto T, Hasegawa T 2007: An empirical model of soil chemical properties that regurate methane production in Japanese rice paddy soil. J. Environ. Qual., 36, 1920–1925. 10.2134/jeq2007.0201
- Cheng W, Yagi K, Sakai H, Xu H, Kobayashi K 2005: Changes in concentration and d13C value of dissolved CH4, CO2 and organic carbon in rice paddies under ambient and elevated concentrations of atmospheric CO2. Organic Geochem., 36, 813–823. 10.1016/j.orggeochem.2005.01.009
- Furukawa Y, Inubushi K 2002: Feasible suppression technique of methane emission from paddy soil by iron amendment. Nutr. Cycl. Agroecosys., 64, 193–201. 10.1023/A:1021150831735
- Furukawa Y, Inubushi K 2004: Effect of application of iron materials on methane and nitrous oxide emissions from two types of paddy soils. Soil Sci. Plant Nutr., 50, 917–924. 10.1080/00380768.2004.10408554
- Inubushi K, Hori K, Matsumoto S, Wada H 1997: Anaerobic decomposition of organic carbon in paddy soil in relation to methane emission to the atmosphere. Wat. Sci. Tech., 36. 523–530.
- Inubushi K, Umebayashi M, Wada H 1990: IUSS Transaction, II-249-254.Japanese Society of Soil Science and Plant Nutrition, Kyoto, Japan.
- Inubushi K, Wada H, Takai Y 1984: Easily decomposable organic matter in paddy soil, IV. Relationship between reduction process and organic matter decomposition. Soil Sci. Plant Nutr, 30, 189–198. 10.1080/00380768.1984.10434682
- Inubushi K, Wada H, Takai Y 1985: Easily decomposable organic matter in paddy soil, VI. Kinetics of nitrogen mineralizaition in submerged soils. Soil Sci. Plant Nutr, 31, 563–572. 10.1080/00380768.1985.10557464
- IPCC 2001: Climate Change: the Scientific Basis, Cambridge University Press, Cambridge.
- IPCC 2007: Synthesis Report, Vol. 31. 36, 503. Cambridge University Press, Cambridge.
- JSSSPN 1986: Methods of Soil Nutrient Analysis, Yokendo, Tokyo, Japan. (in Japanese).
- Kumada K, Asami T 1958: A new method for determining ferrous iron in paddy soils. Soil Plant Food, 3, 187–193. 10.1080/00380768.1957.10431920
- Kyuma K 1985: Fundamental Characteristics of Wetland Soils, Proc, Eds. Watanabe I and De Datta SK, pp. 191–206. Wetland Soils Workshop, IRRI.
- Lal R, Kimble J 1995: Soils and Global Change, Advances in Soil Science, CRC Press, Boca Raton, FL.
- Lu G, Sakagami K, Tanaka H, Hamada R 1998: Role of soil organic matter in stabilization of water-stable aggregates in soils under different types of land use. Soil Sci. Plant Nutr., 44, 147–155. 10.1080/00380768.1998.10414435
- Takai Y 1970: The mechanism of methane fermentation in flooded paddy soil. Soil Sci. Plant Nutr., 16, 313–316. 10.1080/00380768.1970.10433371
- Takai Y, Kamura T 1961: Dynamics of microorganisms in paddy soil. Kagaku, 31, 618–624. (in Japanese).
- Tsutsuki K, Ponnamperuma FN 1987: Behaviour of anaerobic decomposition products in submerged soils. Soil Sci Plant Nutr., 33, 13–33. 10.1080/00380768.1987.10557549
- Wassmann R, Neue HU, Bueno C, Lantin RS, Alberto MCR, Buendia LV, Bronson K, Papen H, Rennenberg H 1998: Methane production capacities of different rice soils derived from inherent and exogenous substrate. Plant Soil, 203, 227–237. 10.1023/A:1004357411814
- Watanabe A, Kimura M 1999: Influence of chemical properties of soils on methane emission from rice paddies. Commun. Soil Sci. Plant Anal., 30, 2449–2463. 10.1080/00103629909370386
- Yagi K, Minami K 1990: Effect of organic matter application on methane emission from some Japanese paddy fields. Soil Sci Plant Nutr., 36, 599–610. 10.1080/00380768.1990.10416797
- Yoshiba M, Morimura T, Aso S, Takenaga H 1996: Methane production and control in submerged soil applied with Mn4+, Fe3+ and SO42- rich materials. Jpn, J. Soil Sci. Plant Nutr, 67, 362–370. (in Japanese).