ABSTRACT
A hydrotalcite-like compound for reducing the leaching of nitrogen from farmland was prepared via a new synthesis procedure, and its chemical structure and anion exchange characteristics were described. The advantage of this new synthesis method is that it is a simple, inexpensive process that involves the mixing of only two types of materials, followed by a hydrothermal reaction that does not require pH or gas control. The materials for synthesis are MgO and FeCl3. Chemical analysis, X-ray diffraction, and scanning electron microscopy were used to confirm an Mg-Fe system hydrotalcite-like compound. The anion exchange capacity was 166 cmolc kg−1, which was determined using NO3− ion as an exchangeable anion. That capacity is equivalent to 23 g N kg−1. The nitrate adsorption isotherm of this compound approximately matched to the isotherm that was predicted by the Langmuir formula. The anion exchange selectivity coefficients of the compound were in the following order: NO3− > Cl− > SO42− > HPO42−. The developed compound is a prospective material for reducing leaching of NO3−N from farmland.
1. Introduction
Nitrate nitrogen (NO3−N) has been listed in the Environmental Quality Standards (EQSs) for groundwater since 1999 in Japan, and 10 mg dm−3 is the critical concentration from the standpoint of human health. Leaching of NO3−N is known to be more common than the leaching of ammonium nitrogen because both NO3−N and soil are negatively charged. Reducing the leaching of NO3−N from soil is an important factor for environmental protection. One of the main origins of NO3−N in groundwater might be applied fertilizers (Nakanishi et al. Citation2001; Nonaka and Kamura Citation1995; Nonaka et al. Citation1996). To reduce losses of NO3−N, researchers have investigated controlled-release fertilizer, partial fertilization (Togashi and Yamazaki Citation2000), and organic materials (Ito and Togashi Citation2002; Ito and Togashi Citation2010). Results have shown that the recovery rate of fertilizer N by crops increased and the losses of applied NO3−N were reduced. However, according to the results of the FY 2015 Water Quality Survey of Ground-water, 2.9% of the surveyed wells exceed the EQS for NO3−N or NO2−N (Ministry of Environment Citation2016). The results of this survey suggest that more effective leaching control technology is required to reduce groundwater pollution on sandy soil, particularly soil with high water percolation rate. Shimada and Takahashi (Citation1979) reported that mixing of an ion exchange resin with soil resulted in lower leaching of NO3−N than in untreated soil. However, ion exchange resins are too expensive for use in agricultural production. A low-cost material is needed.
NO3−N adsorption by biochar (BC) produced from wood-based biomass at 800°C was significantly higher than in BC produced from non-wood-based biomass at 800°C and can be adequate as a soil amendment material (Kameyama et al. Citation2016). However, the adsorption capacity was not high, even for BC with the highest adsorption ability. Mori and Ono (Citation1995) reported that charcoal (wood-based BC) could not adsorb NO3−N, but charcoal treated with ferric chloride (FeCl3) solution adsorbed NO3−N due to the positive charge of the ferric ion. Ito and Yamazaki (Citation2008) reported the effect of defatted rice bran carbide, which was treated with FeCl3 solution to reduce leaching of N from the sandy field. In their test group, the material was supplied with 3 Mg ha−1, and leaching of N was reduced to 2 kg ha−1. The amount of reduced N reaching was not satisfactory, instead of the large amount of application of the soil amendment material of 3 Mg ha−1. Therefore, the author tried to develop a new soil amendment having much larger adsorption capacity in the present study. The material, which has a larger adsorption capacity, was required to reduce the leaching of N.
Hydrotalcite is a clay mineral with a high anion exchange capacity (AEC). A reduction in NO3−N leaching is expected when this clay mineral is applied to soil. Hydrotalcite is a layered double hydroxide (LDH), and its formula is represented as M2+xM3+y(OH)2x+3y−nz (An−)z mH2O, where M2+ and M3+ are divalent and trivalent cations, respectively, and An− represents n-valent anions (Miyata and Kumura Citation1973). Many hydrotalcite-like compounds have been used in industrial or medical applications. The trivalent metal of LDH is typically aluminum (Miyata Citation1983), but aluminum compounds are not desirable for agricultural use in Japan because the crystal structure of hydrotalcite-like compounds is stable under neutral- to high-pH conditions but will dissolve under strongly acidic conditions (Hibino Citation2006); additionally, most upland soils in Japan are acidic (Takahashi et al. Citation2013). Hydrotalcite has well-known isomorphous and polytypic compounds. The Fe3+ derivative of hydrotalcite is known as pyroaurite or iowaite. Pyroaurite has been reported to exhibit the same NO3−N adsorption ability as hydrotalcite with Al3+ as the trivalent metal (Wuxian et al. Citation1995; Wuxian et al. Citation1996). Yamamoto (Citation2006) studied the utility of the Mg–Fe LDH system and reported that this LDH exhibits a high NO3–N adsorption ability. However, the influence of coexisting anions (i.e., phosphate and sulfate anions), which have been extensively applied as a soil conditioner or fertilizer on the adsorption of NO3−N, was not considered. In addition, the application of large amounts of LDH has been observed to increase soil pH and inhibit crop growth (Yamamoto Citation2006).
Most types of LDH are prepared using co-precipitation and hydrothermal methods. For example, LDH was prepared from a mixed solution of divalent and trivalent metals by adjusting the pH from 9 to 11 and treating the solution in an autoclave at 150°C for 3 d; the autoclave was filled with N2 gas beforehand. The resulting precipitate was subsequently washed with deionized and decarbonated water, dried, and ground to a powder (Wuxian et al. Citation1995). These procedures required pH adjustment of the solution, more than three different materials were needed, and the pH of the wastewater was high. Thus, this procedure for preparing LDH is expensive and complex, giving rise to pollution problems. Therefore, an easier procedure for producing LDH for agriculture is needed. In response, I developed a new procedure to prepare hydrotalcite-like LDH under easier, less expensive synthesis conditions than the conventional method. I characterized the new product, including its NO3−N adsorption ability and selective adsorbing capacity among NO3−, PO43−, SO42−, and Cl−.
2. Materials and methods
2.1. Sample preparation
LDH was prepared by mixing an MgO suspension and a FeCl3 solution followed by a hydrothermal method; alkaline materials (e.g., sodium hydroxide solution or aqueous ammonia) were not required. The samples were synthesized via reaction of 0.1 dm3 of 0.5, 1.0, 1.5, or 2.0 mol dm−3 FeCl3 (guaranteed reagent; Wako Pure Chemical Industries, Ltd., Osaka, Japan) solution with a 0.1 dm3 of 8 mol dm−3 MgO (heavy; Wako Pure Chemical Industries, Ltd, Osaka, Japan) suspension. Samples are referred as PA0.5, PA1.0, PA1.5, or PA2.0, respectively. The FeCl3 solution was added to a stirring suspension of MgO, and the pH of the solution was not adjusted. The precipitate settled via stirring for 30 s of the reactant mixture. Next, 0.2 dm3 of distilled water was added to the reactant to form a suspension. The reactant in the autoclave (SP-51; Yamato Scientific Co., Ltd., Tokyo Japan) was heated at 120°C for 2 h under autogenous pressure. The precipitate was collected by decantation, dried at 150°C for 1 d and subsequently washed with distilled water. The products were dried at 200°C for 1 d and subsequently ground into a powder.
2.2. Nitrate adsorption capacity of prepared samples
Five hundred milligrams of samples (i.e., PA0.5−PA2.0) were placed in plastic centrifuge tubes, and 25 × 10–3 dm3 of a 16 mmol dm−3 Ca(NO3)2 solution was added. The tubes were shaken for 1 h on a reciprocal shaker (SA-31; Yamato Scientific Co., Ltd., Osaka, Japan) and centrifuged at 1670 g for 3 min. Supernatants were diluted to 50-fold and filtered through a disk filter (PP Syringe Filter 0.45-μm, Membrane Solutions Co., Ltd., TX., USA). The amount of NO3− in the solution was determined by ion chromatography (SCL-10Asp; Shimadzu Co., Kyoto, Japan). The amount of NO3− adsorbed with samples was calculated according to the following equation:
where QNO3 is the adsorbed NO3 (cmol kg−1), C0 and Ceq are the initial and equilibrium concentration of NO3, respectively (mmol dm−3), V1 is the volume of extraction (dm3), and W1 is the mass of PA (kg).
2.3. Analysis of prepared samples
The Mg and Fe contents of PA1.5 were determined by atomic absorption spectrometry (SpectrAA 220FS; Varian Australia Pty Ltd., Australia) and Cl− content was determined by ion chromatography after the PA1.5 was dissolved in 1 mol dm−3 HNO3. The structure of PA1.5 was analyzed by X-ray powder diffraction (XRD), and the pattern was recorded on a diffractometer using CuKα radiation (λ = 1.54056 Å) with a Bragg angle of 2–70° (2θ) and steps of 0.02°counting 0.6 s/step (MiniFlex; Rigaku Co., Tokyo, Japan). PA1.5 was coated with a thin layer of evaporated carbon, and images were obtained using a HITACHI SU8000 scanning electron microscope (SEM) at a 15-kV accelerating voltage.
2.3.1. Nitrate adsorption isotherms for PA1.5
Solutions with the initial NO3− concentrations of 0, 1.6, 3.2, 8.0, or 16 mmol dm−3 were prepared using Ca(NO3)2. One gram of PA1.5 was suspended with 25 × 10−3 dm3 of each Ca(NO3)2 solution in plastic centrifuge tubes; after being shaken for 1 h, each tube was allowed to stand for 12 h. Supernatant was diluted 50-fold and filtered through a disk filter. The amount of NO3− and Cl− in solution was determined by the aforementioned ion chromatography. The amounts of NO3− adsorbed with PA1.5 were calculated using Equation (1). The NO3− exchange isotherm of PA1.5 was adapted to the Langmuir formula using the least squares method.
The amount of desorbed Cl− was calculated according to the following equation:
where QCl is the concentration of Cl− (mmol kg−1), Ceq is the equilibrium concentration of Cl− (mmol dm−3), C0 is the concentration of Cl− (mmol dm−3) in the equilibrium solution not including NO3−, V2 is the volume of extraction (dm3), and W2 is the mass of PA1.5 (kg).
2.4. Determination of the AEC for PA1.5
AEC was determined according to the method of Nakahara and Wada (Citation1993). Although Nakahara and Wada measured AEC with Cl− as an exchangeable anion, in this study, AEC was measured using NO3− as an exchangeable anion because the adsorptive target ion is NO3−, and the procedure is as follows. The PA1.5 (0.5 × 10−3 kg) was suspended as a 25 × 10−3 dm3 of 1 mol dm−3 KNO3 solution in a plastic centrifuge tube that was weighed in advance. The suspension was shaken for an hour in the abovementioned shaker and left to stand overnight with occasional stirring. The supernatant was disposed after centrifugation by 1670 g, 3 min. Next, the PA1.5 that remained in the tube was washed five times with 0.02 mol dm−3 KNO3 solution. The content of NO3− in the equilibrium solution was measured with supernatant after the fifth washing. Next, the PA1.5 was washed five times with a 1 mol dm−3 KCl solution, and supernatants were collected to measure the NO3− content. AEC was calculated by the following equation:
where AEC is the anion exchange capacity (cmolc kg−1), nex is the amount of NO3− in the extraction (mmol), meq is the mass molar concentration of the equilibrium solution (mmol kg−1), Wresidual is the mass of the equilibrium solution that was residual in the tube (kg), and W3 is the mass of PA1.5 (kg).
2.5. Evaluation of anion exchange selectivity of PA1.5
The following anions in the soil due to fertilization were used as target anions: nitrate, phosphate, and sulfate ions. The anion solutions were prepared using NaNO3 (extra pure reagent: Kanto Chemical Co. Inc., Tokyo, Japan), NaH2PO4 (guaranteed reagent; Wako Pure Chemical Industries, Ltd., Osaka, Japan), and Na2SO4 (guaranteed reagent; Wako Pure Chemical Industries, Ltd., Osaka, Japan). Then, 0.1 × 10−3 kg of PA1.5 was suspended in 25 × 10−3 dm3 of anion solution. Total negative charge of anion contained in the test solution was prepared so as not to exceed the amount that 0.1 × 10−3 kg of PA1.5 could adsorb. The initial concentrations of each anion and the pH of each solution were adjusted to 3 mmol dm−3 and 7.0, respectively. However, a Cl− solution was not prepared because PA1.5 already contains Cl− intercalated into the layered structure. The concentration and pH for the three anions mixed solution was adjusted to the same of single anion solution (i.e., each anion concentration and pH was 3 mmol dm−3 and 7.0, respectively). For this reason, the total negative charge of anions in the three anions mixed solution was 0.2 meq above the amount that 0.1 × 10−3 kg of PA1.5 could adsorb.
Because it is assumed PA1.5 will be used for agricultural purposes, a competitive adsorption experiment on imitative soil solution was conducted. An imitative soil solution was prepared including Cl−, NO3−, PO43−, and SO42− to 2 mmol dm−3, 10 mmol dm−3, 1 mmol dm−3, and 2 mmol dm−3, respectively, based on the author’s experience in the chemical analysis of dune fields. The amount of PA1.5 application was assumed to be 1 Mg ha−1, and the amount of soil solution included in 1 ha of dune field was estimated at 250 Mg (assuming cultivated soil depth is 20 cm, bulk density is 1.3 g cm−3, and water content is 9–10%). Therefore, 0.1 × 10−3 kg of PA1.5 was suspended in 25 × 10−3 dm3 of imitative soil solution. The negative charge of ions contained in the imitative soil solution was 0.28 meq above the amount that 0.1 × 10−3 kg of PA1.5 could adsorb. The anion exchange selectivity of the aforementioned anions was determined as follows. PA1.5 was suspended in solutions containing the aforementioned anions and shaken for 1 h. The supernatant was collected by decanting after centrifugation (1670 g, 3 min) to determine the anion content in the equilibrium solution. The concentration of each anion in the equilibrium solution was measured using ion chromatography. The adsorption of each anion was calculated using the following equation:
where Q is the adsorbed anion (cmol kg−1), C0 and Ceq are the initial and equilibrium concentration of anion, respectively (mmol dm−3), V4 is the volume of extraction (dm3), and W4 is the mass of PA1.5 (kg).
The anion exchange selectivity coefficients for each anion were related to Cl− (KGT) (Gaines and Thomas Citation1953) and the distribution coefficients (Kd dm3 kg−1) were calculated using the following equation:
Fundamentally, the ion exchange selectivity coefficient is calculated using the ion activity in solution. The activity can be computed by multiplying the molar concentration by activity coefficient. In this study, the selectivity coefficient was computed using the molar concentration of solute ions instead of activities, because the activity coefficients will approach 1 in dilute solutions.
The selectivity coefficient (KGT) defined using the charge fraction is known as Gains–Thomas selectivity coefficient (Wada Citation1987). EA and ECl are charge fractions of adsorbed anion (mol kg−1) and chloride (mol kg−1), and [AZ−] and [Cl−] are concentrations in the solution phase (mol dm−3). The anion adsorption selectivity was evaluated using log KGT and Kd. The experiments were performed at ambient temperature (25°C).
3. Results and discussion
3.1. Anion adsorption capacity of prepared samples
The relationship between the Fe/Mg molar ratio of reactants and NO3− adsorption ability of the samples is shown in . PA1.0 and PA1.5 exhibited the highest NO3− adsorption ability among the investigated samples. The molar ratio of Fe/Mg in reactants for the synthesis of samples with high nitrate ion adsorption ability was thus surmised to be 0.13–0.19.
3.2. Determination of the structure of PA1.5
The chemical composition of PA1.5 is shown in . The molar ratio of cations (Fe/Mg) of the product was 0.23, and chloride ion was included in the compound. However, the mole of Cl− in the unit mass of PA1.5 was smaller than for substituted Fe which is the cause of the positive charge on PA1.5. It was deduced that HCO3− and CO32− exist at the exchange site, because PA1.5 was synthesized using distilled water, and the procedure was performed in an air atmosphere with an alkaline reactant.
Table 1. Chemical composition of PA1.5.
The XRD data for PA1.5 is shown in . The data in this work are very similar to that for iowaite (a mineral of the hydrotalcite group) in previous reports (Kohls and Rodda Citation1967; Braithwaite et al. Citation1994). The lattice constants of PA1.5 were determined to be approximately a = 311 pm and c = 2407 pm and are close to those for natural iowaite, a = 311.9 pm and c = 2425 pm (Cavani et al. Citation1991). The basal spacing of the present compound was determined to be d(003) = 802 pm. The results of XRD indicated that PA1.5 is a hydrotalcite-like compound similar to iowaite. The SEM micrograph in shows the morphology of the PA1.5 surface, which consists of an agglomerate of flaky crystals.
Table 2. X-ray powder diffraction data.
3.3. Adsorption characteristics of PA1.5
3.3.1. Adsorption of NO3−N and AEC
The ion exchange isotherm of NO3− at 25°C is shown in . The amount of adsorbed NO3− increased with increasing equilibrium concentration of NO3− in the aqueous solution. The positive charge of the soil (e.g., andosol) is the variable charge reduced by proton adsorption at low-pH condition (Nakahara and Wada Citation1993; Wada Citation1988). The positive charge of the PA1.5 was caused by Fe3+ which was substituted for Mg2+ in the brucite sheet, and the NO3− adsorptive capacity of PA1.5 was observed under a high-pH condition (). The hydrotalcite-like compound has positive charge and electrostatic balance is maintained by anion intercalation between the layers (Hibino Citation2006). The relationship between the amount of NO3− adsorption and the amount of Cl− desorbed into equilibrium solutions is shown in . A high positive correlation was observed between the amount of NO3− adsorption and the amount of Cl− desorption. It was surmised that the adsorption of NO3− occurs via ionic exchange with intercalated or adsorbed Cl−.
Table 3. The pH value at equilibrium and AEC of PA1.5.
Figure 3. NO3– exchange isotherm of the PA1.5. The approximated curve was drawn by the formula of Langmuir.
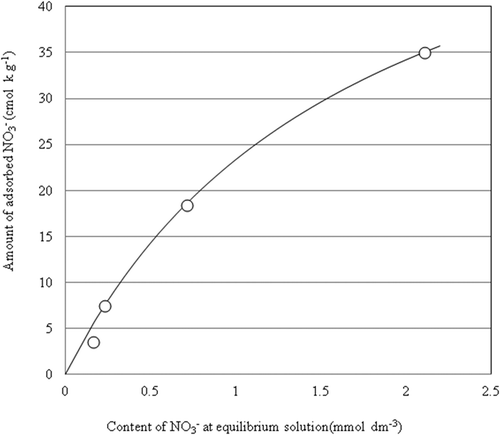
Figure 4. Relationship between NO3– adsorption and the Cl–desorption. Simple regression analysis was done by Excel software.
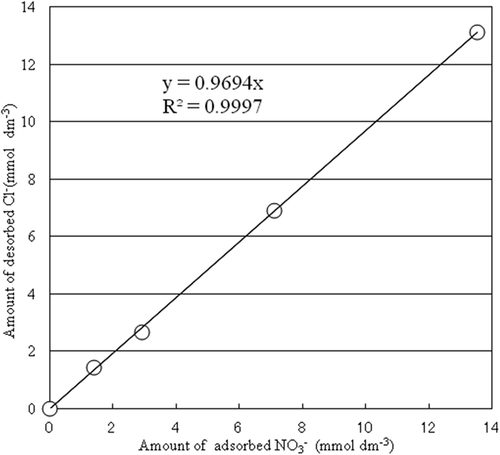
The AEC of PA1.5 was 166 cmolc kg−1 (). This value of AEC is equivalent to approximately 23 g of N per kilogram of PA1.5. This compound exhibits NO3− exchange capacity approximately equal to that of a pyroaurite-like compound synthesized using a conventional method (i.e., 170–190 cmolc kg−1) (Wuxian et al. Citation1995; Wuxian et al. Citation1996).
3.3.2. Adsorption selectivity of anions
shows the adsorbed anion amounts, distribution coefficient (Kd), and anion exchange selectivity coefficient for PA1.5 for anions in the solution containing one anion. The pH of the solution suggests that phosphate ions in the solution were divalent (HPO42−). The order of adsorbed anion amounts was NO3− > SO42− > HPO42−. The distribution coefficients (Kd) for PA1.5 for anions was also in the order NO3− > SO42− > HPO42−. The logarithm of the anion exchange selectivity coefficient (KGT) of NO3− related to Cl− was above 0. However, coefficients of HPO42− and SO42− were below 0, and the coefficient for HPO42− was lower than for SO42−. Therefore, it was inferred that the order of anion exchange selectivity on PA1.5 was as follows: NO3− > Cl− > SO42− > HPO42−.
Table 4. The anion adsorption, selectivity coefficient (KGT), and the distribution coefficient (Kd) for each anion on the equilibrium solution containing one kind of anion.
In the case of the solution with three anions, the amount of adsorbed NO3− was also greater than for SO42− and HPO42− (). Ion exchange selectivity usually increases when valence is high. These conflicting results between the valence of anions and the anion exchange selectivity may be explained by the interlayer space of PA1.5 and the ionic radii of anions. The distance of the interlayer of PA1.5 was 325.3 pm, which was calculated by subtracting the thickness of the brucite layer (476.9 pm: NipponKagakukai Citation1966) from the thickness (802.2 pm) of the unit layer. PA1.5 was prepared as an LDH with intercalated Cl− (ionic radius is 167 pm: Saito Citation2006), and the interlayer space of dried PA1.5 was approximately twice the ionic radius of the intercalated anion (Hibino Citation2006). The ionic radius of NO3− is 165 pm (Saito Citation2006) with a planar structure that comprised oxygen bound to nitrogen. In contrast, sulfate SO42− exhibits a tetrahedral ion structure, and its ionic radius is 230 pm (Saito Citation2006). These ionic radii indicate that NO3− can prioritize exchange with Cl− over SO42−.
Table 5. The anion adsorption and distribution coefficient (Kd) for each anion on the equilibrium solution containing three kinds of anion.
shows that the amounts of adsorbed NO3− and SO42− and Kd were lower than for the solution containing a single anion species. It was suggested that there was competition for adsorption among coexisting ions. However, the adsorbed amount and Kd for HPO42− did not differ from that in the solution with a single anion ( and ). In addition, the amount of Cl− transferred from the solid phase to solution phase in HPO42− solution was significantly smaller than for other solutions (). It was surmised that HPO42− was adsorbed via other processes in addition to ionic exchange. Elution of Mg from Mg-Fe-Al-Cl hydrotalcite was reported at the initial pH of 7.0 or below, and phosphate ion uptake by coagulative precipitation was reported (Kuwabara et al. Citation2007). The present experiment was conducted with a solution adjusted to pH 7.0 from initial solution, and therefore it was surmised that phosphate uptake occurred via coagulative precipitation. The pH suggests that the precipitate was MgHPO4. Moreover, the surface complexation of phosphate with FeOH or MgOH group at the edge of PA1.5 can also be a phosphate removal process. Even if phosphate was taken up by coagulative precipitation or surface complexation by surface hydroxyl groups, it cannot be concluded that phosphate uptake by plant would be hindered. Because MgHPO4 dissolves in a weak acid, coagulative precipitated phosphate is regarded as an available form. Moreover, despite complexation by surface hydroxyl groups, some plants can take up Fe- or Al-bound phosphorus by organic acids in root exudates (Otani and Ae Citation1996).
The competitive adsorption of anions by PA1.5 on an imitative soil solution is shown in ; similar to the aforementioned competitive adsorption experiment, more NO3− was adsorbed compared with SO42−. The most HPO42− was adsorbed, and Cl− was not adsorbed.
Table 6. The competitive adsorption for each anion in the imitative soil solution.
The anion adsorption characteristics of PA1.5 suggest that leaching of NO3−N will be reduced when PA1.5 is applied to an agricultural field.
4. Conclusions
An Mg-Fe system hydrotalcite-like compound (PA1.5) was synthesized using a new method, and its structure and anion adsorption characteristics were determined. The results were as follows.
PA1.5 was synthesized using an easy, cost-effective method that simply involves mixing of a 8 mol dm−3 MgO suspension and a 1.5 mol dm−3 FeCl3 solution, followed by a hydrothermal reaction without pH or gas control.
PA1.5 is a hydrotalcite similar to iowaite; the layered structure was confirmed by XRD.
PA1.5 has AEC with an AEC of 166 cmolc kg−1, equivalent to 23 g for N per kilogram of PA1.5.
The adsorption of NO3− occurs via ionic exchange with intercalated or adsorbed Cl−.
The anion exchange selectivity coefficient (KGT) of PA1.5 was in the order NO3− > Cl− > SO42− >HPO42−.
PA1.5 adsorbed HPO42− via other processes in addition to ionic exchange.
PA1.5 is expected to control the leaching of NO3−N from farmland.
Acknowledgments
The author is grateful to Dr Masami Nanzyo for analysis by powder XRD, SEM at Tohoku University Graduate School of Agricultural Science, and Ho Ando for his valuable advice on this paper.
References
- Braithwaite RSW, Dunn PJ, Pritchard RG, Paar WH 1994: Iowaite, a re-investigation. Mineral. Mag., 58, 79–85.
- Cavani F, Trifiro F, Vaccari A 1991: Hydrotalcite-type anionic clays: preparation, properties and applications. Catal. Today, 11, 173–301.
- Gaines GL, Thomas HC 1953: Adsorption studies on clay minerals. II. A formulation of the thermodynamics of exchange adsorption. J. Chem. Phys., 21, 714–718.
- Hibino T 2006: Synthesis and applications of hydrotalcites. J. Clay Sci. Soc. Jpn., 45, 102–109 in Japanese
- Ito M, Togashi M 2002: Mineralization and effect of continued application of organic materials on sand dune field. Tohoku Agric. Res., 55, 211–212 in Japanese
- Ito M, Togashi M 2010: Effect of successive application of organic matter on melon cultivation in sand field. Jpn. J. Sci. Plant Nutr., 81, 387–390 in Japanese
- Ito M, Yamazaki N 2008: Effect of a carbide of defatted rice bran treated with iron(III) chloride on retention of nitrate nitrogen, growth of melon and radish and leaching of nitrate nitrogen in sand dune area. Jpn. J. Sci. Plant Nutr., 79, 155–161 in Japanese with English summary
- Kagakukai N 1966: Kagakubinran, Kisohen, 2, pp. 1262. Maruzen, Tokyo in Japanese
- Kameyama K, Miyamoto T, Iwata Y, Shiono T 2016: Influence of feedstock and pyrolysis temperature on the nitrate adsorption of biochar. J. Soil Sci. Plant Nutr., 62, 180–184.
- Kohls DW, Rodda JL 1967: Iowaite, a new hydrous magnesium hydroxide-ferric oxychloride from the precambrian of Iowa. Am. Mineralogist, 52, 1261–1271.
- Kuwabara T, Kimura H, Sunayama S, Kawamoto A, Oshima H, Sato T 2007: Removal characteristics of phosphate and nitrate ions with an Mg-Fe-Al-Cl form hydrotalcite. J. Soc. Inorg. Mater .Jpn., 14, 17–25.
- Ministry of Environment 2016: Annual Report on the Environmental, the Sound Material-Cycle Society and the Biodiversity in Japan, 221–223 (in Japanese)
- Miyata S 1983: Anion exchange properties of hydortalcite-like compounds. Clays Clay Miner., 31, 305–311.
- Miyata S, Kumura T 1973: Synthesis of new hydrotalcite-like compounds and their physico-chemical properties. Chem. Lett., 8, 843–848
- Mori A, Ono S 1995: Appearance of the nitrate ion adsorptive ability in charcoal treated with iron(III) chloride solution. Jpn. J. Sci. Plant Nutr., 66, 415–417 in Japanese
- Nakahara O, Wada S-I 1993: Cl– adsorption on andisols from CaCl2 and MgCl2 solutions. Soil Sci. Plant Nutr., 39, 645–652.
- Nakanishi Y, Takahira K, Shimoji K 2001: Estimation of nitogen loading factors for groundwater by multiple regression analysis. Jpn. J. Sci. Plant Nutr., 72, 365–371 in Japanese with English summary
- Nonaka M, Abe R, Kamura T 1996: Nitrate movement through soil profile and groundwater pollution by nitrogen fertilizer in sand dune upland soil. Jpn. J. Sci. Plant Nutr., 67, 633–639 in Japanese with English summary
- Nonaka M, Kamura T 1995: Nitrate leaching and nitrogen balance in sand dune upland soil by Lysimeter. Jpn. J. Sci. Plant Nutr., 66, 372–380 in Japanese with English summary
- Otani T, Ae N 1996: Phosphorous (P) uptake mechanisms of crops grown in soils with low P status. I. Screening crops for efficient P uptake. Soil Sci. Plant Nutr., 42, 155–163.
- Saito G 2006: Yuuki Bussei Kagaku No Kiso, pp. 64–66. Kagaku-dojin Publishing Co., Kyoto in Japanese
- Shimada N, Takahashi N 1979: Research about leaching control of salts with an anion exchange resin. Jpn. J. Sci. Plant Nutr., 50, 5–9 in Japanese
- Takahashi M, Komatsuzaki M, Hasegawa M, Hashimoto M, Kaneko N 2013: Chemical characteristics of forest soils and comparison of their characteristics with agricultural soils according to the criteria for promoting soil fertility: forest soil as a reference for conservation-oriented agriculture. Jpn. J. Soil. Sci. Plant Nutr., 84, 275–284 in Japanese with English summary
- Togashi M, Yamazaki N 2000: Method of effective fertilizer application for open field netted melon in sand dune. Jpn. J. Sci. Plant Nutr, 71, 888–892 in Japanese
- Wada S-I 1987: Recent development in thermodynamics of ion exchange in clays. Nendo Kagaku, 27, 227–236 in Japanese
- Wada S-I 1988: Ion exchange in soils (2) variable charge characteristics of soil. Jpn. J. Sci. Plant Nutr., 59, 435–439 in Japanese
- Wuxian Z, Kinomura N, Sakane H, Suzuki T 1995: Synthesis of Mg/Fe puroaurite-like compounds and their anion-exchange characteristics. Inorg. Mater., 2, 480–485.
- Wuxian Z, Sakane H, Hatsushika T, Kinomura N, Suzuki T 1996: Preparation of pyroaurite-like compounds with ion-memory effect for nitrate ion. Inorg. Mater., 3, 225–230 in Japanese with English summary
- Yamamoto S 2006: Effect of the nitrate ion absorption characteristics of a hydrotalcite-like mineral and improvement of nitric ion retention capacity in soil. Jpn. Soc. Sand Dune Res. Lecture Summaries, 53, 18–19 in Japanese