ABSTRACT
Due to a decrease in phosphorus (P) and potassium (K) mining, manure is incinerated to concentrate P and K in ash. To understand the alternative use of manure-derived ash as P and K sources, laboratory and greenhouse experiments were conducted to determine the relationship between extractability and P and K uptake in cattle manure ash (CMA) and that between CMA application and a grass tetany hazard. The results showed that more P was extracted with 2% citric acid (90% of the total P) than with 2% formic acid (72–84% of the total P). Ninety-one percent of the total K was soluble in water. A greenhouse pot experiment was conducted to test P and K availability to Guinea grass (Megathyrsus maximus). Cattle manure ash or calcium dihydrogen phosphate (CF) was incorporated into sandy soil at 10, 20, and 50 g P2O5 m−2. Two combinations of CMA and CF were tested at 20 g P2O5 m−2. Potassium rates followed K content in CMA applied at different rates of P equivalent to 19, 38, or 96 g K2O m−2. In four harvests, there was no significant difference in the total yields between CMA and CF treatments. The total P uptake was significantly lower in the CMA treatment than in the CF treatment, while it was not in the combined CMA and CF treatments. The P uptake in response to different extraction methods indicated that the extraction of P by 2% formic acid without sonication is recommended to predict P availability in CMA. The potassium uptake from CMA application was comparable to that from the KCl application, and excessive K occurred at 38 and 96 g K2O m−2. The grass tetany hazard ratio higher than 2.2 was observed at the beginning period at the lowest application rates of CMA and CF. In conclusion, the combination use of CMA and CF was better than the single use of CMA. Moreover, CMA would be an available K source, but the grass tetany hazard still needs to be considered in application rates and pretreatments.
1. Introduction
Depletion of fossil phosphorus (P) resources requires an alternative P source for agricultural use. For the sustainable supply of P to agriculture, new technologies of P recycle are necessary in countries where the natural P resource is limited, like Japan. According to Matsubae-Yokoyama et al. (Citation2009), livestock manure, sewage sludge, and steel slag are potential sources for P recycle in Japan. The annual production of livestock manure in Japan has reached 8.8 × 107 t in wet weight (MAFF Citation2011), including 6.8 × 105 t nitrogen (N) and 1.2 × 105 t P (Mishima et al. Citation2009). Livestock manure is usually composted to amend soil. Improper composting, however, can cause serious environmental problems such as runoff and leaching of N, P, and potassium (K) into surface and ground waters (Eghball et al. Citation1997) and emissions of greenhouse gases (N2O and CH4) (Smith et al. Citation2008). Recently, animal manure has been incinerated to remove organic matter and water and to concentrate nutrients in ash, especially P and K, which are limited resources in the world. The recoveries of P and K are, respectively, 76–89% and 56–95% for cattle manure ash, 90–100% and 61–80% for laying-hen manure ash, and 95–100% and 57–78% for swine manure ash (Huang et al. Citation2011). Komiyama et al. (Citation2013) reported that the contents of P and K in ash are 3–4% and 4–5% for cattle manure, 11–12% and 9% for swine manure, 5–7% and 6–7% for layer manure, and 10% and 16% for broiler manure, respectively. Ash derived from livestock manure has been recently considered as an alternative P and K fertilizer to mitigate the aforementioned risks.
Although ash derived from sewage sludge contains a high amount of P (Anderson Citation2002; Schipper et al. Citation2001), sewage sludge ash cannot be directly applied to soil as a P fertilizer due to high contents of heavy metals (Herzel et al. Citation2016). In contrast, direct application of ash from livestock manure as an alternative P fertilizer has been tested recently and revealed in the potential utility of this source. Particularly, some studies suggested the potential use of ash derived from turkey litter (Pagliari et al. Citation2010) or poultry litter (Codling et al. Citation2002) as a P fertilizer. They demonstrated that the growth and P uptake of corn and wheat were similar to ash and chemical fertilizer treatments. In contrast, because P compounds are converted from highly water-soluble forms to less water-soluble forms during incineration (Komiyama et al. Citation2013), ash is considered to be a slow-release source of P for plants (Christel et al. Citation2014). Pagliari et al. (Citation2010) observed the initial plant development of corn to be 15–20% higher with a triple-phosphate fertilizer than that with turkey manure ash. However, there is little information on determining plant-available P in ash. Codling et al. (Citation2002) suggested that the appropriate application rates of poultry litter ash should be determined in further studies. Although bioassay is the most reliable method, it consumes time and money. Extraction of P using chemicals is a simple approach to predict P availability in ash. Extractants such as 2% formic acid, 2% citric acid, and 1 M neutral ammonium citrate were used to predict the P availability of phosphate rock (Rajan et al. Citation1992) and biochar derived from biosolids (produced from wastewater treatment) and cattle manure (Wang et al. Citation2012). These chemical extraction methods are thus useful for testing the availability of P in ash.
There are few studies on the availability of K from manure-derived ash, although it contains a high concentration of K, as mentioned earlier. The availability of K in ash to plants has been controversial. According to Komiyama et al. (Citation2013), K compounds in cattle and swine manure ash are soluble in water. They also reported no significant difference in K uptake between layer manure ash and chemical fertilizers. However, low K uptake by wheat from poultry litter ash resulted from less available K in the ash (Codling et al. Citation2002). Therefore, K availability of CMA should be further studied.
Ash also contains high contents of calcium (Ca) and magnesium (Mg) (Ohno and Erich Citation1990; Unger and Fernandez Citation1990), which enrich Mg and Ca in soil and affect uptake balances of Ca, Mg, and K (Ohno and Erich Citation1990). The availability of Ca and Mg depends on the source of ash amended in soil. For example, the amendment of coal ash increases the availability of Mg and Ca in soil and their uptake by barley, corn, and lettuce (Bierman and Rosen Citation1994; Hammermeister et al. Citation1998), whereas the addition of poultry litter ash showed less Mg uptake by wheat (Codling et al. Citation2002). High uptake of K by plants, especially by forage, depresses Mg and Ca concentration in plants (Grunes and Welch Citation1989), and subsequently causes the risk of grass tetany for ruminants (Grunes et al. Citation1970). The availability of Mg and Ca should be determined to evaluate the risk of grass tetany caused by the availability of K in ash.
Livestock manure per cropland was larger in intensive livestock farming areas in Kagoshima, Miyazaki, Tokushima, Aichi, and Iwate (Tsuiki and Harada Citation1997). Uneven distribution of intensive livestock farms in Japan causes an excess of manure application because of high cost for transportation. Ash would be a promising fertilizer because its low transportation costs and high contents of P and K. Many studies have reported the availability of P and K in poultry litter ash, pig manure ash, or wood ash as mentioned above. The availability of P and K in cattle manure ash (CMA) is not fully understood, although cattle manure accounts for the majority of fresh livestock manure in Japan (MAFF, Tokyo Citation2011). More specifically, the relationship between the extractability of P and K and their plant uptake from manure-derived ash has not been studied extensively. Cattle manure ash was chosen in this study as a source of P and K for cultivating Guinea grass (Megathyrsus maximus). The objectives of this study were to evaluate (i) the extractable P and K in CMA, (ii) the uptake of P and K by Guinea grass in relation with their extractability, and (iii) the relationship between CMA application and the grass tetany hazard.
2. Materials and methods
2.1. Cattle manure ash
Composted cattle manure (composted daily cattle manure and beef manure ratio of 50:50) was collected from a composting facility located in Handa City, Aichi Prefecture, Japan (Oshita et al. Citation2015). Cattle manure without a treatment of solid-liquid separation was aerobically composted with rice straw for 3–6 months with turnover and finally sieved using 20-mm trommel. The water content of the compost was 39% at the collection. The compost was subsequently incinerated using a pilot-scale fluidized bed incinerator at 850°C for 10 s. The ash was digested with a mixture of 3 mL HF, 5 mL HNO3, 2 mL HCl, and 18 mL saturated H3BO3 by mixing 50 mg of CMA with 10 mL of the acid solution. The mixed samples were heated in a microwave oven at 190°C for 15 min. Finally, concentrations of P, K, Ca, Mg, and Na were determined using inductively coupled plasma atomic emission spectrometry (ICP-AES, ICPS-8000, Shimadzu, Japan) (). The electrical conductivity (EC) and pH of CMA were analyzed at a 1:10 ratio (ash:deionized water) with digital EC (DS-14, Horiba, Japan) and pH (F-23, Horiba, Japan) meters. The values of EC and pH were 47.9 dS m−1 and 9.8, respectively. The content of P in CMA was 42,500 mg kg−1, which is similar to P (41,600 mg kg−1) in another cattle manure ash (Komiyama et al. Citation2013) and slightly lower than that in poultry litter ash (Codling et al. Citation2002; Komiyama et al. Citation2013). The content of K was 155,300 mg kg−1 in CMA, while that of K was only 39,000 mg kg−1 in poultry litter ash (Codling et al. Citation2002) and 94,100 mg kg−1 in swine manure ash (Komiyama et al. Citation2013).
Table 1. Cattle manure ash properties.
2.2. Extractability of P and K in cattle manure ash
Phosphorus in CMA was extracted with 2% (w/w) citric acid (CA) or 2% formic acid (FA) according to Rajan et al. (Citation1992). An ultrasonication step in the CA and FA extractions was included to extract more P (Wang et al. Citation2012). Cattle manure ash was shaken with the extracting solution at a ratio of 1:100 on a shaker at 170 rpm for 30 min at 25°C after being dispersed by ultrasonication for 10 min. Each extraction was carried out in triplicate. The solid and extract were then separated by centrifugation at 5,000 rpm for 3 min. The supernatants were filtered through a 0.2 µm filter (Avantec, Toyo Roshi Kaisha, Ltd., Japan) before PO4-P determination with a continuous flow autoanalyzer (QuAAtro 2–HR, Bltec, Japan). In addition, water-soluble P, K, Ca, and Mg were determined at an ash:deionized water ratio of 1:100. The supernatants were subsequently centrifuged at 5000 rpm for 3 min and filtered through a 0.2 µm filter. Finally, water-soluble K, Ca, and Mg were measured with an atomic absorption spectrophotometer (AA-6800, Shimadzu, Japan).
2.3. Greenhouse pot experiment
The soil used in the present study was collected from a mountain that is used to replace cesium-137-contaminated agriculture surface soil in Fukushima, Japan. This soil has a bulk density of 1.54 Mg m−3, pH of 7.8, and not-detectable water-soluble P and Olsen-P (). Tran et al. (Citation2017) reported that in an incubation study, sandy soil in Fukushima can be improved by the application of CMA, but plant availability of P from CMA has not been examined yet.
Table 2. Properties of soil in Fukushima.
Phosphorus and K were applied either as CMA or chemical fertilizers (P as Ca(H2PO4)2H2O, K as KCl, ). Cattle manure ash or chemical fertilizer (CF) was applied at 10 (CMA10, CF10), 20 (CMA20, CF20), and 50 (CMA50, CF50) g P2O5 m−2. Because CMA was assumed to be a low-release P source, CMA and CF were combined at ratios of 50% CMA and 50% CF (R10:10) or 90% CMA and 10% CF (R18:2) at 20 g P2O5 m−2. Since CMA contains both P and K, K application rates were determined by the amount of K in CMA at different P rates, equivalent to 19, 38, and 96 g K2O m−2. A control treatment (CT) with no addition of P and K was added. Nitrogen was applied as NH4Cl at 20 g N m−2 before sowing and at 5 g N m−2 every 15 d (beginning after the first harvest) for all treatments. The application rates were determined according to the guidelines for forage crop cultivation (Department of Agriculture, Forestry, and Fishery, Yamaguchi Prefecture Citation2012). The detailed application rates are presented in . Pebbles (1.5 kg) were placed on the bottom of Wagner pots with a surface area of 200 cm2 and height of 19.8 cm and separated from soil with a nylon mesh (180 mesh). Then, 3 kg of soil with 1% water was mixed thoroughly with CMA and CF, together with NH4Cl and KCl and put into the pot. Soil moisture was maintained between 60% and 70% water holding capacity. All pots were arranged in a randomized block design in triplicate in a greenhouse. The greenhouse pot experiment was conducted from May to October 2016 at the Faculty of Agriculture, Okayama University.
Table 3. Application rates and combined application of P and K at different ratios.
After adding water to soil, 12 Guinea grass (Megathyrsus maximus) seeds were evenly placed in each pot at 1 cm depth. After germination and growth of the seedlings to a 5-cm height, the grass was thinned to four plants per pot. For harvesting, the grass was cut at 5 cm from the soil surface every 25 d (4 harvests). The grass was placed in a paper bag and then dried in an oven at 70°C for 72–96 h until it reached a constant weight. Subsequently, the dried grass was weighed and milled to a powder to determine contents of P, K, Mg, and Ca after Kjeldahl digestion (Bremner Citation1996). The total P in plants was analyzed with the continuous flow autoanalyzer. The total K, Mg, and Ca were determined by the atomic absorption spectrophotometer.
2.4. Data analysis
The results are expressed as an average of three replicates with standard deviation (SD). One-way ANOVA analysis was performed to evaluate statistical differences in grass yield and P uptake among treatments by SPSS software 15.0. The significant difference was determined according to the Tukey HSD test at a level of 0.05.
3. Results and discussion
3.1. Extractability of P and K in cattle manure ash
The results of P and K extractions from CMA are presented in . Different extractants affected P extraction. More specifically, more P was extracted with CA (90%) than FA (72%). The effect of sonication on P extraction by CA was negligible, while sonication increased P extraction by FA. In the P fertilizer industry, P extractability by chemicals such as CA and FA is measured to evaluate the agronomic effectiveness of P fertilizer. According to Hedley and McLaughlin (Citation2005), extraction of P in phosphate rock by CA and FA is used to evaluate the reactivity of phosphate rock and as a criterion for suitability of its direct application. High amounts of P extracted by CA and FA probably indicate the suitability of direct application of CMA as a P fertilizer for plants. In this experiment, only 2.5% of P in CMA was soluble in water (), because P exists in recalcitrant forms in ash (Komiyama et al. Citation2013; Thygesen et al. Citation2011). Since P was mainly present as Ca compounds in CMA (Oshita et al. Citation2016), P was insoluble in water and soluble in 2% CA and 2% FA. Other studies (Kuligowski and Poulsen Citation2009; Kuligowski et al. Citation2008) also showed that the total water extractable P in ash at the same ratio of solid–water as our experiment was only 1% of the total P in pig manure ash. Ash application with low water-soluble P can mitigate P losses in sandy soil compared to chemical fertilizer and cattle manure compost application (Tran et al. Citation2017).
Table 4. Extractability of nutrient elements in cattle manure ash. Fraction is the % of the total P, K, Mg, and Ca in CMA.
Up to 91% of the total K in CMA was soluble in water. Rubæk et al. (Citation2006) reported that the solubility of K depends on types of ash, and 35% and 100% of the total K in pig manure and poultry litter ash were soluble in water, respectively. In agreement with previous studies, K in ash derived from poultry litter, manure cattle manure, swine manure (Komiyama et al. Citation2013), and wood (Ulery et al. Citation1993) was soluble in water. Potassium chloride was a main form of K in CMA and easily leached with water (Oshita et al. Citation2016). Komiyama et al. (Citation2013) also found that K existing as KCl and K2SO4 in ash derived from cattle manure, swine manure, layer manure, and broiler manure is soluble in water. The solubility of Mg and Ca in water was only 1.3% and 1.1% of the total Mg and Ca in CMA, respectively. In agreement with Oshita et al. (Citation2016), 99% of Ca and Mg was not removed from cattle manure ash by washing with water.
3.2. Guinea grass yields and P uptake in relation to P solubility in cattle manure ash
Monthly average temperatures and humidity fluctuated from 23°C to 32°C and 62% to 75%, respectively. Both Guinea grass yield () and P uptake () increased significantly with amendment of CMA and CF compared with the control (p < 0.05). All plants in the CF50 treatment died after germination, while plants in CMA50 treatment developed normally. The reason is unknown in this study. The total plant yields in the CMA treatment were comparable to that in the CF treatments (p > 0.05). Increasing the application rates of CMA and CF had no significant effect on the total plant yield (p > 0.05), although the treatments with higher rates of P always produced higher yields. Yields of each harvest also were not significantly different between CMA- and CF-amended treatments (p > 0.05). Similarly, plant yields with ash from poultry litter (Codling et al. Citation2002; Komiyama et al. Citation2013), turkey manure (Pagliari et al. Citation2010), and wood (Cavaleri et al. Citation2004) were equal to those with P chemical fertilizer. Because Guinea grass yields were higher in CMA-amended treatments than in the CT treatment and not significantly different among CMA treatments, the CMA10 would be appropriate application rate of CMA to prevent excess application of P in this study.
Figure 1. Shoot dry matter yields and root dry weight of Guinea grass cultivated in pots with soil fertilized with cattle manure ash or chemical fertilizer. Lower case is used for each harvest and root weights; capital letters indicate the total biomass. Data are from four harvests and root residue (RR). All plants in CF50 died. Different letters represent significant differences (p < 0.05).
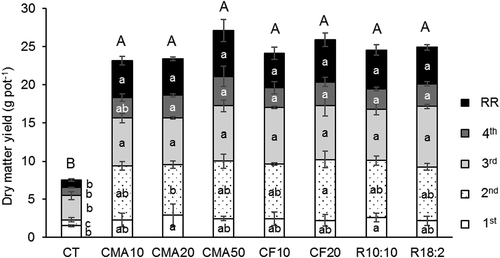
Figure 2. Phosphorus uptake of Guinea grass cultivated in pots with soil fertilized with cattle manure ash or chemical fertilizer. Lower case is used for each harvest and root weights; capital letters indicate the total biomass. Data are displayed from four harvests and root residue (RR). All plants in CF50 died. Different letters represent significant differences (p < 0.05).
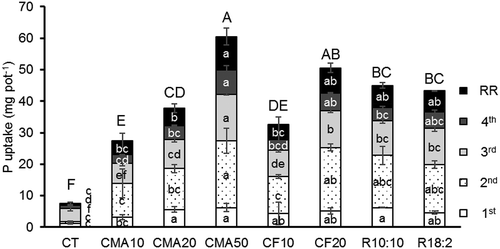
The total P uptake was, however, more sensitive to the application rates of P and sources of P than the total grass yield (). Increasing the rates of CMA and CF produced a significant increase of P uptake (p < 0.05). Particularly, the total P uptake was significantly greater in the CF20 treatment than in the CMA20 treatment, while the difference between CMA10 and CF10 was not significant. In contrast, there was no significant difference in P uptake at each harvest (except for P uptake in CMA20 and CF20 treatments at the third harvest) at the same rate between CMA and CF treatments. The percent of the total P uptake per total P addition was 15–33% in CMA treatments, 30–39% in CF treatments, and 26–27% in combination treatments. According to Wild (Citation1988), annual crops usually take up about 5–25% of the phosphate applied, and the accumulated P was converted into non-labile form. The combination treatments of CMA and CF showed no significant difference of P uptake compared to the CF and CMA20 treatments (p > 0.05). The lower total P uptake in CMA20 than in CF20 can be explained by the existing recalcitrant P compounds in CMA. Tran et al. (Citation2017) also observed that P release from soil amended with CMA was lower than that from soil with chemical fertilizer or cattle manure compost in a laboratory study. The present result is consistent with Kuligowski et al. (Citation2012), who reported that P uptake by ryegrass from chicken litter ash was lower than that from chemical fertilizer. Pagliari et al. (Citation2010) reported a lower uptake of P from ash derived from turkey manure compared to conventional fertilizer at the initial stage of corn growth. However, the P concentration in wheat was higher with poultry litter ash application than with chemical P application (Codling et al. Citation2002).
Many studies have used both plant yields and P uptake to evaluate P availability from rock phosphate (Rajan et al. Citation1992) and sewage sludge (Plaza et al. Citation2007). However, P uptake is more susceptible than plant yield for evaluating P availability (Plaza et al. Citation2007; Wang et al. Citation2012). Overall, the responses of P uptake to CMA and CF application were more different than those of plant yields. Phosphorus uptake was thus considered to be a more valid parameter for evaluating P availability from CMA application compared to grass yield and was used to compare the relationship with the chemical extractants. Because P in CF (Ca(H2PO4)2H2O) is highly soluble in water (Mullins and Sikora Citation1994), P in CF is assumed to be readily available to plants. The plant available P of CF treatments (CF TP, solid line) in A,B were therefore the highest in the present study. In Fig. 3b, the data of P extracted with FA (CMA FA-P) were asymptotic to that of CF TP. This indicates that the FA-P without sonication was a more reliable parameter than the others to predict the available P in CMA. Rajan et al. (Citation1992) reported that the extraction of P with FA was a good parameter to test plant-available P in phosphate rocks, which is similar to our result.
Figure 3. Relationships between (a) dry matter yields and extractable P addition; (b) plant P uptake and extractable P addition. Data are displayed from the total P (TP), 2% formic acid extractable P with or without the ultra-sonication (FA, FAs), and 2% citric acid extractable P with or without the ultra-sonication (CA, CAs).
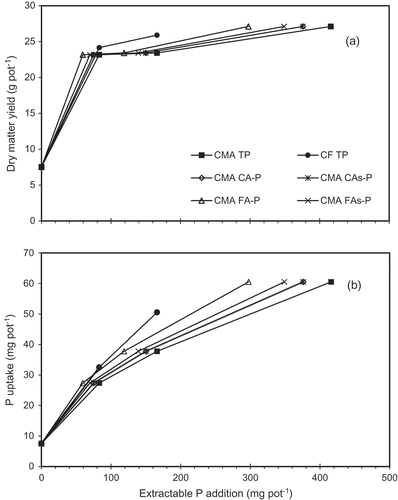
3.3. Potassium availability in relation to grass tetany hazard
Potassium availability from CMA application
shows K uptake by Guinea grass from the different sources at different application rates of K. The addition of K from CMA and CF to the soil significantly increased K uptake (p < 0.05). There was no significant difference in K uptake (p > 0.05), despite increasing the application rates and different types of K source. The availability of K in CMA is explained by the high solubility of K in water (). Similarly, ash derived from layer manure (Komiyama et al. Citation2013) and wood (Haraldsen et al. Citation2011; Sharifi et al. Citation2013) can be used as a K fertilizer for plants. However, Codling et al. (Citation2002) reported that K uptake by wheat was lower from poultry litter ash than from a chemical fertilizer because of less available K and low amount of K. In this study, increasing the amounts of CMA or CF from 16.0 to 79.8 g K m−2 did not increase K uptake in CMA and CF treatments. Adding K was sufficient for plants at the lowest application rate during the experiment period. Amounts of available K in CMA20 and CMA50 treatments were greater than the grass K requirement. Thus, 26% and 65% (data not shown) of K were not used by grass and accumulated in soil in CMA20 and CMA50 treatments, respectively. Sharifi et al. (Citation2013) also reported that available K ranging 38–96% of the total K in wood ash was greater than K requirement by ryegrass, and some of the available K remained in soil after the experiment. Because the K content (155,300 mg kg−1) is approximately four times higher than the P content (42,500 mg kg−1) in CMA, K addition from CMA caused K abundance in soil in the present study. Because K in CMA was soluble in water, the excess of K probably creates a risk of K leaching. As a result, CMA is an available K source comparable to chemical fertilizer for grass, but the application rates should be considered to mitigate K accumulation in soil. In the viewpoint of K uptake and risk of K leaching, the lowest rate of CMA was recommendable in this study.
Figure 4. Potassium uptake of guinea grass cultivated in pots with soil fertilized with cattle manure ash or chemical fertilizer. Lower case was used for each harvest and root weights; capital letters indicate the total biomass. Data were displayed from four harvests and root residue (RR). All plants in CF50 died. Different letters represent significant differences (p < 0.05).
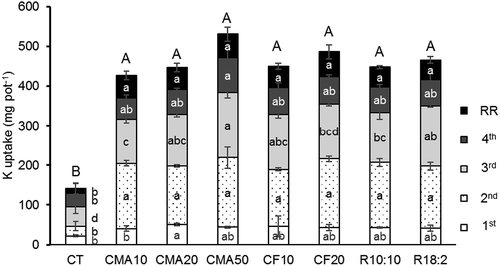
Grass tetany hazard
Increasing application rates of CMA did not significantly change contents of Mg and Ca in the grass (). The Ca content was not different among treatments because of high amount of exchangeable Ca () in the initial soil. Low content of Mg accompanied by low content of Ca in forage also leads to a high risk of grass tetany in ruminants (Grunes et al. Citation1970). In the present study, the Mg in grass at each harvest in CMA and CF treatments were from 1.07 to 1.93 g kg−1, while the recommended safe level in forage is above 2 g kg−1 (Grunes et al. Citation1970). The Ca levels of grass found in the treatments amended with CMA and CF were also relatively low, with range from 2.03 to 4.82 g kg−1, which was lower than safe levels (over 6 g kg−1) mentioned by Metson et al. (Citation1966).
Table 5. Contents of Ca and Mg in Guinea grass shoots (4 harvests) and root-residue.
In this study, the K/(Ca+Mg) ratios were close to 2.2 (), which is hazardous for ruminants (Grunes and Mayland Citation1975; Walker and Graffis Citation1979). It can be explained by the large application rates and high solubility of K from CMA and KCl, which would have depressed Mg content in grass as mentioned earlier. Mozaffari et al. (Citation2002) also observed that the Mg content in corn shoot was reduced with increasing application rates of K derived from alfalfa ash. The less water-soluble Mg in CMA was probably another reason for the low Mg contents in grass. Another possible factor is the ammonium source (NH4Cl) used as an N source in the experiment. Grunes and Welch (Citation1989) indicated that application of ammonium-N resulted in lower uptake of Mg and Ca by plants than nitrate did.
Table 6. Effects of application of CMA on tetany hazard risk based on the ratio of K/(Ca + Mg)a.
The addition of CMA should be reduced to avoid excess application of P and K. To reduce the amount of K added by CMA, a combination of CMA and conventional P fertilizer and/or the use of CMA washed with water (Oshita et al. Citation2016; Tran et al. Citationin press) could be adopted. Further studies are required to mitigate the risk of grass tetany caused by CMA application. On the other hand, the application of CMA would be promising for crops other than forage crops. For example, turkey manure ash, which contains K as much as in the ash used in the present study, was a potential P and K source for corn (Pagliari et al. Citation2010).
4. Conclusions
The study aimed at evaluating the extractable P and K in CMA, the uptake of P and K by Guinea grass in relation with their extractability, and the grass tetany hazard. The extractable P in CMA was in the order of CA-P > FA-P > water-soluble P. Up to 91% of the total K in CMA was soluble in water. The greenhouse experiment revealed no significant difference in the grass yield regardless of the source of P and application rates. However, the P uptake was significantly lower in ash-amended treatments than in the chemical fertilizer-amended treatment. The P uptake indicated that 2% formic acid extraction without sonication can be used to predict the P availability in CMA. Potassium from CMA was available to grass and comparable to K from CF, but the supply was excessive in CMA20 and CMA50 treatments. Grass tetany ratio was around 2.2 in all treatments except the control. High available K in CMA depressed the Mg and Ca contents in the grass and increased the potential hazard of grass tetany, regardless of application rates of CMA. The lowest application rate (10 g P2O5 m−2) of CMA was better from the viewpoint of grass yields in this experiment. However, this application rate caused the risk of grass tetany. We further need to examine less application effects of CMA. CMA washed with water and combining use of CMA and another P source (free K) could be options to reduce excess K addition from CMA. The direct application of CMA to other crops should be considered in further studies. Because CMA was one case of mixture between daily cattle manure and beef manure, further studies are suggested to evaluate the potential use of other cattle manure ashes in other regions in Japan.
References
- Anderson M 2002: Encouraging prospects for recycling incinerated sewage sludge ash (ISSA) into clay-based building products. J. Chem. Technol. Biotechnol. 77, 352–360. doi:10.1002/jctb.586
- Bierman PM, Rosen CJ 1994: Sewage sludge incinerator ash effects on soil chemical properties and growth of lettuce and corn. Commun. Soil Sci. Plant Anal. 25, 2409–2437. doi:10.1080/00103629409369197
- Bremner JM 1996: Nitrogen-total. In Methods of Soil Analysis Part 3—Chemical Methods, Eds. Sparks DL, Page AL, Helmke PA, Loeppert RH. Soil Science Society of America, American Society of Agronomy, Wisconsin, pp. 1085–1121.
- Cavaleri MA, Gilmore DW, Mozaffari M, Rosen CJ, Halbach TR 2004: Hybrid poplar and forest soil response to municipal and industrial by-products. J. Environ. Qual. 33, 1055–1061. doi:10.2134/jeq2004.1055
- Christel W, Bruun S, Magid J, Jensen LS 2014: Phosphorus availability from the solid fraction of pig slurry is altered by composting or thermal treatment. Bioresour. Technol. 169, 543–551. doi:10.1016/j.biortech.2014.07.030
- Codling EE, Chaney RL, Sherwell J 2002: Poultry litter ash as a potential phosphorus source for agricultural crops. J. Environ. Qual. 31, 954–961. doi:10.2134/jeq2002.9540
- Department of Agriculture, Forestry, and Fisheries,Yamaguchi Prefecture 2012: Guidline for forage crop cultivation. http://www.pref.yamaguchi.lg.jp/cmsdata/1/a/e/1ae20c7cc1041764247ad6035cece9de.pdf (in Japanese)
- Eghball B, Power JF, Gilley JE, Doran JW 1997: Nutrient, carbon, and mass loss during composting of beef cattle feedlot manure. J. Environ. Qual. 26, 189–193. doi:10.2134/jeq1997.00472425002600010027x
- Grunes D, Welch R 1989: Plant contents of magnesium, calcium and potassium in relation to ruminant nutrition. J. Anim. Sci. 67, 3485–3494. doi:10.2527/jas1989.67123485x
- Grunes DL, Mayland HF 1975: Controlling Grass Tetany. USDA Leaflet 561. U.S Government Printing Office, Washington, DC.
- Grunes DL, Stout PR, Brownell JR 1970: Grass tetany of ruminants. Adv. Agron. 22, 331–374.
- Hammermeister AM, Naeth MA, Chanasyk DS 1998: Implications of fly ash application to soil for plant growth and feed quality. Environ. Technol. 19, 143–152. doi:10.1080/09593331908616666
- Haraldsen TK, Pedersen PA, Grønlund A 2011: Mixtures of bottom wood ash and meat and bone meal as NPK fertilizer. In Recycling of Biomass Ashes, Eds. Insam H, Knapp BA Springer Berlin Heidelberg, Berlin, Heidelberg, pp. 33–44.
- Hedley M, McLaughlin M 2005: Reactions of phosphate fertilizers and by-products in soils. In Phosphorus: agriculture and the Environment, Eds. Sims JT, Sharpley AN, American Society of Agronomy, Madison, WI, pp. 181–252.
- Herzel H, Krüger O, Hermann L, Adam C 2016: Sewage sludge ash - a promising secondary phosphorus source for fertilizer production. Sci. Total Environ. 542, 1136–1143. doi:10.1016/j.scitotenv.2015.08.059
- Huang Y, Dong H, Shang B, Xin H, Zhu Z 2011: Characterization of animal manure and cornstalk ashes as affected by incineration temperature. Appl. Energy 88, 947–952. doi:10.1016/j.apenergy.2010.08.011
- Komiyama T, Kobayashi A, Yahagi M 2013: The chemical characteristics of ashes from cattle, swine and poultry manure. J. Mater. Cycles Waste Manage. 15, 106–110. doi:10.1016/j.wasman.2009.04.004
- Kuligowski K, Gilkes RJ, Poulsen TG, Yusiharni BE 2012: Ash from the thermal gasification of pig manure—effects on ryegrass yield, element uptake, and soil properties. Soil Res. 50, 406–415. doi:10.1071/SR12075
- Kuligowski K, Poulsen TG 2009: Phosphorus leaching from soils amended with thermally gasified piggery waste ash. Waste Manage. 29, 2500–2508. doi:10.1016/j.wasman.2009.04.004
- Kuligowski K, Poulsen TG, Stoholm P, Pind N, Laursen J 2008: Nutrients and heavy metals distribution in thermally treated pig manure. Waste Manage. Res. 26, 347–354. doi:10.1177/0734242X08090075
- MAFF, Tokyo 2011: Status of emission and management of livestock manure. http://www.maff.go.jp/j/chikusan/kankyo/taisaku/t_mondai/02_kanri/index.html (in Japanese).
- Matsubae-Yokoyama K, Kubo H, Nakajima K, Nagasaka T 2009: A material flow analysis of phosphorus in Japan. J Ind Ecol 13, 687–705. doi:10.1111/j.1530-9290.2009.00162.x
- Metson AJ, Saunders WMH, Collie TW, Graham VW 1966: Chemical composition of pastures in relation to grass tetany in beef breeding cows. N. Z. J. Agric. Res. 9, 410–436. doi:10.1080/00288233.1966.10420793
- Mishima S-I, Endo A, Shirato Y, Kimura SD 2009: Quantity of organic waste resources in Japan and capacity of local farmland to receive composted wastes (in Japanese with English summary). Jap. Journ. Soil Sci. Plant Nutr. 80, 226–232.
- Mozaffari M, Russelle MP, Rosen CJ, Nater EA 2002: Nutrient supply and neutralizing value of alfalfa stem gasification ash. Soil Sci. Soc. Am. J. 66, 171–178. doi:10.2136/sssaj2002.1710
- Mullins GL, Sikora FJ 1994: Effect of soil pH on the requirement for water-soluble phosphorus in triple superphosphate fertilizers. Fertil. Res. 40, 207–214. doi:10.1007/BF00750467
- Ohno T, Erich M 1990: Effect of wood ash application on soil pH and soil test nutrient levels. Agric., Ecosyst. Environ. 32, 223–239. doi:10.1016/0167-8809(90)90162-7
- Oshita K, Kawaguchi K, Takaoka M, Matsukawa K, Fujimori T, Fujiwara T 2015: Emission and control of nitrous oxide N2O and composition of ash derived from cattle manure combustion using a pilot-scale fluidized bed incinerator. Environ. Technol. 37, 439–445. doi:10.1080/09593330.2015.1077190
- Oshita K, Sun X, Kawaguchi K, Shiota K, Takaoka M, Matsukawa K, Fujiwara T 2016: Aqueous leaching of cattle manure incineration ash to produce a phosphate enriched fertilizer. J. Mater. Cycles Waste Manage. 18, 608–617. doi:10.1007/s10163-016-0528-6
- Pagliari P, Rosen C, Strock J, Russelle M 2010: Phosphorus availability and early corn growth response in soil amended with turkey manure ash. Commun. Soil Sci. Plant Anal. 41, 1369–1382. doi:10.1080/00103621003759379
- Plaza C, Sanz R, Clemente C, Fernández JM, González R, Polo A, Colmenarejo MF 2007: Greenhouse evaluation of struvite and sludges from municipal wastewater treatment works as phosphorus sources for plants. J. Agric. Food Chem. 55, 8206–8212. doi:10.1021/jf071563y
- Rajan SSS, Brown MW, Boyes MK, Upsdell MP 1992: Extractable phosphorus to predict agronomic effectiveness of ground and unground phosphate rocks. Fertil. Res. 32, 291–302. doi:10.1007/BF01050366
- Rubæk GH, Stoholm P, Sørensen P 2006: Availability of P and K in ash from thermal gasification of animal manure. In: Petersen SO Ed. Proceedings from the 12th Ramiran International Conference on Technology for Recycling of Manure Organic Residues in a Whole-Farm Perspective. Vol. II, pp. 177–180. Department of Agroecology, Danish Institute of Agricultural Sciences, Tjele.
- Schipper WJ, Klapwijk A, Potjer B, Rulkens WH, Temmink BG, Kiestra FDG, Lijmbach ACM 2001: Phosphate recycling in the phosphorus industry. Environ. Technol. 22, 1337–1345. doi:10.1080/09593330.2001.9619173
- Sharifi M, Cheema M, McVicar K, LeBlanc L, Fillmore S 2013: Evaluation of liming properties and potassium bioavailability of three Atlantic Canada wood ash sources. Can. J. Plant Sci. 93, 1209–1216. doi:10.4141/cjps2013-168
- Smith P, Martino D, Cai Z et al. 2008: Greenhouse gas mitigation in agriculture. Philos. Trans. R. Soc. Lond., B, Biol. Sci. 363, 789–813. doi:10.1098/rstb.2007.2184
- Thygesen AM, Wernberg O, Skou E, Sommer SG 2011: Effect of incineration temperature on phosphorus availability in bio-ash from manure. Environ. Technol. 32, 633–638. doi:10.1080/09593330.2010.509355
- Tran QT, Maeda M, Oshita K, Takaoka M 2017: Phosphorus release from cattle manure ash as soil amendment in laboratory-scale tests. Jap. Journ. Soil Sci. Plant Nutr. 63, 369–376. doi:10.1080/00380768.2017.1355217
- Tran QT, Maeda M, Oshita K, Takaoka M, Fujiwara T in press: Effects of a washing process of cattle manure ash on root and shoot growth of Komatsuna (Brassica rapa var. perviridis) at the seedling stage. J.Environ.Sci.Sustain.Soci 8.
- Tsuiki M, Harada Y 1997: A computer program of estimating the amount of livestock waste. J. Jpn. Agricsyst. Soc. 13, 17–23.
- Ulery AL, Graham RC, Amrhein C 1993: Wood-ash composition and soil pH following intense burning. Soil Sci. 156, 358–364. doi:10.1097/00010694-199311000-00008
- Unger YL, Fernandez IJ 1990: The short-term effects of wood-ash amendment on forest soils. Water Air Soil Pollut. 49, 299–314.
- Walker WM, Graffis DW 1979: Grass species and cultivar differences in magnesium concentration. Commun. Soil Sci. Plant Anal. 10, 1239–1248. doi:10.1080/00103627909366977
- Wang T, Camps-Arbestain M, Hedley M, Bishop P 2012: Predicting phosphorus bioavailability from high-ash biochars. Plant Soil 357, 173–187. doi:10.1007/s11104-012-1131-9
- Wild A 1988: Plant nutrients in soil: phosphate. In Russell’s Soil Conditions and Plant Growth, Ed. Wild A. Longman Scientific and Technical, Harlow, pp. 695–742.