ABSTRACT
To successfully afforest coastal forest belts ensuring high disaster prevention, growth bases for them have been constructed by piling up soil in the low wetlands along the Kujukuri coastline. Ground surfaces in such bases are often covered with water because of soil compaction, leaving them susceptible to stagnant water. Water stagnation in soil is problematic, potentially interfering with afforesting coastal forests. Therefore, row deep tillage was conducted for parts of growth bases to combat the poor physical properties of the existing soil. Here, we surveyed soil profiles and measured vertical soil hardness distribution in two forest stands with piling up soil to evaluate the effectiveness of tillage for man-made soil. Soil hardness measurements indicated that the vertical areas with ‘soft’ and ‘hard/consolidated’ soil alternately appeared in growth base profiles. Generally, soil of the dense and very hard layers was apt to be formed by strong compaction of the filled-up soil because of heavy machinery usage during growth base preparation. Such dense and hard soils in the untilled areas of the profiles were also observed in this study. By contrast, it was confirmed that row deep tillage drastically improved soil physical properties, i.e., decreased hardness and increased water permeability, because the sequential hardened subsoil layers were well-broken-up. Moreover, it was observed many thick and large roots penetrated deeper layers with deep tillage areas. These results suggest that row deep tillage of hardened soil is quite effective at securing the areas and providing the physical conditions for deeply penetrated roots into deeper soils, which increases healthy root development. They show the effects of soil hardness reduction and water permeability improvement have been maintained for two decades, at least, after construction. These findings will be useful for alleviating some problems of soil compaction, water stagnation, and tree-growth hindrance that have been encountered on afforestation sites with man-made soil.
1. Introduction
Coastal forests perform an important role in disaster prevention against sea breeze, tide, and blown sand. They also provide landscape amenities and places for recreation (Murai et al. Citation1992). To ensure fulfillment of those roles, healthy coastal forest lands need to be constructed.
Japanese black pines (Pinus thunbergii Parlat.) on the seashore of Kujukuri and Futtsu-Misaki in Chiba Prefecture, Japan, often wither in low-lying sandy areas (lower than 5 m above sea level [ASL]) with a high groundwater level behind coastal sand dunes (Oda Citation2001; Nohara and Takahashi Citation2007). The areas of the coastal prevention forests with a high groundwater level (>−1.0 m below ground level) in Chiba were estimated to be 287 ha, accounting for 29% of total prefectural coastal forestland area (Chiba Prefecture Citation1997). Of these, 81 ha of areas designated as coastal prevention forestlands were treeless (Chiba Prefecture Citation1997). Water stagnation periods on the ground surface in the low-lying forestlands ranged from 0 to 5 months per year at the Kujukuri coastline (Oda Citation2001). In some areas of the town of Ichinomiya along the Kujukuri coastline, water stagnation periods extended for 9–11 months during the year (Oda Citation2001). Black pine is originally a deep-rooted tree (Karizumi Citation2010). Thus, its main root can penetrate deeply if the soil condition is satisfactory (i.e., the maximum penetration of roots was 5.45 m for 35-year-old black pine trees in Tozaki, Chiba; Karizumi Citation1990). Conversely, the root systems of black pine trees that grow in damp low-lying sandy areas are mostly distributed in the shallow topsoil layers and do not develop below the groundwater level, even if its soil has high water permeability (Oda Citation2001; Karizumi Citation2010). Such damp subsoil conditions could influence healthy root development of planted seedlings. To successfully afforest the coastal forests to ensure high disaster prevention, the Forestry Research Institute in the Chiba Prefectural Agriculture and Forestry Research Center has proposed and strongly recommended that plant growth bases for coastal prevention forests are constructed. Since the 1990s, this new afforestation method for coastal prevention forests has been done by piling up soil on the low-lying areas before planting seedlings (Oda Citation2001). This is an extremely important proposal, especially for forestry in Japan, because ‘the right tree for the right land’ is a fundamental principle for traditional afforestation methods in Japan (Morisada Citation1993). For example, in Japan, the rather dry sandy soil on the coastlines with sunny conditions are generally among ‘the right lands’ for black pine tree growth (Nakagawa Citation1994) because black pine demands much light and is resistant to the sea breeze (Karizumi Citation1990). According to this proposal, coastal forests in Chiba have been developed by piling up soil in the wetlands along the coastline of Kujukuri and Futtsu-Misaki (Nohara and Takahashi Citation2007). A stand age of 50 years is the goal in afforested sites in Chiba Prefecture, and Oda (Citation2000) estimated that it is necessary to ensure a 1.2-m soil thickness for coastal forests to reach the 50-year goal. Considering that filled land settles, it is necessary to initially ensure a 1.8-m-thick layer of filled-up materials (Nohara and Takahashi Citation2007).
The required soil properties of growth bases for healthy plant growth are as follows: high water permeability (drainage), suitable soil hardness, moderate acidity (pH), and no harmful materials (Research Committee of Japanese Institute of Landscape Architecture Citation2000). However, in the growth bases constructed for abovementioned purpose, the root systems of planted trees were mostly distributed in the shallow topsoil layers (<0.45 m in depth), thus they hardly developed in the deeper layers for the first decade after planting. Nevertheless, afforestation in piled-up soil areas generally shows high survival rates (around 90%) of the planted trees and satisfactory growth (average of height (H): 3.8 m, average of diameter at breast height (DBH): 6 cm) of aboveground plants (Nohara and Takahashi Citation2007). Incidentally, survival rates of black pine trees (average H: 5.0 m, average DBH, 8 cm) for the lowland ranged from 0% (altitude, below 1.4 m ASL; maximum groundwater level was 1.37 m ASL in February 1989) to 30% (altitude, over 2.4 m ASL; maximum groundwater level was 1.47 m ASL in March 1989) before constructing growth bases by piling up soil (Forestry Research Center of Civil Engineering Facilities Citation1990). One reason why roots did not develop might be because of the hardness of filled-up soil (Nohara and Takahashi Citation2007). It has often been reported in previous works on park development and urban greening that dense and very hard layers of soil with a low composition of macropores and low hydraulic conductivity were apt to be formed by strong compaction because of heavy machinery usage when preparing the planting base (Hasegawa Citation1985; Horn Citation2015). Such deterioration of soil physical properties in the man-made growth bases had also been pointed out in the construction of Tama New Town and the redevelopment of the Osaka Expo site (Morimoto Citation1985; Yabashi and Konko Citation1985; Yabashi and Konko Citation1987). Therefore, soil amendment has to be carried out for plant growth bases with the poor physical properties. Tillage is one of the most fundamental methods of soil amendment. Therefore, row deep tillage (1-m depth) of filled-up soil was conducted on part of the growth bases constructed in Chiba Prefectural coastal forests as countermeasures to combat the poor physical properties of the soil. In addition, the Forest Agency of Japan (FAJ) and various local public bodies (e.g., Iwate, Miyagi, and Fukushima) have eagerly tried to construct filled-up growth bases and amend the soil in forestlands to restore the coastal forests damaged by the mega-tsunami following Great East Japan Earthquake of March 2011. Taking into account that background, it is expected that the areas of the coastal forests constructed on the growth bases by piling up soils will gradually increase in Japan.
In the present study, we aimed to (1) evaluate the effectiveness of row deep tillage of man-made soil on deeply rooting of planted trees in filled-up growth bases on the basis of observation results by digging out whole root systems and soil surveys, and (2) confirm the durability of soil softening for the two decades after soil physical amendments by measuring the vertical distribution of soil hardness and analyzing soil physical properties in the growth bases of the coastal prevention forests on the Kujukuri coastline in Shirako, Chiba Prefecture. This approach allowed us to understand how important the design of the right soil physical properties of man-made growth bases is, and to steadily afforest/restore the coastal forests on the coastline of the Kujukuri and Tohoku districts in Japan.
2. Materials and methods
2.1. Study sites
A survey was conducted in 2016 in Chiba Prefectural coastal prevention forests at Ushigome (US site: 35°27′58″ N, 140°24′41″ E) and Hamashuku (HM site: 35°28′00″ N, 140°24′49″ E) in Shirako. These two forests neighbor the area of the afforestation project (). Those sites are in the central part of the Kujukuri coastline facing the Pacific Ocean. The US and HM sites were 21- and 20-year-old pine-hardwood mixed forests, respectively (). In both sites, Japanese black pine (P. thunbergii), bayberry (Myrica rubra), ubame oak (Quercus phillyraeoides), Japanese mockorange (Pittosporum tobira), and Japanese spindle (Euonymus japonicus) were planted as bare-rooted seedlings. Detailed stand descriptions of the respective sites are summarized in .
Table 1. Summary of stand description of study sites.
Figure 1. Study sites in the present study. Terrain maps and aerial photographs were obtained from the GSI website (Geospatial Information Authority of Japan Citation2017).
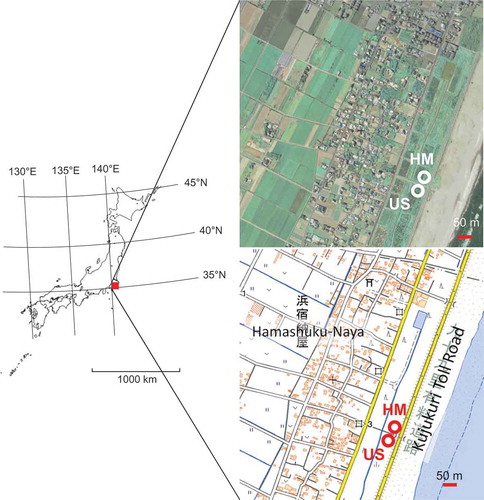
Figure 2. Overviews of the study sites in Chiba Prefectural coastal prevention forests. (a) Ushigome (US), (b) Hamashuku (HM).
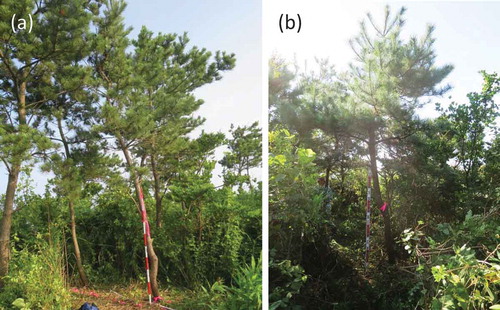
The growth bases of the coastal forests were constructed by piling up soil at the low-lying swamp areas behind the sand dunes in 1995 and 1996 at the US and HM sites, respectively. The constructed methods of bases for each site are shown in and . Both growth bases were constructed with compacting at every 0.3 m of thickness. For the upper layer (1.1–1.2 m thick) of the bases, the sandy soil purchased from a source pit in the adjacent hills was used, and the surplus soil (originating from mudstones) excavated by road and home construction was used as the bottom layer of the soil filling (0.8 m thick) (). Before tree planting, deep tillage in a row (0.6 m wide, 1.0 m deep) was conducted at intervals of 2 m, and rice straw (60 kg 100 m−2) was buried on the bottom layer. An additional layer of rice straw (60 kg 100 m−2) was also buried at a 0.3-m depth in the topsoil of the bases at intervals of 1.0 m. The planted seedling lines with deep tillage alternated with those without deep tillage. The mean annual temperature and precipitation for the last 10 years (from 2007 to 2016) of the Automated Meteorological Data Acquisition System (AMeDAS) at Yokoshiba-Hikari were 15.5°C and 1,595 mm, respectively (Japan Meteorological Agency, http://www.jma.go.jp/jma/index.html).
Figure 3. Summary of the growth mediums constructed at each site. Part of these diagrams is modified from Nohara and Takahashi’s (Citation2007) report. (a) US site, (b) HM site.
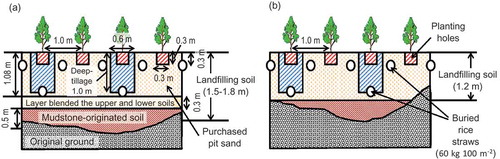
2.2. Soil survey, sampling, and analyses of the soil samples
A soil survey was conducted in the respective study sites according to Guidelines for Soil Description (FAO Citation2006). Before making soil pits, the representativeness of surveyed soil profiles regarding each site’s pedological condition was confirmed by a multipoint exploratory survey using a hand auger according to Boone et al. (Citation1999). The soil in both study sites was classified as a Spolic Technosol according to the World Reference Base for Soil Resources (FAO Citation2015). Soil samples were taken from each horizon in the respective profiles using 400-ml cylinders for soil sampling (DIK-1506; Daiki Rika Kogyo Co., Ltd. Saitama, Japan). Some soil samples in horizons that could not accommodate collection using 400-ml core cylinders were taken by 100-ml core cylinders (DIK-1801; Daiki Rika Kogyo Co., Ltd.). Those undisturbed cylinder samples collected from the present study sites were analyzed to determine the bulk density, water permeability, and porosity of the soil according to Kawada and Kojima (Citation1976). Water permeability was measured as the saturated hydraulic conductivity of each horizon in a constant water level-type permeability test (Soil permeability test apparatus, Mashimo type; Fujiwara Scientific Co., Ltd. Tokyo, Japan). Porosity was measured using the porous plate method (Soil pF measuring porous plate, Mashimo type; Fujiwara Scientific Co., Ltd.). Bulk density and volumetric distribution of three phases (solid, liquid, and gas) in soil were measured and calculated according to Marshall et al. (Citation1999). Size distributions of soil particles were measured by a laser diffraction particle size analyzer (Mastersizer 2000; Malvern Instruments Ltd., Worcestershire, UK.). Those properties regarding soil physics could become indicators of the intensity of soil compaction and hindrance on soil permeability using heavy machinery.
2.3. Evaluation of the vertical distribution of soil hardness in the growth bases
To clarify the vertical distribution of soil hardness in the growth bases of the respective sites, we settled transect lines (line length: 3.0 m) on the respective sites. We measured the vertical distribution of soil hardness to about 1.0 m deep on those lines at intervals of 0.20–0.25 m using a dynamic cone penetrometer (H-100, Daitou Techno Green, Inc.; Tokyo, Japan; ). The detailed measurement conditions for each line are summarized in . Soil hardness was indicated as ‘softness’ (cm drop−1) according to Hasegawa (Citation2008) and Miyajima et al. (Citation2015). The term ‘softness’ describes the soil penetration resistance based on the depth (cm) of the cone (diameter: 20 mmϕ) penetrating the soil per stroke by dropping a 2-kg mass hammer from a 0.5-m height. Low ‘softness’ indicates hard soil. The criteria of soil hardness were evaluated according to the five-grade system based on the Research Committee of the Japanese Institute of Landscape Architecture (Citation2000) ().
Table 2. Criteria of soil hardness (“softness”) according to the Research Committee of the Japanese Institute of Landscape Architecture (Citation2000).
To visually evaluate the vertical distribution of soil hardness, the result of each soil penetration test was described as a contour diagram in the respective sites using graphic software OriginPro 8.5J (OriginLab Corp Citation2010, Northampton, MA, USA).
2.4. Measurement of tree biomass and vertical root development
To determine the representativeness of the sampled black pine trees, the heights and DBHs of trees in the US and HM sites were measured as much as possible. Afterwards, representative types of trees on the sites were chosen (). They were harvested to measure the biomass of the above- and belowground parts. After felling, trees were individually brought to the institute and divided into needles, branches, and trunks. Large roots were manually excavated using shovels and small roots that had been cut in the field were carefully picked up from the soil by hand. To grasp the development of the vertical root systems, the maximum depth of the penetrated roots was determined in the field. All roots were washed to remove mineral soil using a high-pressure washer, and weighed using digital scales (DS10 (range 0.2–50 kg); Ohaus Corporation, Parsippany, NJ, USA). Representative subsamples (2–50 kg) were taken from parts of each type of plant for each individual and dry/fresh mass ratios were determined after ovendrying to a constant weight for 72 h or more at 70°C; longer drying times (maximum = 7 days) were required for relatively large subsamples, usually root stumps from large trees.
2.5. Comprehensive evaluation of analysis results for the constructed growth bases of coastal forests
The judgment whether the soils that we surveyed satisfy the quality management standards as the growth base of coastal forest was conformed to the ‘current specification document on erosion and torrent control and afforestation’ by FAJ (Citation2016). Specifically, the criterion of water permeability was more than 30 mm h−1, and that of soil hardness was less than 23 mm of which obtained by Yamanaka’s soil hardness tester and/or more than 1.0 cm drop−1 of which obtained by a dynamic cone penetrometer. The difference of tree sizes among the study sites were analyzed using Student’s t-test. Significant differences in this study were α < 0.05. Mean and standard deviation were calculated for each data set. All statistical analyses were performed with JMP software (SAS Institute Citation2010).
3. Results
3.1. Soil hardness distribution and soil physical properties in the growth bases with row deep tillage
Soil profile descriptions, soil physical properties, soil profile photos, contour diagrams of soil hardness, and particle size distributions of the soil in the growth bases at the US and HM sites are shown in and and –. Soil morphology in both profiles indicated similar characteristics; having thin litter layers (2–3 cm thick) of fallen pine needles and hardwood/weed foliage, having brownish-black/black topsoil layers (8–20 cm thick) of relatively soft soil (index value of Yamanaka’s hardness tester: <10 mm) with a high density of living roots, having brown subsoil layers that generally exhibit moderate soil hardness (index value of Yamanaka’s hardness tester: 13–20 mm) with low root density, and having grayish bottom soil layers formed from very firm and consolidated soil without living roots (, ). There was no structure or massive structure throughout the horizons in either soil profile except for topsoil at only the US site with a very weak fine granular structure (). According to soil hardness measurement results, the vertical areas with ‘soft’ and ‘hard/consolidated’ soils alternately appeared in the two-dimensional contour diagrams of soil hardness at both sites (). The ‘too soft’ soil layers also appeared in the topsoil layers (20–30 cm deep) in both soil profiles (). The distribution of the ‘too soft’ and ‘soft’ areas seemed to overlap with root distribution of sampled trees in both sites ( and ), although uneven vertical two-dimensional distributions of the roots were observed throughout the soil profiles ().
Table 3. Description of soil profiles in the growth bases with row deep tillage (1-m deep, 0.6-m wide) along the Kujukuri shoreline, Chiba Prefecture.
Table 4. Physical characteristics of the soil in the growth bases at sites along the Kujukuri shoreline, Chiba Prefecture.
Table 5. Datasets for the dry weights of the tree parts, total aboveground biomass (TAGB), total biomass (TB), the aboveground biomass to belowground biomass (T/R) ratio, tree height (H), diameter at ground level (D0 m), diameter at breast height (DBH), and maximum penetrated depth of vertical roots (Max. depthroot), and in the sampled trees that were represented in the study sites.
Figure 5. Soil profiles in newly constructed growth bases formed by piling up soil materials at both sites. (a) US site (for ID No. 1), (b) HM site (for ID No. 2).
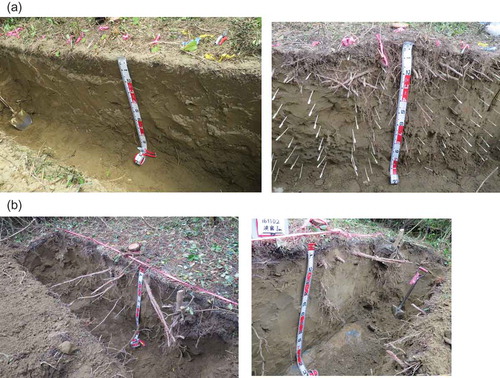
Figure 6. Contour diagrams of soil hardness in the growth bases with the row deep tillage. A part of this figure is modified from Ono and Imaya’s (Citation2017) report. (a) US site (for ID No. 1), (b) HM site (for ID No. 2), (c) HM site (for ID No. 3).
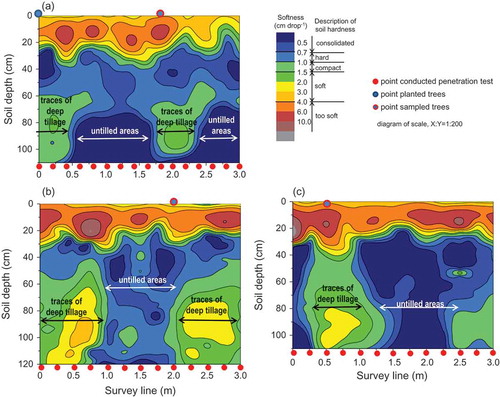
Figure 7. Soil profiles of particle size distributions in the growth bases of the study sites. (a) US site, (b) HM site.
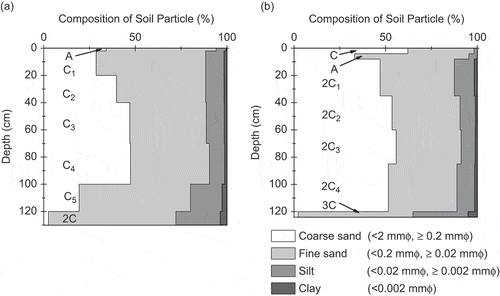
Regarding soil physical properties of the growth bases (), bulk densities of soil cores in both profiles, except for the A horizons at the US site and C-2A horizons at the HM site, were generally high, over 1.15 Mg m−3. Volume percentages of the solid phase were not so low throughout both profiles, ranging from 31 to 56 vol%. Thus, soil porosities ranged from 44 to 69 vol%. However, volume percentages of coarse pores were generally high, over 20% in most horizons of both profiles. The areas of soil layers with low coarse porosity, i.e., less than 11 vol% of coarse pores, were limited to only the C5 and 2C horizons at the US site and the 2C3 horizon at the HM site; these were mostly bottom soil layers. Maximum water-holding capacities were roughly constant throughout the soil profiles of both study sites, ranging from 41 to 66 vol%. However, the volume percentages of the liquid phase (= soil water content of cores) indicated various values, ranging from 13 to 52 vol%. The soils, except for in bottom layers, i.e., C5 and 2C horizons, in the US site were generally much drier than those in the HM site. This was a result of the different survey seasons, summer and autumn. Therefore, those values were equivalent to 19–96% of the maximum water-holding capacity (i.e., saturated water content) of each soil layer. Moreover, the minimum air capacity generally showed small values, less than 10 vol%, throughout soil profiles in both sites. The water permeability of the soil profiles in the US and HM sites was relatively high, over 40 mm h−1. Throughout the profiles, the particle size distributions of the soils in the growth bases also showed relatively similar properties except for the soils in the bottom layers, e.g., both 2C and 3C horizons at the US and HM sites (). Major size components throughout the soil profiles in both sites were sand (including coarse and fine sands), followed by silt. The soil in both sites was classified into Immature Soil according to the Classification of Forest Soils in Japan (Forest Soil Division Citation1976). They could also be classified as Spolic Technosol according to the World Reference Base for Soil Resources 2014 (FAO (Food and Agriculture Organization of the United Nations) Citation2015). These classifications were mainly because of the high content of artefacts (i.e., the use of various sources of filled materials obtained from borrow pits in hilly areas with the natural soils of the original coastal forest in swamp areas for constructing the planting medium) without low permeable horizons in both profiles.
3.2. Comparison of the biomass, sizes, and root depths of the sampled trees among the sites
shows the distribution of the size (tree height and DBH) in black pine trees grown in the US and HM sites. Trees sampled in the present study (i.e., ID No. 1–3) did not deviate greatly from the distribution of the respective sites, thus their sizes were considered to be roughly typical. Both the height and DBH of the trees were not significantly different (α > 0.05) between the sites, although the completion year of coastal forest construction, including piling up of the soil and seedling planting were 1-year earlier in the US site than in the HM site ().
Figure 8. Frequency distribution of height and diameter at breast height (DBH) of trees at the present study sites. (a) US site, (b) HM site.
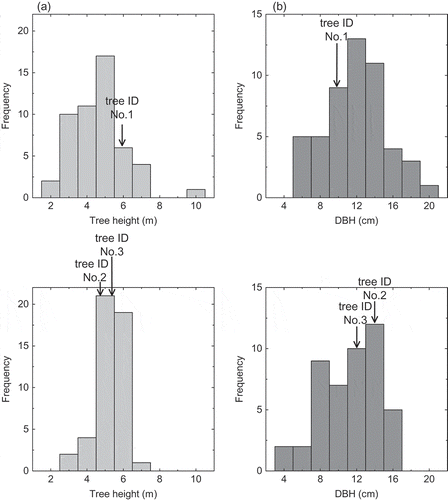
summarizes the biomass, size, and maximum vertical root depth of the three sampled trees that were chosen for the present study, and shows the roots dug up at the US and HM sites. The DBH and total biomass (TB) of sampled trees in the US site showed a little smaller value than those in the HM site (). However, the biomass of needles and roots, excluding aboveground woody tissues such as stems and branches, was generally smaller in the US site than that in the HM site. Therefore, the ratios of above- to belowground biomass (T/R) for all sampled trees were relatively wide, ranging from 2.1 to 4.8. According to the observation of the respective soil profiles, the distributions of very fine roots and fine roots were concentrated in the surface layers for all sites ( and ). Main thick and large roots of trees at both the US and HM sites spread and penetrated horizontally and vertically into the soil ( and ) as if to choose the ‘soft’ areas in the soil profiles, located in topsoil layers 20–40 cm deep and the vertical area at −100 cm deep directly under the sampling trees (). However, main roots hardly penetrated into the very hard and tightly packed gley layer in both sites that lay down at a depth of ≥1.2–1.4 m as a bottom layer (). Exceptionally, it was observed that the meager and small vertical fine roots penetrated 110 cm (for tree ID No. 1) and 123 and 145 cm (for tree ID No. 2 and 3) into the bottom layer, e.g., the quite ‘consolidated’ 2C and 3C/3G horizons in the US and HM sites, respectively ().
Figure 9. Photos of black pine roots grown at the US (ID No. 1: a, b) and HM (ID No. 2: c, d, ID No. 3: e, f) sites, in which row deep tillage treatment for growth bases was done. A puddle formed at the bottom of the survey pit in the US site (photo b) because of rain on the day before taking pictures.
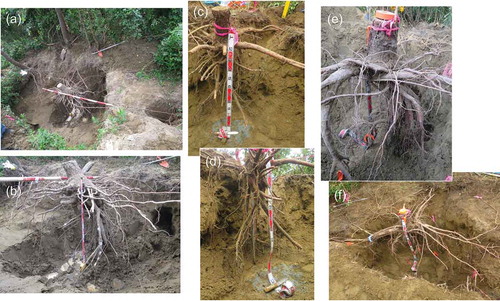
4. Discussion
4.1. Effects of deep tillage treatment on soil amendment for the constructed growth bases with piled up soil for coastal forests
It seems obvious in the fields of civil engineering and landscape engineering that man-made soil made of fine earth tends to aggregate and solidify if it is built up using heavy machinery to compact each layer (Hasegawa Citation1985; Horn Citation2015). Such compacted soil can be hardly to be rooted deeply (Nohara and Takahashi Citation2007; Murakami Citation2015). In the present study, the vertical areas with ‘soft’ and ‘hard/consolidated’ soil alternately appeared in the soil profiles of both the US and HM sites with row deep tillage (). The distribution of ‘hard/consolidated’ soils probably indicated the vestiges of soil compaction from the building up of the growth bases using heavy machinery and of the traces that remained untilled during the subsequent row deep tillage. Conversely, the areas of ‘soft’ soil showed the vestiges of row deep tillage and seedling planting holes. These traces of the soil compaction and its deep tillage were also observed in the growth bases of the coastal prevention forests in Sendai Bay, which were built up during the reforestation of the areas damaged by the mega-tsunami damaged areas (Ono et al. Citation2016). The distribution of ‘too soft’ soils might show the vestiges of the buried straw from when the growth bases were constructed (). Relatively dense, very fine, and fine roots were observed to be distributed in the areas of these ‘soft’ soils in the soil profiles in both sites (, ). In addition, thick and large roots of trees also penetrated vertically into such ‘soft’ areas (). However, no root penetration into ‘hard/consolidated’ areas was observed in the soil profiles, perhaps because of the compacted soil.
‘The right tree for the right land’ is still a principle of forestry in Japan (Morisada Citation1993), so most foresters in Japan have been generally dealing with natural soil so far. Understanding of the relationships between soil physical properties for man-made soil and root development as mentioned earlier is insufficient in the forestry field in Japan, even though it should be quite common in the field of agriculture, civil engineering, and landscape engineering. Thus, there have been always some reports that the park development and erosion control afforestation with the construction of the man-made growth bases has been often gone wrong (Morimoto Citation1985; Yabashi and Konko Citation1985; Yabashi and Konko Citation1987; Nohara and Takahashi Citation2007; Murakami Citation2015; Shinomiya et al. Citation2016). Therefore, it must be noted that the soil physical properties of growth bases for healthy plant growth should not be deteriorated by compaction using heavy machinery when afforesting the coastal forests.
Soil water content can heavily influence the results of penetrometer measurements, especially for fine soils, i.e., silt- and clay-rich soils (Ohnuki et al. Citation2007). Thus, penetrometer measurement requires parallel measurement of the soil water content. However, this rarely applies to sandy soil (Ohnuki et al. Citation2007). In the present study, most soil for each horizon in the profiles (<100–120 cm depth) was sandy at both sites (). The distribution of soil hardness in the US and HM sites had similar patterns (). Specially, ‘soft’ and ‘hard/consolidated’ areas were alternately observed in the profiles (), although soil water content at soil-core sampling (= volumetric percentage of the liquid phase) was quite different between the US and HM sites (), perhaps as a result of the difference of the sampling periods (). Thus, it can be concluded that the results of the penetrometer test in the present study were not influenced by the soil water content.
The construction firms that built up the plant growth bases in the restoration of mega-tsunami damaged coastal forest in Sendai Bay have often reported that the growth bases piled up using sandy soil had been gotten harder with the passage of time (i.e., Murakami Citation2015). This may in some way be true because the soil might have been compacted by watering. However, could it make the soils hard enough to inhibit the healthy growth of tree roots? The present study has shown that the effects of soil softening, i.e., reduction of soil hardness and the improvement of water permeability of the growth bases, have been maintained for about two decades after their construction, FY1995 and FY1996. This result implies that the influence of soil compaction, which could inhibit the root growth, by watering with the passage of time on soil physical properties of the growth bases would be limited during the afforestation of the coastal prevention forests in Chiba Prefecture.
4.2. Comprehensive judgment of the soil physical quality as plant growth bases of coastal prevention forests on the Kujukuri coastline
According to the current specification document on erosion and torrent control and afforestation by FAJ (Citation2016), the criteria of the quality management standards of the plant growth bases in the coastal prevention forests are as follows: water permeability is >30 mm h−1, ‘softness’ (soil hardness) index value is <23 mm of which measured by Yamanaka’s soil hardness tester, and/or >1.0 cm drop−1 of which measured by a dynamic cone penetrometer. If either the soil layer with ‘softness’ <1.0 cm drop−1 is consecutive 10 cm in thickness or that with ‘softness’ <0.7 cm drop−1 is consecutive 5 cm in thickness, the soils below the emergence depth of such a consolidated layer are decided as an unsuitable growth base. The criterion for ‘softness’ is almost the same as the criterion of the Research Committee of Japanese Institute of Landscape Architecture (Citation2000).
Regarding the vertical distribution of soil hardness in the filled soils of the US and HM sites, either the soil layers with ‘softness’ <0.7 cm drop−1 in which thickness is 5 cm or more, or those with ‘softness’ <1.0 cm drop−1 in which thickness is 10 cm or more were not generally stratified sequentially in the respective soil profiles (). This result therefore suggests that the ‘softness’ in the US and HM sites fulfilled the required conditions as growth bases for the afforestation of the coastal prevention forests. Of course, the criterion by Yamanaka’s soil hardness tester, i.e., index value of <23 mm, was also fulfilled throughout the depth of <120 cm in both profiles (). The water permeability of the soil profiles in the US and HM sites also fulfilled the criterion of FAJ; ranging from 40 to 480 mm h−1 (). Moreover, from the standpoint of healthy root development, it was observed that many thick and large vertical roots penetrated deep into soil layers of the constructed growth bases at the US and HM sites; maximum penetrated depths of the vertical roots ranged from 110 to 145 cm (, ). Of course, some of the horizontal roots also spread into the surface layers of the growth bases at both sites, but they were evenly distributed, generally. The size and biomass (DBH, TB, needles, and roots) of sampled trees in the US site were generally smaller than that in the HM site (). These measured items showed conventional values compared with previous reports that estimated black pine tree biomass (Kanagawa Pref Citation1967; Oda Citation1973; Oda Citation1977; Oda Citation1983; Karizumi Citation1990). Specifically, those items in the present study showed general allometric relationships between DBH and biomass (). The ratios of above- and belowground biomass for all sampled trees in the present study ranged from 2.1 to 4.8 (), also matching general ranges compared with previous data ().
Figure 10. Allometric relationships between diameter at breast height and dry mass of above- and belowground black pine biomass in Japan, and the top to root ratio. Open points show the data for trees grown in mountainous forests and closed points show the data for coastal forests. The star indicates the present study data. Lowercase numbers in the legends indicate the data sources of previous studies: 1Oda (Citation1973), 2Oda (Citation1977), 3Karizumi (Citation1990), 4Kanagawa Pref (Citation1967), 5Oda (Citation1983), 6Ono (unpublished data).
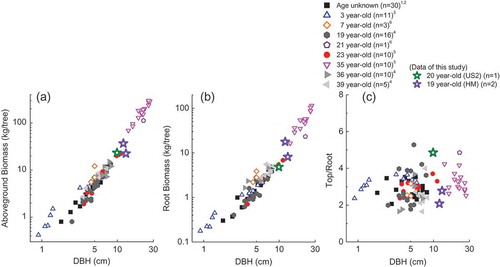
Based on the aforementioned observations, row deep tillage at the present study sites had a sufficient effect on establishing low-altitude coastal forests and on the ability of roots to deeply penetrate the soil two decades after construction of the growth bases. This agrees with a previous report that evaluated root growth in 10-year-old black pine trees at the US site and different neighboring sites of coastal forests in Kujukuri (Nohara and Takahashi Citation2007). Thus, the results of the present study imply that piling up soil with row deep tillage is an effective method to secure the space and the soil physical conditions for vertical root growth.
In the recovery of the coastal forests heavily damaged by the 2011 Tohoku mega-tsunami, soil preparation and soil amendment of the growth bases to restore coastal forests have been eagerly attempted by the FAJ and the various local public bodies (e.g., Iwate, Miyagi, and Fukushima Prefectures). If this procedure permeates through the coastal forest recovery projects in various places, it will be helpful for alleviating some problems that have been encountered on forest recovery sites, such as soil compaction, water stagnation, and hindrance of tree growth.
Acknowledgments
We are grateful to Mr Hiroyoshi Murai, the section chief of the Northern Forestry Administrative Office, the Department of Agriculture, Forestry and Fishery, Chiba Prefecture for their kind support and for arranging our field research. We sincerely thank Mr Shuzo Hasegawa of Geo-Green Tech. Inc. and Mr Keigo Inomata of Daitou Techmo Green Inc. for their help and encouragement. We also appreciate the kind support of the technical assistant Ms Keiko Sawai from Tohoku Research Center, the Forestry and Forest Products Research Institute, and the Tokyo University of Agriculture students, Mr. Yuta Hayashi and Mr. Shin Kumazaki, for assisting with our field surveys, sample preparations, and analyses.
Additional information
Funding
References
- Boone RD , Grigal DF , Sollins P , Ahrens RJ , Armstrong DE 1999: Soil sampling, preparation, archiving, and quality control. In Standard Soil Methods for Long-Term Ecological Research, Robertson GP , Coleman DC , Bledsoe CS , Sollins P. eds , pp. 3–28, Oxford Univ. Press, New York, USA.
- Chiba Prefecture 1997: Master Plan of Management for Coastal Protection Forests. pp. 330. Chiba Prefecture, Chiba, Japan.
- FAJ (Forest Agency of Japan) 2016: Standard Specification Documents on Erosion and Torrent Control and Afforestation. pp. 156, FAJ, Tokyo. http://www.rinya.maff.go.jp/j/sekou/gijutu/pdf/ts-tokekomi-h28s1.pdf.
- FAO (Food and Agriculture Organization of the United Nations) 2006: Guidelines for Soil Description. 4th edition. pp. 97, Rome, Italy.
- FAO (Food and Agriculture Organization of the United Nations) 2015: World Reference Base for Soil Resources 2014. pp. 192. World Soil Resources Reports 106, FAO, Rome, Italy.
- Forest Research Center of Civil Engineering Facilities 1990: Contract Research Report for Working-Out of the Management Master Plan in Chiba Prefectural Coastal Prevention Forests, pp. 169. Forest Research Center of Civil Engineering Facilities, Tokyo, Japan. (in Japanese).
- Forest Soil Division 1976: Classification of forest soils in Japan 1975. Bull. Gov. Forest Exp. Stn . 280, 1–28. (in Japanese with English summary).
- GSI (Geospatial Information Authority of Japan) 2017: GSI web site. http://mapps.gsi.go.jp/maplibSearch.do#1
- Hasegawa S 1985: The management method of growth bases in soil amendment by using a dynamic corn penetrometer. Proceedings of the 5th meeting for Japan Highway Landscape Association. p10–11. (in Japanese)
- Hasegawa S 2008: The estimating method of tree root distribution. Various subjects on slope stability and root system. J. Jpn. Soc. Reveget. Tech . 31, 346–351. (in Japapnese). doi:10.7211/jjsrt.31.346
- Horn R 2015: 3.1 Soil compaction and consequences of soil deformation on changes in soil functions. In Task Force: soil Matters. –Solutions under Foot–”, Nortcliff S. Ed., pp. 28–32. Catena Verlag GMBH, Reiskirchen, Germany.
- Kanagawa Pref 1967: Research Report of Coastal Disaster Prevention Forest in Shonan Coastline. pp. 114. Sediment Control Division, Public Works Section, Kanagawa Prefecture, Kanagawa. (in Japanese).
- Karizumi N 1990: Reduction of inorganic matters to soil and formation of porosity resulting from root system. Bull. For. For. Prod. Res. Inst. 357, 1–49.
- Karizumi N 2010: The Latest Illustrations of Tree Roots. pp. 188–230. Seibundo Shinkosha, Tokyo, Japan.
- Kawada H , Kojima T 1976: Environmental Measurement Methods IV. – forest Soils -. pp. 210. Kyoritsu Shuppan Co. Ltd., Tokyo, Japan. (in Japanese)
- Marshall TJ , Holmes JW , Rose CW 1999: Soil Physics. 3rd edition. pp. 453. Cambridge University Press, Cambridge, UK.
- Miyajima S , Uoi N , Murata T , Takeda M , Morishima W , Watanabe M 2015: Effect of structural modification of heat transfer through man-made soils in urban green areas. Soil Sci. Plant Nutr . 61, 70–87. doi:10.1080/00380768.2015.1051929
- Morimoto Y 1985: Study on the growth medium in tracts of green land. Kyoto University Research Information Repository. http://dx.doi.org/10.14989/doctor.r5589.
- Morisada K 1993: “The Right Tree for the Right Land” and the Fertility of Forest Soil, Shikoku Information 10, Shikoku Research Center, FFPRI, Kochi, Japan. (in Japanese)
- Murai H , Ishikawa M , Endo J , Tadaki Y 1992: The Coastal Forests in Japan. pp. 513. Soft Science Inc., Tokyo, Japan.
- Murakami T 2015: Reconstruction works for the coastal forests using embankment and the work process to the planting seedlings. J. Jpn. Soc. Reveget. Tech . 41, 341–343. (in Japanese). doi:10.7211/jjsrt.41.341
- Nakagawa S 1994: A Pictorial Book of Conifers. pp. 188. Hoikusha Publishers Co.,Ltd., Osaka, Japan. (in Japanese)
- Nohara S , Takahashi T 2007: The effect of landfill on damp lowlands of coastal sand dunes: growth of the roots of 10 year-old black pines (Pinus thunbergii). Bull. Chiba For. Res. Cent . 2, 1–6. in Japanese.
- Oda 1977: The tree growth and stand density in the coastal black pine forests (I). Sand Dune Res . 23, 14–22.
- Oda 1983: The productivity of the coastal black pine forests. Rev. For. Culture . 4, 87–90.
- Oda T 1973: Study on the trees that grow bent in one direction due to strong sea winds in the coastal forests. Bull. Chiba Pref. Forest. Ex. Stn . 8, 55–59.
- Oda T 2000: Minimum filling thickness based on characteristics of distribution of Pinus thunbergii root systems in damp, lowlands sand dunes of the coastal forests. Sand Dune Res . 47, 102–110. in Japanese.
- Oda T 2001: Study on the reaction of planted tree root systems to water-logging and its application to developing forests in damp lowlands of coastal sand dunes. Spec. Bull. Chiba For. Res. Cent . 3, 1–78. (in Japanese with English summary).
- Ohnuki Y , Kimhean C , Shinomiya Y , Sor S , Toriyama J , Ohta S 2007: Apparent change in soil depth and soil hardness in forest areas in Kampong Thom Province, Cambodia. In Forest Environments in the Mekong River Basin, Sawada H , Araki M , Chappell NA , LaFrankie JV , Shimizu A . eds., pp. 263–272. Springer, Tokyo, Japan.
- Ono K , Imaya A 2017: 5. Soils filling in swamplands behind coastal sand dunes to prevent the coastal disaster. In Anthropic Soils in Asia, Watanabe M , Kawahigashi M . eds., pp. 45–55. Tokyo Metropolitan Univ., Tokyo, Japan.
- Ono K , Imaya A , Takanashi K , Sakamoto T 2016: Evaluation of the berms built on the Restoration of the Mega-Tsunami-Damaged Coastal Forests. -Comparison with the effects of soil-scratching as a soil physical correction method among the various types of machinery. Bull. For. For. Prod. Res. Inst . 15, 65–78. in Japanese with English abstract.
- OriginLab Corp 2010: OriginPro 8.5.0J SR1, Originlab Corporation, Massachusetts, USA.
- Research Committee of Japanese Institute of Landscape Architecture 2000: Groundmaintenance manual in landscape planting. J. Jpn. Inst. Landscape Architect . 63, 224–241. in Japanese.
- SAS Institute 2010: JMP Statistics and Graphic Guide, Version 9.0.0. SAS Institute Inc., Cary, USA.
- Shinomiya Y , Imaya A , Takanashi K , Sakamoto T 2016: The physical property of embankment with a rain pool: A case study of embankment built in the early stage of the Coastal Forest Restoration Project after tsunami. Bull. For. For. Prod. Res. Inst . 15, 151–159. in Japanese with English abstract.
- Yabashi S , Konko T 1985: Studies on the improvement of planting land consist of problem soils. I. Physical properties of Inagi sand soil. Tech. Bull. Fac. Hort. Chiba Univ . 35, 43–46. in Japanese with English abstract.
- Yabashi S , Konko T 1987: Studies on the Improvement of planting land consisting of problem oils. II. Effect pf mixing with Inagi sand soil and Kanto Loam on compaction. Tech. Bull. Fac. Hort. Chiba Univ . 39, 69–76. in Japanese with English abstract.