ABSTRACT
We examined the effect of two types of iron (Fe) material produced by the casting industry (spent steel shot [SSS] and residual iron material from steel shot production) on the mobility of arsenic (As) and cadmium (Cd) in soils. We also examined the uptake of these elements by rice plants (Oryza Sativa L.) under continuously flooded (CF) and water-saving (WS) cultivation. The application of both Fe materials (at 10 and 30 t ha‒1) strictly limited As mobilization in soils under CF cultivation. As a result, As uptake by rice plants declined, along with the total and inorganic As (iAs) concentration in rice grains. In comparison, As immobilization caused by the application of Fe material was less clear under WS cultivation. The rate of Fe material application was negatively correlated with As uptake by rice plants. It was also negatively correlated with total and iAs concentration in rice grains under both water management practices. The combination of applying Fe materials and WS cultivation decreased iAs concentration in rice grains to approximately one-fifth of that in rice grains produced from plants grown on soils without Fe material application under CF cultivation. CF cultivation strictly decreased dissolved Cd in soils, as well as Cd in rice grains with and without Fe material application. The application of Fe materials decreased Cd mobility and, hence, Cd uptake in rice plants, ultimately reducing the accumulation of Cd in rice grains under WS cultivation. Residual Fe material had a statistically greater effect at attenuating Cd accumulation in rice grains than SSS. The present study demonstrated the potential of combining by-product Fe material application and water management practices to attenuate iAs and/or Cd concentrations in rice grains. Practical countermeasures should be carefully adopted that consider the existing risks of iAs and Cd on each paddy field, and the combined effect of Fe material application and water management practices.
1. Introduction
Arsenic (As) and cadmium (Cd) are well-known hazardous elements that are ubiquitously present in soil environments. Long-term exposure to inorganic As (iAs) causes some kinds of cancers; therefore, the International Agency for Research on Cancer has classified iAs compounds as carcinogenic to humans (WHO Citation2016). Cd, which is causes Itai-Itai disease, induces kidney failure and cancer (Huff et al. Citation2007). Rice consumption is one of the major sources of both iAs and Cd in Asian countries (Shimbo et al. Citation2001; Li et al. Citation2011; Oguri et al. Citation2014). In recent years, the Codex Alimentarius Commission had adopted maximum levels (maximum permitted concentrations) for iAs in polished rice and husked rice as 0.2 and 0.35 mg kg−1, respectively (Codex Citation2014; Citation2016), whereas the maximum level for Cd in polished rice was adopted as 0.4 mg kg−1 in 2006 (Codex Citation2006). The concentrations of iAs and Cd in rice grains should be carefully controlled to decrease the risk to human health and the agricultural economy.
Flooded cultivations attenuate Cd concentration in rice grains, whereas As concentration in rice grains is markedly increased by As dissolution from soils under such anaerobic soil conditions (Arao et al. Citation2009). Thus, there is an obvious trade-off relationship between mitigation measures for As and Cd in rice grains, in terms of water management for cultivation. Ishikawa et al. (Citation2016) reported that the application of a low-Cd rice cultivar (‘Koshihikari Kan No.1’) with a water-saving (WS) cultivation keeps both As and Cd in rice grains at low levels, even though WS conditions had an adverse effect on grain yield and quality. Furthermore, we demonstrated that the combined application of commercial iron (Fe) materials and a flooded cultivation simultaneously limited iAs and Cd in rice grains, without any significant decrease in grain yield and quality (Makino et al. Citation2016). However, the high cost of the commercial Fe materials might limit their future use in agricultural practices.
To decrease the cost of Fe materials, we previously examined an Fe-type water treatment residue, and showed its ability to attenuate As in rice grains under flooded conditions (Suda et al. Citation2016). In addition, large quantities of inexpensive Fe materials could be obtained from the casting industry. Spent steel shot (SSS) is generated after the daily use of steel shots for removing casting sand. Furthermore, large amount of residual Fe material (RIM) is also produced in the process of steel shot making. Our previous work demonstrated that these by-product Fe materials are mainly composed of zero-valent Fe [Fe(0)], wüstite, and magnetite/maghemite, and they significantly immobilize soil As during long-term flooding (Suda et al. Citationin press). These major components of Fe materials might also immobilize Cd via sorption and insoluble Cd sulfide formation (Chowdhury et al. Citation2013; Hashimoto and Yamaguchi Citation2013). For example, Ok et al. (Citation2011) demonstrated that Cd concentration in rice grains grown on polluted soils in Korea are reduced by 42% following the application of Fe(0) at approximately 2 wt%. Thus, the application of SSS and RIM, which are composed of Fe(0) and Fe oxides, is expected to alleviate the uptake of both As and Cd by rice plants at low cost.
In most studies focusing on the attenuation of As uptake by rice plants using Fe material application, rice plants have been grown on flooded soils over most of the cultivation period (e.g., Ultra et al. Citation2009; Farrow et al. Citation2015; Matsumoto et al. Citation2015), with a few exceptions (Honma et al. Citation2016a). Because Cd mobility in flooded soils is strictly limited, evaluating the effect of Fe material application on Cd uptake is difficult under such conditions. In addition, the stability and ability of Fe minerals in Fe materials to immobilize As and Cd probably changes in relation to the surrounding environment, such as pH and dissolved oxygen concentration (Mishra and Farrell Citation2005; Chowdhury and Yanful Citation2013).
Therefore, the main objective of the present study was to determine how by-product Fe materials from the casting industry combined with different water management practices affect the dissolution of As and Cd in soils, the uptake of As and Cd, and concentrations of As (especially iAs) and Cd in rice grains. Our results are expected to contribute novel information toward improving the management of hazardous materials in agricultural soils.
2. Materials and methods
2.1. Pot experiment
Soil was collected from the plow layer of a paddy field (Fluvisols) in Japan for a pot experiment. The soil had a pH of 5.88, cation exchange capacity of 16.0 cmol kg−1, and contained 21 g kg−1 total carbon, 1.74 g kg−1 total nitrogen, 9.78 g kg−1 oxalate-extractable Fe, 14.4 g kg−1 dithionite-citrate-extractable Fe, 277 g kg−1 clay, 2.48 mg kg−1 1 mol L‒1 hydrochloric acid-extractable As, and 0.306 mg kg−1 of 0.1 mol L‒1 hydrochloric acid-extractable Cd. According to the Agricultural Land-Soil Pollution Prevention Law in Japan, the soil is not defined as As-polluted soil (1 mol L−1 hydrochloric acid-extractable As >15 mg kg−1). Also, the sampling field is located in a non-Cd-polluted area, which is determined by Cd concentration in rice grains.
Two types of by-product Fe materials were tested for their ability to attenuate the uptake of As and Cd by rice plants, and their concentration in rice grains. One of the Fe materials was SSS, which was collected from some foundries and was screened magnetically. The other Fe material was RIM, which is generated through steel shot making. Details on the properties of the Fe materials (<1 mm) were reported previously (Suda et al. Citationin press). In brief, SSS was composed of Fe(0) and a small amount of quartz, whereas RIM contained magnetite and/or maghemite (magnetite/maghemite), wüstite, and a small amount of Fe(0) (steel shot-like spherical particles). RIM showed a finer particle size distribution compared to SSS. Unlike RIM, SSS contained minor amounts of particles larger than 1 mm (21.9 wt%), therefore the concentrations of elements in SSS were slightly different from those reported in the literature. SSS and RIM contained 876 and 642 g kg‒1 Fe, 49.7 and 57.4 g kg‒1 of silicon (Si), and 6.61 and 49.3 g kg‒1 of manganese (Mn), respectively.
A pot experiment was conducted in a greenhouse under natural sunlight from May to September 2016. Two-point-two kilograms (oven-dry basis) of raw soil (<8 mm) was thoroughly mixed together with a compound fertilizer equivalent to 0.2 g nitrogen, 0.087 g phosphorus (P), and 0.17 g potassium, and 0, 20, or 60 g of either SSS or RIM. The added amounts of Fe materials were equivalent to the application rates of 0, 10, and 30 t ha−1, respectively. Tap water was added to each pot with soil (3 May 2016). Three rice plant seedlings (Oryza sativa L. cv. Koshihikari) were transplanted to each pot (13 May 2016). The rice plants were grown under each water management practice described below during the cultivation period. The rice plant was cut at the base of the stem after its seeds matured (6 September 2016). The harvested rice plant was air-dried, and then separated into straws (stems and leaves), husks, and grains. Unfilled rice grains were screened with a 1.85-mm-mesh sieve.
We carried out two water management practices; namely, continuously flooded (CF) and WS cultivation. For CF cultivation (conducted in triplicate), the soil in a pot was flooded by water throughout the growing period (until 2 September 2016). For WS cultivation (conducted in quadruplicate), the soil in a pot was kept flooded during the early part of cultivation, and was then intermittently irrigated from approximately 2 weeks before heading to the end of the growing period (until 2 September 2016). During the period of flooded cultivation, the irrigation depth was kept between 2 and 5 cm. During the period of intermittent irrigation, irrigation water was not supplied until the soil surface had visually dried out.
2.2. Sampling and analysis of the soil solution
On days 25, 40, 52, 69, 83, and 97 after transplanting, approximately 10 mL of the soil solution was collected two times at a depth of 5‒10 cm with a soil solution sampler (DIK-305A; Daiki Rika Kogyo, Saitama, Japan) connected with a syringe. Sampling was carried out approximately 24 h after the pot was last irrigated. The pH of the first soil solution was measured as soon as possible. The second soil solution was mixed with 1 mL of 10% nitric acid to dissolve the Fe oxides that were generated when the solution was sampled and to prevent the Fe precipitating during storage. The acidified soil solution was left until the precipitated Fe oxides completely disappeared, and was then filtrated with a 0.2 μm filter and diluted with ultrapure water. Dissolved As and Cd in the diluted solution were measured by inductively coupled plasma mass spectrometry (ICP-MS: Elan DRCe; PerkinElmer, Waltham, MA, USA). The concentrations of Fe, Si, and Mn were determined by inductively coupled plasma optical emission spectrometry (ICP-OES: Agilent 700 Series; Agilent Technologies, Santa Clara, CA, USA).
Soil Eh was measured at a soil depth of 7 cm on days 11, 17, 24, 31, 39, 52, 60, 69, 78, 83, 89, and 97 after transplanting. A platinum electrode and a silver–silver chloride reference electrode (Type 4400, DKK-TOA Corporation, Tokyo, Japan) were used for the measurement.
2.3. Analysis of harvested rice plants
The harvested rice straws, husks, and grains (not polished) were dried at 75°C. Only the straw samples were crushed coarsely in advance. All of the different types of plant samples were ground in a ball mill to a fine powder. The powdered samples were used for various chemical analyses. Rice powder was digested with nitric acid on a heating block, and the digested solution was used for As speciation with a high-performance liquid chromatography/ICP-MS (HPLC: Flexar, PerkinElmer; ICP-MS: Nexion 300X, PerkinElmer). The rice powder was also digested with nitric acid and hydrogen peroxide to quantify total As (tAs) and other elements. For the same purpose, straw and husk powders were digested with nitric acid, hydrogen peroxide, and hydrogen fluoride. Although straw and husk powders were incompletely digested, the obtained value was referred to as the total amount of each element for simplicity. Silicon in straw and husk powder was extracted using a mixture of 1.5 mol L−1 hydrofluoric acid and 0.6 mol L−1 hydrogen chloride, as described in Saito et al. (Citation2005). The element concentrations in the digested solution were measured with ICP-MS (Elan DRCe or Nexion) and ICP-OES (Agilent 700 Series). Details on the procedures and conditions for the chemical analyses of the plant samples are described in our previous report (Suda et al. Citation2016).
2.4. Statistical analysis
Simple linear regression and Pearson’s correlation coefficient analyses were carried out to examine the effect of Fe material application rate under each water management strategy, and, if necessary, the differences between the slopes of the regression lines were assessed by testing the F-value. The effect of water management alone was evaluated by analysis of variance (ANOVA), using data obtained from the cultivations without Fe material application (control). The concentrations of iAs, tAs, and Cd in rice grains were expressed as ordinary water content (15%); however, the data of other plant samples were shown as an oven-dried (135°C) basis. When a dataset contained values below the limit of determination (LOD) or limit of quantitation (LOQ) of measurements, only its average was calculated using half the value of LOD or LOQ, and further statistical analysis was not performed. The statistical analyses were carried out using Microsoft Excel and R software.
3. Results and discussion
3.1. Grain yield and biomass production
For both water management treatments, simple linear regression analyses showed no significant negative relationships between grain yield or shoot biomass and the SSS application rate up to 30 t ha‒1 (). In comparison, the increase in RIM application rate statistically lowered grain yield. WS cultivation tended to decreased grain yield and shoot biomass (Fig. S1). The uptake of P and Si by rice plants was not negatively affected by increasing the application rate of SSS and RIM (Table S1). Further studies at the field scale are needed to clarify how Fe material applications affect rice growth and grain yield under paddy field conditions.
Table 1. Simple linear regression analyses for grain yield and shoot biomass in relation to iron material application rates.
3.2. Soil solution pH and soil eh
The soil solution pH of Fe material-applied soils tended to be higher than that of soils without Fe material application (control soils; ,). However, the differences among treatments decreased with time, and converged at a pH of approximately 6.5. The soil solution pH remained around 6.5 until the final sampling event under CF cultivation. Nozoe et al. (Citation2001) demonstrated a similar trend of pH change during the 45-day flooded incubation of soils mixed with powdered Fe(0). The increases of pH by Fe(0) application can be explained by the following equations:
Additionally, surface hydroxyl groups of Fe oxides in RIM consumed proton from soil solution, because they would have higher point of zero charges (e.g., around 6.5 for maghemite; Kosmulski Citation2011) than pH of the control soils.
Figure 1. Time courses of soil solution pH and soil Eh during the cultivation period. (a) and (c) are under CF cultivation and (b) and (d) are under WS cultivation. The plot and error bar represent the average and standard deviation, respectively. SSS and RIM denote SSS and residual iron material, respectively. The double-headed arrow indicates intermittent irrigation period.
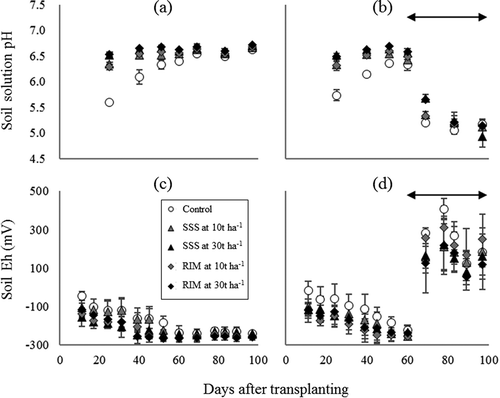
In comparison, under WS cultivation, the soil solution pH drastically dropped as the soil dried out during the intermittent irrigation period. The decrease of pH can be explained by the oxidation of Fe(II), which is expressed by the following equation:
The Eh of soils applied with Fe materials remained at lower levels than that of control soils in the early period of cultivation (,). The Eh of all soils gradually decreased and converged at around ‒230 mV. Then, the soil Eh value was maintained until the final measuring event under CF cultivation. In comparison, under WS cultivation, soil Eh drastically rose to more than +80 mV as the soil dried out during the intermittent irrigation period.
3.3. As in the soil solution and rice plants
3.3.1. Soil solution
Dissolved As concentration in control soils under CF cultivation gradually increased, peaking at 120 μg L−1 on the final day of sampling (day 97) (). As dissolution from soils is caused by the reductive dissolution of As-bearing Fe oxides and the transformation of As(V) to As(III), which progresses under flooded conditions. In contrast, dissolved As concentration in control soils under WS cultivation sharply dropped with soil-drying, and became less than 2 μg L−1 in the later sampling days (). The decrease of dissolved As by soil-drying is explained by co-precipitation (including sorption) of As with newly formed Fe oxides and the oxidative transformation of As(III) to As(V). This reaction is accelerated when air penetrates soils when soil-drying occurs during the intermittent irrigation period.
Figure 2. Time courses of the concentrations of dissolved As and Cd in soil solution during the cultivation period. (a) and (c) are under CF cultivation and (b) and (d) are under WS cultivation. SSS and RIM denote SSS and residual iron material, respectively. The plot and error bar represent average and standard deviation, respectively. The double-headed arrow indicates intermittent irrigation period. n.d. means dissolved Cd in soil solution was not detected (< 4.05 × 10–3 μg L−1).
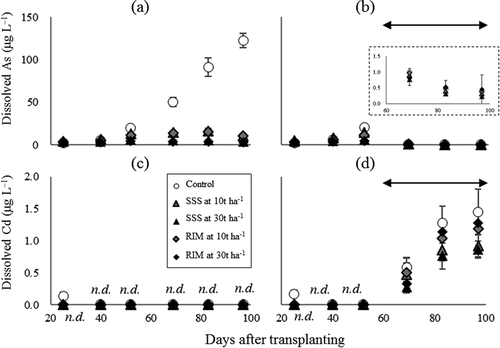
The application of Fe materials strictly limited the mobilization of As in soils under CF cultivation (). The maximum dissolved As concentration in control soils was 50 times greater than that on the first sampling day. In comparison, the maximum dissolved As concentration in soils with Fe materials was less than five times greater than that of the control soils on the first sampling day. On the final sampling day, the dissolved As concentration in soils with Fe materials at the rate of 10 and 30 t ha‒1 was less than 10% and 4%, respectively, than that of control soils under CF cultivation. In comparison, the effect of Fe material application was not clear on the sampling days during the intermittent irrigation period under WS cultivation. This result was probably obtained because the flooded duration before sampling (24 h) followed by soil-drying was not long enough for the considerable re-mobilization of As in the soil solution (dissolved As levels were less than 2 μg L‒1). Therefore, the data obtained on the final three sampling days might not exactly reflect actual As availability to rice plants during the intermittent irrigation period.
Evaluating the extent to which potential mechanisms contribute toward immobilizing As by applying Fe contribute seems to be very difficult. Fe oxides have high capabilities for removing As from solution. The application of Fe materials increases Fe oxides in the applied soils. In particular, RIM directly supplies Fe oxides to the soils, because certain types of Fe oxides (magnetite/maghemite and wüstite) are its major components. Furthermore, the oxidation of Fe(II) and Fe(0) in Fe materials could provide an indirect pathway for supplying Fe oxide from Fe materials. The increase of dissolved Fe by Fe material application (Fig. S2a‒b) probably occurs because of the dissolution of Fe oxides and Fe(0). Because dissolved Fe, mainly Fe(II), is oxidized under aerobic (i.e., enough dissolved oxygen) conditions, soil-drying during the intermittent period should be an inevitable trigger for the formation of flesh Fe oxides and consequent As immobilization. The Fe(II) oxidation should also occur at locally oxidized areas, even in flooded soils, such as around the root surface of rice plants and the oxidized layer of the soil surface (Kirk and Bajita Citation1995; Ratering and Schnell Citation2000). Furthermore, Fe(0), which is a major and minor component of SSS and RIM, respectively, might immobilize As via another mechanism. Our previous work showed that Fe(0) strictly limits As mobilization in anaerobic soils and enhances the formation of orpiment and/or arsenopyrite-like compounds (Suda et al. Citationin press). This phenomenon might occur and contribute toward immobilizing As by SSS and RIM, which contain Fe(0), under flooded conditions (i.e., entire period of CF cultivation and the early part of WS cultivation).
3.4. Rice plants
–f shows the relationship between the concentrations of each element (iAs, tAs, and Cd) and the application rate of Fe materials. The maximum iAs concentration of rice grains under CF and WS cultivation (0.354 and 0.159 mg kg‒1, respectively) was obtained from rice grains grown on control soils. The ANOVA test demonstrated that the two values were significantly different (p < 0.001), indicating that WS cultivation decreases iAs concentration in rice grains on control soils.
Figure 3. Simple linear regressions of the concentration of inorganic arsenic (iAs), total arsenic (tAs) and Cd in grains of rice grown under CF [(a), (c), (e)] and WS [(b), (d), (f)] cultivation in relation to iron (Fe) material application rates. Plot colors of gray and white indicate CF and WS cultivation, respectively. SSS and RIM denote SSS and residual iron material, respectively. n.d. and tr. indicate that the Cd concentration in grains range from 0 to 7.57 × 10–3 and 7.57 × 10–3 to 2.53 × 10–2 mg kg−1, respectively.
![Figure 3. Simple linear regressions of the concentration of inorganic arsenic (iAs), total arsenic (tAs) and Cd in grains of rice grown under CF [(a), (c), (e)] and WS [(b), (d), (f)] cultivation in relation to iron (Fe) material application rates. Plot colors of gray and white indicate CF and WS cultivation, respectively. SSS and RIM denote SSS and residual iron material, respectively. n.d. and tr. indicate that the Cd concentration in grains range from 0 to 7.57 × 10–3 and 7.57 × 10–3 to 2.53 × 10–2 mg kg−1, respectively.](/cms/asset/d142a3af-2244-41df-9498-363c9274d96e/tssp_a_1450077_f0003_b.gif)
A regression analysis clearly demonstrated that Fe materials attenuated iAs in rice grains in response to their application rate (R2 = 0.881 for SSS under CF cultivation, 0.873 for RIM under CF cultivation, 0.937 for SSS under WS cultivation, 0.961 for RIM under WS cultivation; p < 0.001). The slope of the regression line for each Fe material under CF cultivation was statistically greater than that under WS cultivation (p < 0.001), indicating that Fe materials work more effectively under CF cultivation than under WS cultivation. This difference might arise from the difference in soil physical-chemical conditions between CF and WS cultivation, and/or from the difference in As levels in rice grains, as pointed out by Makino et al. (Citation2016). In comparison, the slope of the regression line of two Fe materials under the same water management practice was not statistically different (p > 0.05). Thus, the two Fe materials might have comparable ability for decreasing iAs in grains, even though they have different Fe contents and mineralogical properties. The comparable ability of RIM to that of SSS was probably attributable to the finer particle size of RIM (Suda et al. Citationin press).
The results of the statistical analyses for tAs in grains and As uptake in the shoot were similar to those obtained for iAs in grains (Table S2). In brief, tAs in rice grains and As uptake in the shoot linearly decreased with increasing Fe material application rates. The effect of Fe material application under CF cultivation was stronger than that under WS cultivation. The two Fe materials had comparable ability at attenuating As under both water management practices.
Under CF cultivation, strong positive correlations were obtained between the logarithmic concentration of dissolved As in soil solution (average of three sampling days around heading) and the concentrations of iAs and tAs in rice grains (,; r = 0.977 for iAs;; r = 0.971 for tAs; p < 0.001). Thus, the immobilization of soil As by Fe material application attenuated the As load in rice grains grown on soils under CF cultivation. For WS cultivation, this relationship less clear than under CF cultivation (a,b; r = 0.430, p = 0.058 for iAs; r = 0.455, p < 0.05 for tAs). These relatively low correlation coefficients probably reflect the fact that the measured dissolved As does not represent actual dissolved As levels during the intermittent irrigation period, rather than dissolved As being a weak factor for determining As uptake under WS cultivation. Alternatively, the inhibition of As(III) absorption by dissolved Si is a known mechanism for the attenuation of As uptake by rice plants (Guo et al. Citation2005; Li et al. Citation2009). More or less, the competition of absorption between As and Si contributes toward alleviating As uptake by rice plants, because the application of Fe materials tends to increase dissolved Si in the soil (Fig. S2c‒d).
Figure 4. Relationships between the concentrations of dissolved As and Cd and the logarithmic concentrations of iAs (a), tAs (b), and Cd (c) in rice grains. Only for Cd, data under CF cultivation was not shown because most of the concentration of dissolved Cd in the soils and Cd in rice grains were less than LOD or LOQ. Circle, triangle, and square indicate control, application of SSS, and application of residual iron material, respectively. Plot colors of gray and white indicate CF and WS cultivation, respectively.
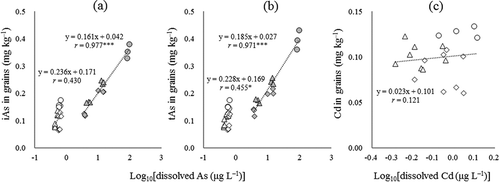
3.5. Cd in the soil solution and rice plants
3.6. Soil solution
The level of dissolved Cd in control soils under CF cultivation remained very low and was not detectable, except on the first sampling day (). In contrast, dissolved Cd in control soils under WS cultivation rose sharply following soil-drying, which enhanced the aerobic conditions of the soil (). These observations were consistent with well-established relationships between soil redox potential and Cd mobility in soils (e.g., Huang et al. Citation2012). Because pH is a prime factor to determine Cd solubility in soils (Chuan et al. Citation1996), the decrease in soil pH causes Cd mobilization when soil dries. The oxidative dissolution of Cd sulfide, which would occur during the CF period, might also explain the increase of dissolved Cd after soil-drying (Furuya et al. Citation2016).
The application of Fe materials tended to decrease dissolved Cd in soil solution after the start of intermittent irrigation period of WS cultivation. For the flooded period during WS cultivation and whole period during CF cultivation, Cd solubilization was highly limited, making discussion difficult. For the intermittent irrigation period during WS cultivation, the higher soil solution pH () might cause the lower dissolved Cd in soils with Fe materials than that in control soils. Furthermore, the direct and indirect increase of Fe oxides along with Fe material application might immobilize Cd, even though the affinity of Cd to Fe oxides is relatively low when compared to other heavy metals (Kinniburgh et al. Citation1976). Hashimoto and Yamaguchi (Citation2013) reported that Fe(0) enhances the formation of Cd sulfide, which is an insoluble compound, and decreases Cd in soil solution. Furuya et al. (Citation2016) showed that Cd sulfide forms under anaerobic conditions, and remains, to some extent, even under subsequent aerobic conditions, for more than 20 days. Therefore, the acceleration of Cd sulfide formation, which is promoted by Fe(0) in Fe materials, might be a possible mechanism for the immobilization of Cd in soils ().
3.7. Rice plants
,F shows the effect of Fe material application on Cd concentration in rice grains. The highest Cd concentration of 0.127 mg kg‒1 was obtained from rice grains grown on control soils under WS cultivation. In comparison, rice grains grown on control soils under CF cultivation had Cd less than LOQ (2.20 × 10‒2 mg kg‒1).
The effect of Fe material application on Cd in rice grains grown under CF cultivation was unclear, because the concentration of Cd in rice grains was less than LOQ (2.20 × 10‒2 mg kg‒1), regardless of Fe material input under CF cultivation (). In comparison, under WS cultivation, an increase in the application rate of Fe materials caused a linear decrease of Cd in rice grains (; R2 = 0.775 for SSS and 0.953 for RIM; p < 0.001). The slope of the regression line for RIM under WS cultivation was statistically greater than that for SSS (p < 0.01). Thus, the application of RIM more efficiently decreased Cd in rice grains than SSS.
Table S2 shows that Fe material application caused a linear decrease in total Cd uptake in shoots grown on soils under WS cultivation in response to their application rate (p < 0.001). The slope of the regression line for RIM was distinctly greater than that of SSS under WS cultivation, but was not significant at the 0.05 significance level (p = 0.059). For CF cultivation, the total uptake of Cd in the shoot could not be determined because Cd concentration in rice grains was too low to quantify. However, total Cd uptake seemed to decrease in response to Fe material application rates, judging from the sum of straw Cd, husk Cd, and possible range of Cd in grains (Table S2).
Although Honma et al. (Citation2016b) reported a linear relationship between dissolved Cd in soil solution and Cd in grains, we found no linear or logarithmic relationship between these two parameters in the present study (). Of note, at the equivalent application levels, RIM attenuated Cd in rice grains more than SSS (). This result was unexpected because SSS lowered dissolved Cd in soils more than RIM under WS cultivation (). Differences in the ability of the two Fe materials to supply Mn to the soil solution (Fig. S2f) might explain this inconsistency, because dissolved Mn inhibits Cd absorption by rice roots (Yoshikawa et al. Citation1986).
3.8. Practical application
In the present study, we clarified the combined effect of Fe material application and water management practices on iAs, tAs, and Cd concentrations in rice grains. summarizes effect of water management practices with and without applying Fe materials. The application of Fe materials decreased both Cd and, especially, iAs accumulation in rice grains in relation to their application rate. As expected from previous studies (Arao et al. Citation2009; Hu et al. Citation2013), CF cultivation kept Cd in rice grains at very low levels, whereas WS cultivation attenuated the iAs load in rice grains. Focusing on iAs only, the combination of Fe material application and WS cultivation seemed to be the most powerful countermeasure. In the present study, iAs concentration in rice grains grown on Fe material-applied soils (at 30 t ha‒1) under WS cultivation was approximately only 20% of that grown on control soils under CF cultivation. However, Cd in rice grains under WS cultivation was substantially greater than that under CF cultivation. Moreover, grain yield under WS cultivation tended to be less than that under CF cultivation (Fig. S1). Therefore, the application of this combination might be limited because of these adverse effects. Alternatively, the combination of Fe material application and CF cultivation might be a better choice in many cases, because it simultaneously achieves relatively low iAs and extremely low-Cd in rice grains, and probably maintains grain yields. However, for all the countermeasures involving Fe material application, cost-cutting of Fe material is essential. Therefore, further studies focusing on inexpensive by-product Fe materials, such as SSS and RIM, are needed to establish practical countermeasures for alleviating iAs and Cd concentrations in rice grains.
Figure 5. Relationship between the relative concentrations of iAs and Cd in grains grown under each culture condition. CF and WS mean CF and WS, respectively. The concentrations of iAs and Cd in grains grown on soils under CF and WS cultivation, respectively, were taken as 100%. circle, triangle, and square indicate control, application of SSS, and application of residual iron material, respectively. Plot colors of white, gray, and black indicate an iron material application rate of 0, 10, and 30 t ha−1, respectively.
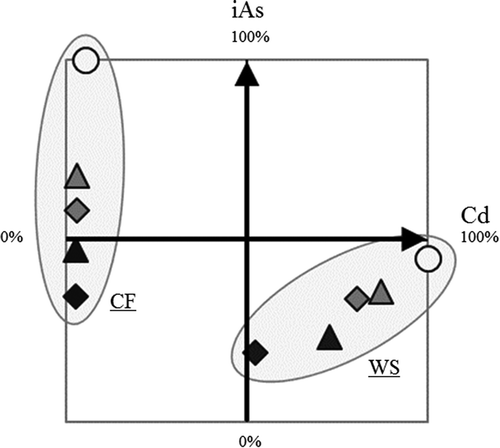
Supplemental_Materials.docx
Download MS Word (1 MB)Acknowledgments
We thank Ms Saruul Narangerel, Ms Manami Furuya and other part-time workers (Institute for Agro-Environmental Sciences, NARO) assisting our experiments. We thank Mr Hiroshi Yamaguchi and Mr. Terushi Kamada (Headquarter, NARO) for providing support with the pot experiment. We thank Prof. Shingo Matsumoto (Shimane University), Dr Gen Sakurai, Dr Noriko Yamaguchi, Dr Koji Baba, Dr Hidetaka Katou, Dr Ken Nakamura, and Dr Ikuko Akahane (Institute for Agro-Environmental Sciences, NARO) for providing useful suggestion and advice. We thank Mr Hayato Taniguchi (Sintokogio Ltd.) for sending Fe materials from a steel shot making factory. The ICP-OES and ICP-MS measurements were carried out at the Advanced Analysis Center, NARO. Part of the work was conducted under the collaborative research agreement between the Institute for Agro-Environmental Sciences, NARO, and Sintokogio Ltd.
Supplemental material
Supplemental data for this article can be accessed here.
Additional information
Funding
References
- Arao T, Kawasaki A, Baba K, Mori S, Matsumoto S 2009: Effects of water management on cadmium and arsenic accumulation and dimethylarsinic acid concentrations in Japanese rice. Environ. Sci. Technol., 43, 9361–9367. doi:10.1021/es9022738
- Chowdhury SR, Yanful EK 2013: Kinetics of cadmium (II) uptake by mixed maghemite-magnetite nanoparticles. J. Environ. Manage., 129, 642–651. doi:10.1016/j.jenvman.2013.08.028
- Chuan MC, Shu GY, Liu JC 1996: Solubility of heavy metals in a contaminated soil: effects of redox potential and pH. Water Air Soil Pollut., 90, 543–556. doi:10.1007/BF00282668
- Codex Alimentarius Commission 2006: Joint FAO/WHO Food Standards Programme, Codex Alimentarius Commission, 29th Session, Geneva, Switzerland. Available at http://www.fao.org/fao-who-codexalimentarius/meetings-reports/en/ (October, 2017).
- Codex Alimentarius Commission 2014: Joint FAO/WHO Food Standards Programme, Codex Alimentarius Commission, 37th Session, Geneva, Switzerland. Available at http://www.fao.org/fao-who-codexalimentarius/meetings-reports/en/ (October, 2017).
- Codex Alimentarius Commission 2016: Joint FAO/WHO Food Standards Programme, Codex Alimentarius Commission, 39th Session, Geneva, Switzerland. Available at http://www.fao.org/fao-who-codexalimentarius/meetings-reports/en/ (October, 2017).
- Farrow EM, Wang J, Burken JG, Shi H, Yan W, Yang J, Hua B, Deng B 2015: Reducing arsenic accumulation in rice grain through iron oxide amendment. Ecotoxicol. Environ. Saf., 118, 55–61. doi:10.1016/j.ecoenv.2015.04.014
- Furuya M, Hashimoto Y, Yamaguchi N 2016: Time-course changes in speciation and solubility of cadmium in reduced and oxidized paddy soils. Soil Sci. Soc. Am J., 80, 870–877. doi:10.2136/sssaj2016.03.0062
- Guo W, Hou YL, Wang SG, Zhu YG 2005: Effect of silicate on the growth and arsenate uptake by rice (Oryza sativa L.) seedlings in solution culture. Plant Soil., 272, 173–181. doi:10.1007/s11104-004-4732-0
- Hashimoto Y, Yamaguchi N 2013: Chemical speciation of cadmium and sulfur K-Edge XANES spectroscopy in flooded paddy soils amended with zerovalent iron. Soil Sci. Soc. Am J., 77, 1189–1198. doi:10.2136/sssaj2013.01.0038
- Honma T, Ohba H, Kaneko A, Nakamura K, Makino T, Katou H 2016a: Effects of soil amendments on arsenic and cadmium uptake by rice plants (Oryza sativa L. cv. Koshihikari) under different water management practices. Soil Sci. Plant. Nutr., 62, 349–356. doi:10.1080/00380768.2016.1196569
- Honma T, Ohba H, Kaneko-Kadokura A, Makino T, Nakamura K, Katou H 2016b: Optimal soil Eh, pH, and water management for simultaneously minimizing arsenic and cadmium concentrations in rice grains. Environ. Sci. Technol., 50, 4178–4185. doi:10.1021/acs.est.5b05424
- Hu P, Huang J, Ouyang Y, Wu L 2013: Water management affects arsenic and cadmium accumulation in different rice cultivars. Environ. Geochem. Health., 35, 767–778. doi:10.1007/s10653-013-9533-z
- Huang J-H, Wang S-L, Lin J-H, Chen Y-M, Wang M-K 2012: Dynamics of cadmium concentration in contaminated rice paddy soils with submerging time. Paddy Water Environ., 11, 483–491. doi:10.1007/s10333-012-0339-x
- Huff J, Lunn RM, Waalkes MP, Tomatis L, Infante PF 2007: Cadmium-induced cancers in animals and in humans. Int. J. Occup. Environ. Med., 13, 202–212. doi:10.1179/oeh.2007.13.2.202
- Ishikawa S, Makino T, Ito M, et al. 2016: Low-cadmium rice (Oryza sativa L.) cultivar can simultaneously reduce arsenic and cadmium concentrations in rice grains. Soil Sci. Plant Nutr., 62, 327–339. doi:10.1080/00380768.2016.1144452
- Kinniburgh DG, Jackson ML, Syers JK 1976: Adsorption of alkaline-earth, transition, and heavy-metal cations by hydrous oxide gels of iron and aluminum. Soil Sci. Soc. Am. J., 40, 796–799. doi:10.2136/sssaj1976.03615995004000050047x
- Kirk GJD, Bajita JB 1995: Root-induced iron oxidation, pH changes and zinc solubilization in the rhizosphere of lowland rice. New Phytol.., 131, 129–137. doi:10.1111/nph.1995.131.issue-1
- Kosmulski M 2011: The pH-dependent surface charging and points of zero charge V. Update. J. Colloid Interface Sci., 353, 1–15. doi:10.1016/j.jcis.2010.08.023
- Li G, Sun G, Williams PN, Nunes L, Zhu Y 2011: Inorganic arsenic in Chinese food and its cancer risk. Environ. Int., 37, 1219–1225. doi:10.1016/j.envint.2011.05.007
- Li RY, Stroud JL, Ma JF, McGrath SP, Zhao FJ 2009: Mitigation of arsenic accumulation in rice with water management and silicon fertilization. Environ. Sci. Technol., 43, 3778–3783. doi:10.1021/es803643v
- Makino T, Nakamura K, Katou H, et al. 2016: Simultaneous decrease of arsenic and cadmium in rice (Oryza sativa L.) plants cultivated under submerged field conditions by the application of iron-bearing materials. Soil Sci. Plant Nut., 62, 340–348. doi:10.1080/00380768.2016.1203731
- Matsumoto S, Kasuga J, Taiki N, Makino T, Arao T 2015: Inhibition of arsenic accumulation in Japanese rice by the application of iron and silicate materials. Catena, 135, 328–335. doi:10.1016/j.catena.2015.07.004
- Mishra D, Farrell J 2005: Evaluation of mixed valent iron oxides as reactive adsorbents for arsenic removal. Environ. Sci. Technol, 39, 9689–9694. doi:10.1021/es050949r
- Nozoe T, Sekiguchi T, Inoue T 2001: Effects of the addition of Fe(0) on the reduction of Fe(III) in submerged rice soil. Soil Sci. Plant Nutr., 47, 123–130. doi:10.1080/00380768.2001.10408374
- Oguri T, Yoshinaga J, Tao H, Nakazato T 2014: Inorganic arsenic in the Japanese diet: daily intake and source. Arch. Environ. Contam. Toxicol., 66, 100–112. doi:10.1007/s00244-013-9947-8
- Ok YS, Kim S-C, Kim D-K, Skousen JG, Lee J-S, Cheong Y-W, Kim S-J, Yang JE 2011: Ameliorants to immobilize Cd in rice paddy soils contaminated by abandoned metal mines in Korea. Environ. Geochem. Health., 33, 23–30. doi:10.1007/s10653-010-9364-0
- Ratering S, Schnell S 2000: Localization of iron-reducing activity in paddy soil by profile studies. Biogeochemistry, 48, 341–365. doi:10.1023/A:1006252315427
- Saito K, Yamamoto A, Sa T, Saigusa M 2005: Rapid, micro-methods to estimate plant silicon content by dilute hydrofluoric acid extraction and spectrometric molybdenum method. Soil Sci. Plant Nut., 51, 29–36. doi:10.1111/j.1747-0765.2005.tb00003.x
- Shimbo S, Zhang Z, Watanabe T 2001: Cadmium and lead contents in rice and other cereal products in Japan in 1998-2000. Sci. Total Environ., 281, 165–175. doi:10.1016/S0048-9697(01)00844-0
- Suda A, Baba K, Akahane I, Makino T 2016: Use of water-treatment residue containing polysilicate-iron to stabilize arsenic in flooded soils and attenuate arsenic uptake by rice (Oryza sativa L.) plants. Soil Sci. Plant Nut., 62, 111–116. doi:10.1080/00380768.2015.1137200
- Suda A, Yamaguchi N, Hayato T, Makino T in press: Arsenic immobilization in anaerobic soils by the application of by-product iron materials obtained from the casting industry. Soil Sci. Plant Nut. doi:10.1080/00380768.2017.1385371
- Ultra VU, Nakayama A, Tanaka S, Kang Y, Sakurai K, Iwasaki K 2009: Potential for the alleviation of arsenic toxicity in paddy rice using amorphous iron-(hydr)oxide amendments. Soil Sci. Plant Nut., 55, 160–169. doi:10.1111/j.1747-0765.2008.00341.x
- World Health Organization 2016. Arsenic. Fact Sheet. Available at http://www.who.int/mediacentre/factsheets/fs372/en/August 2017).
- Yoshikawa T, Jikihara T, Tanaka H 1986: Effects of manganese application on controlling cadmium uptake by rice. J. Plant Nutr. Soil Sci., 57, 77–80. (in Japanese).