ABSTRACT
Degradation of chitin, which is an aminopolysaccharide used as a soil amendment, has been often monitored in soil via its degradation products such as carbon dioxide and ammonium. We report here the applicability of thermogravimetry to measure the amount of chitin added to soil. The maximum pyrolysis rate of the upland surface soil of Brown Forest soil supplemented with chitin was strongly correlated with added chitin content (r = 0.999) when the content exceeded 6.0 g kg−1. The maximum pyrolysis rates of chitin-added soil (around 385°C) was distinctive from those of soil supplemented with cellulose, chitosan, N-acetylglucosamine, and N,N’-diacetylchitobiose (around 340°C, 300°C, 200°C, and 240°C, respectively), indicating the specific detection of chitin. Soil incubation study demonstrated that 60 g kg−1 chitin added to the soil declined exponentially (r = 0.993) within days and could not be detected at 90 days after the addition of chitin. Total carbon (C) content also decreased within days whereas total nitrogen (N) remained almost constant over the 90 days. The amount of ammonium-N increased in the initial 30 days after the addition of chitin and reached about 3.6 g kg−1, which corresponded to the amount of N in the added chitin (4.1 g kg−1) while the amount of nitrite-N and nitrate-N were below 2.0 and 15 mg kg−1, respectively. Comparison of the measured ammonium-N and total-C contents with those calculated from the measured chitin-content implied that addition of chitin enhanced degradation of native organic compounds in soil.
1. Introduction
Chitin is a polymer of N-acetylglucosamine (GlcNAc) that is linked by β-1,4 bonds. It has been suggested that soil treated with chitin or chitinous material serves as a means of controlling both soilborne fungal and nematode plant pathogens (Gooday Citation1990). In order to use chitin efficiently, it is necessary to monitor the degradation of chitin in agricultural soils. Some reports evaluated the degradation of chitin by measuring the weight of remaining chitin squares or flakes in nylon bags (Sato et al. Citation2010), others by measuring chitin-degradation products such as carbon dioxide and/or ammonium (Okafor Citation1966; Wieczorek et al. Citation2014), and yet others by converting chitin to hexosamine, which was finally measured colorimetrically (Johnson Citation1971; Pacovsky and Bethlenfalvay Citation1982; De Boer et al. Citation1999). More methods to detect and quantify chitin mixed with soil have remained to be developed.
Thermogravimetry continuously records the weight change of a material as a function of increasing temperature. Thermogravimetric analysis of chitin was reported and pyrolysis of chitin was observed around 380°C (Stolarek and Ledakowicz Citation2005; Stawski et al. Citation2008). We here report a method to measure the amount of chitin added to soil using thermogravimetry. The method was developed being inspired by the report (Ogura et al. Citation2013), in which the degradation of lignocellulosic compounds during the fermentation process of milled rice straw was evaluated by thermogravimetry.
2. Materials and methods
2.1. Soil sample and chemicals
The surface soil of Brown Forest soil in an upland field at the Center for Education and Research, Field Sciences (at latitude of 34.905502 north and longitude 138.272157 east), Faculty of Agriculture, Shizuoka University, was sampled in 2013. After air-drying at room temperature, the soil sample was passed through a sieve with 2 mm meshes. The physicochemical properties of the dried soil are shown in . The texture of soil used in this study was classified to Light Clay, based on the particle size distribution (). Chitin powder, chitosan powder, cellulose powder, and GlcNAc were purchased from Wako Chemicals, Japan. N,N'-diacetylchitobiose [(GlcNAc)2] was kindly supplied from Yaizu Suisankagaku Insustry (Yaizu, Shizuoka, Japan).
Table 1. Selected physicochemical properties of the air-dried Brown Forest soil examined in this study.
2.2. Evaluation of quantitative capability and specificity of thermogravimetry for measuring amount of chitin in soil
A portion of soil sample whose water content was adjusted to 60% of maximum water holding capacity (WHC) was mixed with chitin powder, chitosan powder, cellulose powder, GlcNAc, and (GlcNAc)2, separately, with a desired content (0–108 g kg−1), placed into a microtube and dried at 105°C overnight. After the dried soil was ground to a powder in a mortar, approximately 70 mg of each of the ground soil samples was loaded into an aluminum pan (5.0 mm diameter × 2.5 mm depth) and vaporized (heating rate = 5°C min−1, from room temperature to 500°C) in a nitrogen (N) atmosphere with a flow rate of 200 ml min−1 by using a thermogravimetry system (TG-DTA2020S/MS, Brucker) whose balance capacity, measurement range, and sensitivity are 1.0 g, ±200 mg, and ±0.1 mg, respectively. Based on the thermogravimetric data, rates of decline in soil weight (RDSW) (mg min−1) were calculated. The measurement was repeated three times and the average and the standard deviation values were calculated.
2.3. Measurement of chitin-content in soil
To 157 g of the air-dried sieved soil in a box, 10 g of powdered chitin [final ca. 60 g kg−1] was added and mixed. We selected 60 g kg−1 chitin as the optimal amount, based on the quantitative range of the method used to measure the amount of chitin added to soil using thermogravimetry (see Section 2.2. for the detailed method). The water content was kept at 60% of the maximum WHC. The box was covered with a lid with pine halls and incubated at 25°C. For analyses, soil was taken from 10 distinctive points in the box, mixed, and stored at −80°C. A portion of soil was placed into a microtube and dried at 105°C overnight. The dried soil was ground in a mortar, loaded into an aluminum pan, and vaporized as described in the above section. Based on the thermogravimetric data, the local maximum RDSW around 385°C was determined and the corresponding amount of chitin was calculated, based on a standard curve ().
Figure 1. Analysis of soil with or without chitin powder by thermogravimetry. (A) Alteration of relative weight (dashed lines) and rate of decline in weight (normal line) with or without chitin powder. The contents of chitin added to soil (g kg−1) are also indicated. (B) Relationship between the maximum rate of decline in weight and chitin content. The averages of triplicate measurements are plotted. Error bars indicate standard deviations. The result of linear approximation is shown by a formula with its correlation coefficient. ***p < 0.001.
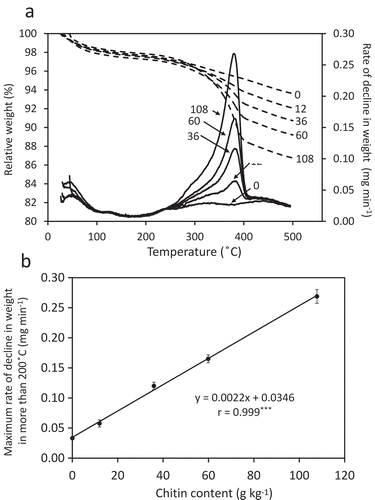
2.4. Measurement of total carbon, total nitrogen, ammonium, nitrite, nitrate, and acetic acid contents
Soil samples were dried at 105°C, ground in mortar, and loaded into a NC analyzer SUMIGRAPH NC-TR22 (Sumika Chemical Analysis Service, Osaka, Japan) to measure the total C and N contents. For measuring the ammonium nitrogen (NH4+-N), nitrite nitrogen (NO2–N), nitrate nitrogen (NO3–N), and acetic acid contents, 1 g of each soil sample was suspended in 10 ml of 2 mol L−1 potassium chloride (KCl), shaken at 250 rpm for 1 h, and centrifuged at 3000 rpm for 1 min, and the supernatant was subjected to assays. NH4+-N content was measured by the indophenol method. NO2–N content was measured by the Greiss reaction. NO3− was reduced using hydrazine sulfate to measure the NO3–N content. Acetic acid was measured using an Enzymatic BioAnalysis/Food Analysis kit for acetic acid (Roche).
2.5. Chitinase and N-acetylglucosaminidase assay
Two grams of each of the soil samples were suspended in 10 ml of 0.1 mol L−1 potassium phosphate buffer (pH 7.0), shaken at 250 rpm for 30 min, and centrifuged at 3,500 rpm for 10 min. The supernatant was passed through a filter paper. Proteins in the filtrate were then precipitated with 80% saturated ammonium sulfate. After correcting the precipitated proteins by centrifugation (15,000 rpm, 10 min, 4°C), proteins were dissolved in the 0.1 mol L−1 phosphate buffer (pH 7.0) and desalted using a PD-10 desalting column (GE Healthcare). Chitinase activity was measured by using 4-methylumbelliferyl-N,N'-diacetylchitobioside or 4-methylumbelliferyl-N,N',Nʺ-triacetylchitotrioside (Sigma, St. Louis, MO) according to a previously described method (Miyashita et al. Citation1991). N-acetylglucosaminidase (GlcNAcase) activity was evaluated using 4-methylumbelliferyl-N-acetyl-β-D-glucosamine (Sigma) as the substrate as reported previously (Saito et al. Citation2013). One unit of chitinase or GlcNAcase activity was defined as the amount of enzyme that liberated 1 × 10–6 mol of 4-metylumbelliferone from the corresponding substrate in 1 min at 37°C.
3. Results
3.1. Quantitative capability and specificity of thermogravimetric analysis for measuring the content of chitin added to soil
When pure chitin powder or air-dried soil mixed with chitin was simply analyzed by thermogravimetry after drying at 105°C, maximum RDSW was observed at 360°C (data not shown). In contrast, the main peak of RDSW of chitin-added soil was found between 380°C and 388°C when the soil had been wet once with water to 60% of WHC prior to drying at 105°C (). We assume that this could be attributed to the formation of hydrogen bonds between chitin and soil particles, which are formed directly or via water molecules during the drying process.
Local maximum RDSW values around 385°C seemed to increase in accordance with the increase in chitin content (). There was a significant correlation between the local maximum RDSW values and chitin content (). Chitin at 6.0 g kg−1 was measurable, 1.2 g kg−1 appeared to be below the detection limit (). It is thus concluded that chitin, when added to soil, can be accurately measured if the content exceeds 6.0 g kg−1.
The specificity of chitin detection by RDSW was confirmed by comparing the RDSW patterns with those of other polymers, as well as with the monomer GlcNAc and its dimer (GlcNAc)2. Cellulose and chitosan, which are respectively the polymers of glucose and glucosamine linked by β-1,4 bonds, formed RDSW peaks at 340°C and 300°C, respectively, whereas the RDSW peak of chitin was at 385°C (). The RDSW peaks of GlcNAc and (GlcNAc)2 were at 210°C and 240°C, respectively (). It was thus concluded that chitin polymer was specifically detected by thermogravimetric analysis and could be distinguished from other polysaccharides and shorter chitin-saccharides.
Figure 2. Thermogravimetric analysis of soil supplemented with cellulose or chitosan powder (A) and with N-acetylglucosamine (GlcNAc) or N,N'-diacetylchitobiose [(GlcNAc)2] (B) in comparison that with chitin powder. Rates of decline in weight are plotted. The content of each supplement added to soil was set to 60 g kg−1.
![Figure 2. Thermogravimetric analysis of soil supplemented with cellulose or chitosan powder (A) and with N-acetylglucosamine (GlcNAc) or N,N'-diacetylchitobiose [(GlcNAc)2] (B) in comparison that with chitin powder. Rates of decline in weight are plotted. The content of each supplement added to soil was set to 60 g kg−1.](/cms/asset/98b353b9-853c-4a50-9914-357003a5ccc0/tssp_a_1457408_f0002_b.gif)
3.2. Chitin-degradation evaluated by thermogravimetric analysis
To monitor degradation profile of chitin in soil by using thermogravimetry, we added 60 g kg−1 of powdered chitin to the upland surface soil of Brown Forest soil (). We selected 60 g kg−1 by considering the measurable range of thermogravimetric analysis (), although the amount was higher than those in reported studies (less than 24 g kg−1) (De Boer et al. Citation1999; Hora and Baker Citation1972; Mitchell and Alexander Citation1962; Murakami et al. Citation2009; Sato et al. Citation2010; Setälä and McLean Citation2004; Schippers and Palm Citation1973; Sneh and Henis Citation1972; Takagi et al. Citation1992; Williams and Robinson Citation1981). The peak of RDSW around 385°C decreased with incubation days (), which data indicated the decline of the amount of chitin added to the soil. The chitin contents, which were calculated from the local maximum RDSW values around 385°C, decreased exponentially with days and chitin was not detected in the soil at 90 days after the addition of chitin (). The exponential approximation formula for the chitin remain was as follows.
[c, chitin content (g kg−1) at the day t; t, days after the addition of chitin]
The rate of chitin decrease should be expressed as follows, based on the above formula.
[v, rate of chitin decrease (g kg−1 d−1) at the day t; t, days after the addition of chitin]
Figure 3. Thermogravimetric analysis of the soil incubated at 25°C after adding chitin. Alteration of relative weight (dashed lines) and rate of decline in weight (normal line) of the soil samples (A) and chitin contents determined based on the maximum rates of decline in weight (B). The logarithmic scale version of panel B is shown in (C). In panel A, days after the addition of chitin are indicated. In panels B and C, averages of triplicate measurements for the duplicate samples are plotted. Error bars indicate standard deviations of total six measurements. The result of exponential approximation is shown with the formula and correlation coefficient in panel C. The dotted line in panel B has drawn according to the approximation formula obtained in C. ***p < 0.001.
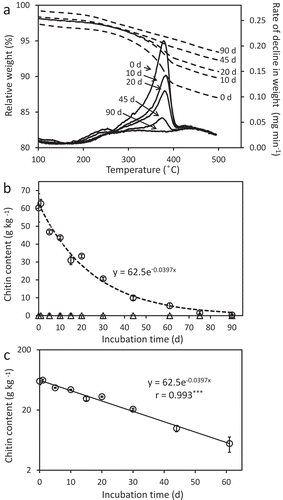
The initial increased total C content due to the chitin addition (28 g kg−1) declined with incubation period, but the total C content at 90 days after the addition of chitin was significantly (6 g kg−1) higher than that of the soil without chitin amendment () in contrast to chitin amount (). It was assumed that most of the amended chitin was released from the soil as gas, probably as carbon dioxide. The remained C should be biomass C converted from added chitin, although it was unclear if the biomass were contained in alive or dead cells. In contrast to C, total N contents were rather constant (). The initial increased N content (about 4 g kg−1) corresponded well with that of the added chitin (4.1 g kg−1). As chitin was not detected at 90 days after chitin addition (), most of the remained N at 90 days in the chitin-amended soil must be N of chitin-degradation products or their converted compounds.
Figure 4. Total carbon (A) and total nitrogen (B) contents, and carbon/nitrogen ratio (C/N ratio) (C) in soil with (circles) or without (triangles) the addition of chitin powder. Soil was incubated at 25°C for 90 days after adding chitin. Soil samples were prepared in duplicate. Averages of duplicate measurements are plotted. Error bars indicate the maximum and minimum values obtained in the duplicate measurement. The dashed line in panel A indicates the estimated total carbon contents that were calculated by adding the carbon contents in the remaining chitin to the initial measured total carbon content.
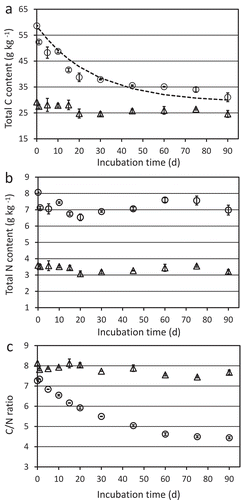
3.3. Ammonium, nitrite, nitrate, and acetate contents, and pH
In the chitin-amended soil, ammonium nitrogen (NH4+-N) contents were increasing in the initial 15–20 days after the addition of chitin, whereas nitrite nitrogen (NO2–N) and nitrate nitrogen (NO3–N) contents were quite low (below 2.0 and 15 mg kg−1, respectively) (). The final NH4+-N contents were around 4.3 g kg−1 and corresponded well with the N contents in the added chitin (4.1 g kg−1). We thus concluded that the added chitin-N was mostly accumulated as NH4+-N in the soil. pH was increased in the incubated soil until 20 days after the addition of chitin, then gradually lowered (). The increment pattern of pH apparently coincided well with that of the NH4+-N content (), implying the contribution of NH4+ to the pH increment. Acetate, which would be formed by deacetylation of chitin or GlcNAc, was not detected (data not shown), as Wieczorek et al. (Citation2014) reported in a soil incubation study in oxidative condition.
Figure 5. Ammonium (A), nitrite (B), and nitrate (C)-nitrogen contents, and pH (D) in soil with (circles) or without (triangles) the addition of chitin powder. Soil was incubated at 25°C for 90 days after adding chitin. Soil samples were prepared in duplicate and the average values are plotted. Error bars indicate the maximum and minimum values obtained in the duplicate measurement. The dashed line in panel A indicates the estimated NH4+-N contents that were calculated from the measured chitin contents under the hypothesis that all the degraded chitin-N is accumulated as NH4+-N in the soil. pH was measured in water (soil:water = 1:2.5).
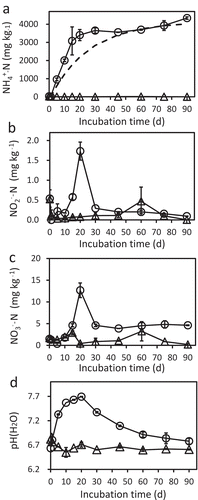
3.4. Chitinase and GlcNAcase activity
Chitin degradation activity in soil was evaluated by measuring chitinase and GlcNAcase activity in soil extracts. Activities of the both enzymes were induced by the chitin amendment (). Chitinase activity peaked at 5 or 10 days after the addition of chitin, whereas GlcNAcase activity increased until 15 or 20 days and then decreased gradually (). The chitinase and GlcNAcase activities, respectively, peaked in the initiation and middle of chitin degradation process ().
Figure 6. Chitinase (circles and triangles) and N-acetylglucosaminidase (squares) activities in the extracts from chitin-added soil. Chitinase activity was measured by using 4-methylumbelliferyl (MU)-(GlcNAc)2 (circles) or 4MU-(GlcNAc)3 (triangles), while N-acetylglucosaminidase activity was measured with 4MU-GlcNAc. Soil samples were prepared in duplicate and the average enzymatic activities are shown. Error bars indicate the maximum and minimum activities of the duplicated soil samples.
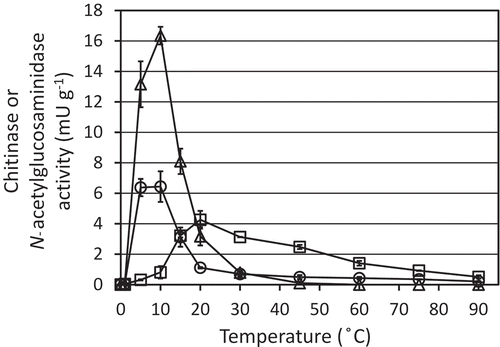
4. Discussion
In this study, we attempted to measure chitin content by thermogravimetry and successfully demonstrated its capability for measuring chitin added to soil in a range exceeding 6.0 g kg−1. The detection limit could be lowered to 2.4 g kg−1 by using a deeper sample pan (5.0 mm diameter × 2.5 mm depth) (data not shown). However, this sensitivity is still lower than the chitin content found in some field and laboratory experiments (Mitchell and Alexander Citation1962; Hora and Baker Citation1972; Sneh and Henis Citation1972; De Boer et al. Citation1999; Setälä and McLean Citation2004), which may have been done according to the general recommended application rate of chitin (less than 6 g kg−1). Nevertheless, the thermogravimetric quantification of chitin may find applications if chitin is added more than 6.0 g kg−1, as often found in several experiments (Schippers and Palm Citation1973; Williams and Robinson Citation1981; Takagi et al. Citation1992; Murakami et al. Citation2009; Sato et al. Citation2010), although we have to investigate the applicability of the method to soils of other textures. For low levels of chitin, other chemical methods should be used because of their high sensitivities. Alternatively, by increasing the heating rate in the thermogravimetric measurement, the sensitivity could be improved (Stolarek and Ledakowicz Citation2005) and the analysis time could be saved, which the probabilities are being investigated.
Thermogravimetry could also be applied for the detection and possible measurement of the amount of cellulose and chitosan added to soil (). Other compounds could also be detected and quantified in soil by the method.
In this report, we added 60 g kg−1 of chitin according to the quantifiable range (more than 6.0 g kg−1) of the thermogravimetric analysis, in order to obtain detailed chitin degradation profile in soil. The soil incubation experiment clearly demonstrated that the chitin amount exponentially decreased (). It is thus concluded that the chitin degradation has occurred according to a primary reaction formula, indicating the chitin degradation rate depends on the amount of chitin at the time. Although it is didactically known that organic compounds are often degraded exponentially in soil, the obtained formula () enabled us to estimate the amounts of N and C which are formed with chitin-degradation. If the all of the N in chitin was converted to ammonium, the NH4+-N content in the soil should have increased according to the chitin degradation. Similarly, the total-C content should have decreased with the chitin-degradation. However, the NH4+-N and total-C contents seemed increased and decreased, respectively, earlier than those estimated from the decrease of the chitin content ( and ). We assume that the addition of chitin enhanced the mineralization of native organic N, showing priming effect like addition of other organic matters.
In this study, the added chitin-N seemed mostly accumulated as NH4+-N in the soil (). Such almost complete conversion and accumulation of chitin-N as NH4+-N has already been reported (Williams and Robinson Citation1981). It is known that ammonia, which is a fungistatic volatile, is formed in chitin amended soil (Schippers and Palm Citation1973; Wieczorek et al. Citation2014). Accumulation of NH4+-N with little nitrification activity () may be characteristics of chitin added soils. Alternatively, addition of much amount of chitin (60 g kg−1 in this study) may cause such abnormal accumulation of NH4+-N.
Induction of soil chitinase and GlcNAcase activity by chitin has been observed (Mitchell and Alexander Citation1962; Ueno and Miyashita Citation2000; Jacquiod et al. Citation2013). The mechanisms of the induction of production of chitinolytic enzymes by chitin have been investigated in chitinolytic soil bacteria such as streptomycetes and Serratia marcescens (Saito et al. Citation2013; Takano et al. Citation2014). The induced chitinase and GlcNAcase activities declined immediately and gradually, respectively (). Biotic and abiotic factors of declines of chitinase and GlcNAcase activity in soil remain to be elucidated. The enzymes might have been degraded by proteolytic enzymes or adsorbed to solid phase of the soil.
It was noteworthy that the increment pattern of GlcNAcase activity seemed similar with that of the NH4+-N content ( and ). The induced chitinolytic enzymes, especially GlcNAcase, might be involved in the enhancement of mineralization of soil-native organic N to ammonium.
Acknowledgments
The authors thank Ayano Masui at Yaizu Suisankagaku Industry for providing N,N'-diacetylchitobiose.
Additional information
Funding
References
- De Boer W, Gerards S, Pja KG, Modderman R 1999: Response of the chitinolytic microbial community to chitin amendments of dune soils. Biol. Fertil. Soils, 29, 170–177. doi:10.1007/s003740050541
- Gooday GW 1990. The ecology of chitin degradation. Adv. Microb. Ecol., 11, 387–430.
- Hora TS, Baker R 1972: Soil fungistasis: microflora producing a volatile inhibitor. Trans. Br. Mycol. Soc., 59, 491–500. doi:10.1016/S0007-1536(72)80130-X
- Jacquiod S, Franqueville L, Cécillon S, Vogel TM, Simonet P 2013: Soil bacterial community shifts after chitin enrichment: an integrative metagenomic approach. PLoS One, 8, e79699. doi:10.1371/journal.pone.0079699
- Johnson AR 1971: Improved method of hexosamine determination. Anal. Biochem., 44, 628–635. doi:10.1016/0003-2697(71)90252-1
- Mitchell R, Alexander M 1962: Microbiological processes associated with the use of chitin for biological control. Soil Sci. Soc. Am. Proc., 26, 556–558. doi:10.2136/sssaj1962.03615995002600060013x
- Miyashita K, Fujii T, Sawada Y 1991: Molecular cloning and characterization of chitinase genes from Streptomyces lividans 66. J. Gen. Microbiol., 137, 2065–2072. doi:10.1099/00221287-137-9-2065
- Murakami H, Kuroyanagi Y, Tsushima S, Shishido Y 2009. Effects of application of organic materials on the development of clubroot disease caused by Plasmodiophora brassicae (in Japanese). Soil Microorg., 63, 3–8.
- Ogura T, Date Y, Kikuchi J 2013: Differences in cellulosic supramolecular structure of compositionally similar rice straw affect biomass metabolism by paddy soil microbiota. PLoS One, 8, e66919. doi:10.1371/journal.pone.0066919
- Okafor N 1966: Estimation of the decomposition of chitin in soil by the method of carbon dioxide release. Soil Sci., 102, 140–142. doi:10.1097/00010694-196608000-00010
- Pacovsky RS, Bethlenfalvay GJ 1982: Measurement of the extraradical mycelium of a vesicular-arbuscular mycorrhizal fungus in soil by chitin determination. Plant Soil, 68, 143–147. doi:10.1007/BF02374736
- Saito A, Ebise H, Orihara Y, Murakami S, Sano Y, Kimura A, Sugiyama Y, Ando A, Fujii T, Miyashita K 2013: Enzymatic and genetic characterization of the DasD protein possessing N-acetyl-β-D-glucosaminidase activity in Streptomyces coelicolor A3(2). FEMS Microbiol. Lett., 340, 33–40. doi:10.1111/fml.2013.340.issue-1
- Sato K, Azama Y, Nogawa M, Taguchi G, Shimosaka M 2010: Analysis of a change in bacterial community in different environments with addition of chitin or chitosan. J. Biosci. Bioeng., 109, 472–478. doi:10.1016/j.jbiosc.2009.10.021
- Schippers B, Palm LC 1973: Ammonia, a fungistatic volatile in chitin-amended soil. Neth. J. Plant Pathol., 79, 279–281. doi:10.1007/BF01976677
- Setälä H, McLean MA 2004: Decomposition rate of organic substrates in relation to the species diversity of soil saprophytic fungi. Oecologia, 139, 98–107. doi:10.1007/s00442-003-1478-y
- Sneh B, Henis Y 1972: Production of antifungal substances active against Rhizoctonia solani in chitin-amended soil. Phytopathology, 62, 595–600. doi:10.1094/Phyto-62-595
- Stawski D, Rabiej S, Herczyriska L, Draczyriski Z 2008. Thermogravimetric analysis of chitins from different origin. J. Therm. Anal. Calorim., 93. doi:10.1007/s10973-007-8691-6
- Stolarek P, Ledakowicz S 2005: Pyrolysis kinetics of chitin by non-isothermal thermogravimetry. Thermochim. Acta, 433, 200–208. doi:10.1016/j.tca.2005.03.012
- Takagi S, Kitamura A, Marumoto T 1992. Control of Fusarium disease using antagonistic actinomycetes II. Selection of organic matter and the presentation of microbial materials containing antagonistic actinomycetes (in Japanese). Soil Microorg., 39, 41–48.
- Takano S, Honma S, Miura T, Ogawa C, Sugimoto H, Suzuki K, Watanabe T 2014: Construction and basic characterization of deletion mutants of the genes involved in chitin utilization by Serratia marcescens 2170. Biosci. Biotechnol. Biochem., 78, 524–532. doi:10.1080/09168451.2014.882755
- Ueno H, Miyashita K 2000: Inductive production of chitinolytic enzymes in soil microcosms using chitin, other carbon-sources, and chitinase-producing Streptomyces. Soil Sci. Plant Nutr., 46, 863–871. doi:10.1080/00380768.2000.10409152
- Wieczorek AS, Hetz SA, Kolb S 2014: Microbial responses to chitin and chitosan in oxic and anoxic agricultural soil slurries. Biogeosciences, 11, 3339–3352. doi:10.5194/bg-11-3339-2014
- Williams ST, Robinson CS 1981. The role of streptomycetes in decomposition of chitin in acidic soils. J. Gen. Microbiol., 127, 55–63.