ABSTRACT
The global warming has a potential for acceleration of labile soil organic carbon decomposition. Arrhenius equation is one of the useful equation for predicting temperature sensitivity of carbon decomposition, with the activation energy of rate constant being a key factor. The purpose of this study is the evaluation of temperature sensitivity of labile soil organic carbon decomposition under anaerobic condition in wetland soil using the activation energy of rate constant among different vegetation types. The soil samples were incubated at three different temperatures (10, 20, and 30°C) under anaerobic condition and carbon decomposition rates (sum of CO2 and CH4 production) were measured by gas chromatography. The first-order kinetic model with Arrhenius equation was used for approximate of anaerobic carbon decomposition. For determination of activation energy of rate constant, non-linear least-squares method was conducted between observed carbon decomposition rate and predicted carbon decomposition rate which calculated by Arrhenius equation. The activation energy of rate constant of anaerobic labile soil organic carbon decomposition was different among vegetation types. We successfully determined the activation energy of rate constant of CO2 or CH4 production from Phragites, Juncus, and Miscanthus+Cirsium-dominated vegetation soil with Arrhenius equation. Hence, this study suggests that Arrhenius equation was useful for evaluation of temperature sensitivity of labile soil organic carbon decomposition not only aerobic condition, but also anaerobic condition among several vegetation types in the wetland ecosystem. Moreover, gaseous carbon production from soil under Juncus yocoscensis dominated soil appeared higher activation energy and temperature sensitivity than that from soil under other vegetation types.
1. Introduction
Global carbon dynamics of terrestrial ecosystems affects the concentration of greenhouse gases (e.g. CO2 and CH4) in the atmosphere. Rising temperatures are expected to stimulate soil respiration, both by accelerating rates of carbon cycling via autotrophic respiration and by providing a potentially powerful positive feedback to climatic warming via heterotrophic decomposition of organic matter (Carey et al. Citation2016). Labile soil organic carbon (LSOC) is the most active fraction of soil organic carbon with rapid turnover rates less than a few years (Parton et al. Citation1987). The temperature sensitivity of LSOC decomposition is an intensively discussed because it has important feedback on the global carbon dynamics (Davidson and Janssens Citation2006). Conventionally, the increase in reaction rate per 10°C rising, i.e. the Q10 coefficient is been used to describe the temperature response of soil carbon mineralization, likewise is the Arrhenius equation (Wang et al. Citation2015).
It is one of the most reasonable equations of thermodynamic process. It showed the activation energy of rate constant as follows:
where k is rate constant of the chemical reaction, A is the pre-exponential factor, T is the absolute temperature (K), R is the universal gas constant (8.31 J K−1 mol−1), and Ea is the activation energy of the reaction (J mol−1). According to this equation, activation energy is a key factor for evaluating the temperature sensitivity of chemical reaction. However, most of the study about the evaluation of the effect of temperature on soil carbon mineralization using the Arrhenius equation has been conducted under oxic condition (Knorr et al. Citation2005; Fierer et al. Citation2005; Craine et al. Citation2010).
Soil organic carbon decomposition under anoxic conditions is different to that under oxic condition because not only CO2 but also CH4 is produced. Moreover, decomposition rate is slower than under oxic conditions (Boon et al. Citation2006). Inubushi et al. (Citation1997) showed the usefulness of Arrhenius equation for evaluation of activation energy of carbon decomposition from paddy soil under anaerobic condition. However, the activation energy of anaerobic carbon decomposition has not been yet determined in cool-template wetland soil. Wetlands represent the largest component of the terrestrial biological carbon pool, and thus play an important role in global carbon cycles (Dixon and Krankina Citation1995; Sahagian and Melack Citation1996). The positive global warming feedback is more likely occurred at cool-temperature field compared with at warming field. Moreover, the above vegetation types are having also big an effect on the temperature sensitivity of soil organic carbon decomposition (Inglett et al. Citation2012). The objective of this study is the evaluation of the temperature sensitivity of anaerobic LSOC decomposition using activation energy calculated by Arrhenius equation in cool-temperate brackish marsh among three vegetation types.
2. Materials and methods
2.1. Study site
The study site was located in a cool-temperate brackish marsh around Lake Obuchi in Aomori Prefecture (40°57´N, 141°22´E; ). The average annual precipitation and temperature is 1488 mm and 10.8°C, respectively (Japan Meteorological Agency,2005; Available from: http://www.jma.go.jp/jma/menu/report.html).
The brackish marsh was classified into three typical vegetation types: Phragmites vegetation, where Phragmites. australis (Cav.) Trin. ex Steudel represented 72.5% of the vegetation, Juncus vegetation which Juncus yokoscensis (Franch. & Sav.) Satake covering in 54.3%of the vegetation, and Miscanthus+Cirsium vegetation, with Miscanthus sinensis Andersson and Cirsium inundatum Makino, which covered 37.8% and 26.0%, respectively (Suzuki et al. Citation2010).
Each vegetation boundaries were distinct, and the order of vegetation arrangement, Phragmites vegetation, Juncus vegetation, and Miscanthus+Cirsium vegetation, corresponded to the distance from the shore of Lake Obuchi (). Phragmites vegetation is the nearest vegetation from the lake shore. The average water table level was around the soil surface level for Phragmites and Juncus vegetation, and approximately 15 cm below the soil surface for Miscanthus+Cirsium vegetation. The soil chemical properties in each vegetation types are described in .
Table 1. Soil chemical properties among three vegetation types.
2.2. Incubation experiments and gas production
Five soil cores (30 cm in depth and 5.5 cm in diameter) were collected on late June 2008 using a core sampler (Hand sampler HS-30S, Fujiwara Scientific CO., LTD, Tokyo, Japan) from each of three vegetation types (in total 15 cores). Surface layer soils (0–5 cm) were collected from each core. Each surface layer soil in each vegetation samples was immediately sieved (2 mm) for use in the incubation experiment. The soil sample was flooded with distilled water and preincubated under O2 free nitrogen condition for 14 days. In addition, 10 g of soil samples were put into 30-mL serum vials and their soil water content was adjusted to simulate a waterlogged condition with distilled water prior to the incubations. Headspace gas in the bottle was then replaced with nitrogen gas. After that, the bottle was sealed tightly with a butyl stopper. Five tubes of soils from each vegetation were incubated at each three temperatures (10, 20, and 30°C) in the dark for 168 days, thus representing the LSOC as it is decomposed within such short time (Parton et al. Citation1987). At seven-day intervals, CO2 and CH4 were quantified by gas chromatography with thermal conductivity detector (TCD) and flame ionization detector (FID), respectively (Inubushi et al. Citation1997). The sum of CO2+CH4 production was determined as the observed gaseous carbon production (Gobs: g–C g soil−1). After each sampling, the headspace gas was replaced with fresh N2.
2.3. Calculation of activation energy of rate constant
Soil organic carbon (C) mathematically separated into two components is represented as follows:
where C1 (g–C g soil−1) represents the LSOC fraction and C2 (g–C g soil−1) represents the recalcitrant organic carbon fraction, which was hardly decomposed during the incubation period (168 days).
In C1 fraction, the first-order kinetic model proposed changes of organic carbon content in time (t) as follows:
where k1 is the decomposition rate constant of C1 fraction.
The LSOC decomposition rate is described with first-order model as follows:
where C1,t is LSOC decomposition rate in the time t, t is incubation days (d), and C1,0 is LSOC fraction at day 0. Therefore, cumulative LSOC decomposition during incubation period (Gcal) is indicated as follows:
The apparent activation energy of the anaerobic LSOC decomposition rate constant is described with Arrhenius equation as follows:
where k10 is the decomposition rate constant at the soil temperature of 10°C, E1 (J mol−1) is the activation energy in the Arrhenius equation, R is the universal gas constant (8.31 J K−1 mol−1), T (K) is soil temperature on incubation days, and T0 (K) is the reference temperature (283 K in this study). Therefore, Equation (4) can be written as
For fitting Gcal at Equation (6), C1,0, k10, and E1 were calculated to minimize the residual sum of squares between Gcal and Gobs using a non-linear least-squares method under all incubation temperature and incubation days with Microsoft Excel Solver program. The suitable C1,0, k10, and E1 were determined on each vegetation types (n = 5). The determination coefficient was obtained by linear regression analysis between Gobs and Gcal.
The Q10 values of k1 are calculated as follows:
where k1t is k1 in temperature t (K) and k1(t+10) is k1 in temperature t + 10 (K).
3. Statistical analysis
Statistical analysis was performed by using SPSS software (SPSS Inc., Chicago, IL, USA). The significant differences in CO2, CH4 and CO2+CH4 production among vegetation types were estimated by the Tukey method at p < 0.05 level.
4. Results and discussion
CO2+CH4 production rates from soil under Miscanthus+Cirsium vegetation were the highest among the three vegetation types, while that from soil under Juncus vegetation had the lowest rates under all temperatures (). However, CO2+CH4 production from soil under Juncus increased more than four times and twice between 10 and 20°C and 20–30°C, respectively (see in Supplementary Material). Soil under Phragmites vegetation and Miscanthus+Cirsium vegetation experienced doubled carbon production rates between the ranges 10–20°C and 20–30°C, respectively. In all samples, the temperature dependency of CO2 or CH4 production was differed ( in Supplementary Material). The CO2 production from soil under Juncus vegetation increased about 4.7 times between 10 and 20°C while that from other vegetation and temperature indicates around twice ( in Supplementary Material). The CH4 production was increased about four and six times between 10 and 20°C from soil under Juncus and Miscanthus+Cirsium vegetation, respectively. The Q10 values of CO2+CH4 production from wetland and paddy field are ranged from 1.2 to 9.13 (Kirwan et al. Citation2014; Wang et al. Citation2015; Leroy et al. Citation2017). The Q10 values of CH4 production were higher in Miscanthus+Cirsium vegetation, which was the farthest vegetation from the lake shore, than other vegetation types. In this site, EC was drastically changed along with the distance from the lake shore. Neubauer (Citation2013) indicated that temperature sensitivity of CO2 emission did not vary with salinity, while that of CH4 emission decreased with increasing salinity. However, this did not explain fully the difference of temperature sensitivity of anaerobic LSOC decomposition among vegetation types.
Figure 2. The carbon mineralization from anaerobic soil among each vegetation types under 10, 20, and 30°C during incubation period. Opened circle; Phragmites vegetation, opened square; Juncus vegetation and opened triangle; Miscanthus+Cirsium vegetation.
Error bars indicate the standard variation of each sample (n = 5).
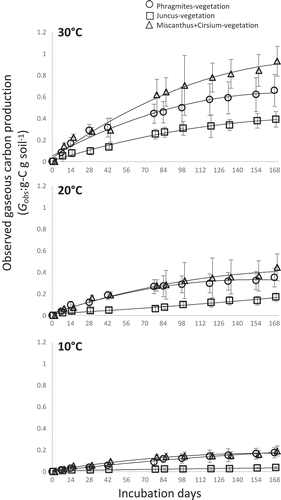
The residual sum of square between Gobs and Gcal of CO2+CH4 was <0.05 in each vegetation types (). Under this condition, E1, k10, and C1,0 were determined for CO2+CH4 prediction from soil under each vegetation (). The determination coefficients of linear regression between Gobs and Gcal of CO2+CH4 were >0.98 in each vegetation types and they were positively correlated ( and ). Therefore, anaerobic LSOC decomposition was well fitted for first-order models with Arrhenius equation, like it was done for aerobic carbon decomposition as Knorr et al. (Citation2005). These high correlations indicated that the E1, k10, and C1,0 were successfully estimated by non-linear least-squares method. E1 for CO2+CH4 production was determined as 7.65 × 104, 1.05 × 105, and 7.98 × 104 J mol−1 from soil under Phragmites, Juncus and Miscanthus+Cirsium vegetation, respectively (). E1 of CO2+CH4 production from soil under in each vegetation in the brackish marsh was higher than previous studies, anaerobic carbon decomposition in field soil and aerobic carbon decomposition (3.9 × 104 to 5.3 × 104 J mol−1, Inubushi et al. Citation1997; Knorr et al. Citation2005). Therefore, temperature effects of LSOC decomposition in wetland were more sensitive than that in paddy field and under aerobic condition. Several studies also showed that the labile carbon pool was less temperature sensitive than recalcitrant organic carbon fraction, because recalcitrant carbon pool has a higher activation energy (Knorr et al. Citation2005; Davidson and Janssens Citation2006). Before plantation, paddy fields were plowed and soil was exposed to oxygen. In this process, soil organic carbon might be drastically decomposed and LSOC fraction might be increased than that in the wetland ecosystem.
Table 2. Residual sum of square (RSS) and determination coefficient (R2) between the calculated and the observed CO2, CH4 and CO2 + CH4 production.
Table 3. The labile soil organic carbon fraction on day 0 (C1,0), rate constant at 10°C (k10), and activation energy of rate constant (E1) in each vegetation types.
Figure 3. Relationships between the calculated gaseous carbon production (Gcal) and the observed gaseous carbon production (Gobs).
Dotted line indicated a line with a slope of 1.
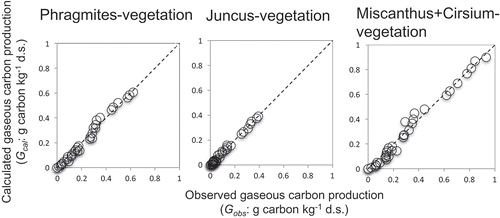
Temperature sensitivity of anaerobic labile soil carbon decomposition was higher under Juncus vegetation than under the other vegetation types. As CO2+CH4 production, these parameters were also successfully determined in CO2 or CH4 production from each vegetation soil, respectively ( in Supplementary Material). E1 was the highest in case of Juncus vegetation, and Miscanthus+Cirsium vegetation for CO2 and CH4 production, respectively. Q10 of k1 for CO2 or CO2+CH4 production from soil under Juncus vegetation was higher than other vegetation ( and in Supplementary Material). Therefore, soil under Juncus vegetation indicated higher temperature sensitivity of anaerobic labile carbon decomposition than under the other vegetation types. Moreover, CO2 production was more strongly affecting than CH4 production from soil under Juncus vegetation. Bray et al. (Citation2012) showed that plant residue of Juncus related species has lower lignin content and faster decomposition rate than other wetland plants. This study suggested that soil chemical properties and LSOC molecular construction might complexly correlated temperature sensitivity of anaerobic LSOC decomposition.
Table 4. The Q10 values of decomposition rate constant (k1) between for CO2, CH4, and CO2+CH4 production among different vegetation types.
Future research will focus on the interaction between the detailed chemical composition of labile carbon fraction, soil chemical properties and temperature sensitivity of carbon decomposition under anaerobic condition.
Supplemental Material
Download PDF (191.6 KB)Supplementary material
Supplementary materials can be accessed here
References
- Boon PI, Pollard PC, Ryder D 2006: Wetland microbial ecology and biogeochemistry. In Ecology of Freshwater and Estuarine Wetlands, Ed. Batzer DP, Sharitz RR, pp. 87–132. University of California Press, Berkeley.
- Bray SR, Kitajima K, Mack MC 2012: Temporal dynamics of microbial communities on decomposing leaf litter or 10 plant species in relation to decomposition rate. Soil Biol. Biochem., 49, 30–37. doi: 10.1016/j.soilbio.2012.02.009
- Carey JC, Tang J, Temple PH et al. 2016: Temperature response of soil respiration largely unaltered with experimental warming. Pros. Natl. Acad. Sci. USA, 113, 13797–13802. doi: 10.1073/pnas.1605365113
- Craine JM, Fierer N, McLauchlan KK 2010: Widespread coupling between the rate and temperature sensitivity of organic matter decay. Nat. Geosci, 3, 854–857. doi: 10.1038/ngeo1009
- Davidson EA, Janssens IA 2006: Temperature sensitivity of soil carbon decomposition and feedbacks to climate change. Nature, 440, 165–173. doi: 10.1038/nature04514
- Dixon RK, Krankina ON 1995: Can the terrestrial biosphere be managed to conserve and sequester carbon? In NATO ASI Series I Global Environmental Change, Ed. Beran MA, pp. 153–182. Springer-Verlag, New York.
- Fierer N, Craine JM, Mclauchan K, Schimel JP 2005: Litter quality and the temperature sensitivity of decomposition. Ecology, 86, 320–326. doi: 10.1890/04-1254
- Inglett KS, Inglett PW, Reddy KR, Osborne TZ 2012: Temperature sensitivity of gas production in wetland soils of different vegetation. Biogeochemistry, 108, 77–90. doi: 10.1007/s10533-011-9573-3
- Inubushi K, Hori K, Matsumoto S, Wada H 1997: Anaerobic decomposition of organic carbon in paddy soil in relation to methane emission to the atmosphere. Water Sci. Technol., 36, 523–530.
- Kirwan ML, Guntenspergen GR, Langley JA 2014: Temperature sensitivity of organic-matter decay in tidal marshes. Biogeoscience, 11, 4801–4808. doi: 10.5194/bg-11-4801-2014
- Knorr W, Prentice IC, House JI, Holland EA 2005: Long-term sensitivity of soil carbon turnover to warming. Nature, 433, 298–301. doi: 10.1038/nature03226
- Leroy F, Gogo S, Guimbaud C, Bernard-Jannin L, Hu Z, Laggoun-Défarge F 2017: Vegetation composition controls temperature sensitivity of CO2 and CH4 emission and DOC concenteraion in peatlands. Soil Biol. Biochem., 107, 164–167. doi: 10.1016/j.soilbio.2017.01.005
- Neubauer SC 2013: Ecosystem responses of a tidal freshwater marsh experiencing saltwater intrusion and altered hydrology. Estuar. Coast., 36, 491–507. doi: 10.1007/s12237-011-9455-x
- Parton WJ, Schimel DS, Cole SV, Ojima DS 1987: Analysis of factors controlling soil organic matter levels in great plains grasslands. Soil Sci. Soc. Am. J., 51, 1173–1179. doi: 10.2136/sssaj1987.03615995005100050015x
- Sahagian D, Melack J (eds) 1996: Global wetland distribution and functional characterization: Trace gases and the hydrologic cycle, Wetlands Workshop Report, Report from the joint GAIM-DIS-BAHC-IGAC-LUCC workshop, Santa Barbara, California.
- Suzuki S, Sakata H, Baba T, Kagiya S 2010: Effects of soil characteristics on plant zonation and species diversity in a cool-temperate brackish marsh. Eco-Engineering, 22, 19–26.
- Wang C, Lai DYF, Tong C, Wang W, Huang J, Huang J, Zeng C 2015: Variations in temperature sensitivity (Q10) of CH4 emission from a subtropical estuarine marsh in southeast China. PLoS ONE, 10, e0125227. doi: 10.1371/journal.pone.0125227