ABSTRACT
The increase in two controversial global environmental issues, climate warming and nitrogen (N) deposition, may have distinct effects on the processes and functioning of terrestrial ecosystems. Nutrient resorption is an important determinant of plant community nutrient dynamics, especially in nutrient-limited ecosystems, but information about N and phosphorus (P) resorption in alpine ecosystems is still lacking. A long-term simulated warming and exogenous N addition experiment initiated in July 2010 was conducted in an alpine meadow in Damxung County in northern Tibet. The experiment consisted of conditions of warming and no warming crossed with three N addition levels: 0 (CK), 20 (N20), and 40 (N40) kg N · ha−1 · year−1. With increasing N addition levels, the N content and the N/P ratio in plant leaves gradually increased, while the P limitation of plant growth was aggravated by N addition. The moderate N addition level (N20) increased plant N resorption efficiency (NRE), while the high N addition level (N40) had no effect on the NRE of Kobresia pygmaea or Anaphalis xylorhiza. N addition significantly increased the P resorption efficiency (PRE) in Stipa capillacea leaves. However, N addition did not change the community NRE or the community PRE. The soil N content decreased under the warming treatment. At the community level, warming significantly increased the NRE by 12% and 16%, and the PRE by 26% and 24% under the CK and N40 treatments, respectively. The NRE and PRE were higher in S. capillacea than in K. pygmaea and A. xylorhiza, especially at the high N addition level (38% and 45% higher NRE and 36% and 15% higher PRE compared to K. pygmaea and A. xylorhiza, respectively). Correlation analysis showed that the NRE and PRE in plant leaves were mainly mediated by soil inorganic N availability, and tended to decrease with increase of soil N availability, suggesting that N loss due to warming could induce changes in nutrient resorption in alpine ecosystems. The species-specific responses to N addition and the stronger competitive advantage of S. capillacea may change the community structure and subsequently affect the decomposition process in this alpine meadow under future global climate change scenarios.
1. Introduction
Increasing global warming and atmospheric nitrogen (N) deposition are important issues in global climate change. However, the effects of warming on the ecosystems also depend on the extent of N deposition (Zhang et al. Citation2015). N is one of the most important elements limiting terrestrial ecosystem productivity (Elser et al. Citation2007; LeBauer and Treseder Citation2008; Wedin and Tilman Citation1996). Global N deposition has more than tripled in the last century (Gruber and Galloway Citation2008; IPCC Citation2007) and is projected to increase an additional 2–3 times by the end of the century (Lamarque et al. Citation2005). Increases in global warming and N deposition will have large impacts on terrestrial ecosystems; however, there is still a lack of studies on how increasing climate warming and N deposition affect the structure and function of ecosystems in alpine meadows (Fu et al. Citation2015; Fu and Shen Citation2016).
Nitrogen and phosphorus (P) play important roles in regulating plant metabolism and growth (Vitousek et al. Citation1997). In natural ecosystems, N and P are often important elements limiting ecosystem primary production. In order to reduce their dependence on nutrient uptake in the current season, plants generally recover some mineral elements from senescent leaves, save the nutrients in storage organs and re-use them in next growing season (Jiang et al. Citation2012). Resorption efficiency (RE), defined as the percentage decrease in nutrient content between mature and senescent leaves, is often used to quantify the resorption capacity of plants (Jiang et al. Citation2012; Lü et al. Citation2013). Nutrient resorption is an important driving force of plant nutrient utilization strategies, and has important effects on plant litter quality and further decomposition processes. Meanwhile, ecological stoichiometry and nutrient resorption efficiency can reflect the soil nutrient supply condition (Chen et al. Citation2007). Therefore, nutrient resorption has important significance for plant population and community stability, as well as ecosystem nutrient cycling (Lü et al. Citation2013).
Because of the high altitude, ecosystem productivity on the Qinghai-Tibet Plateau is generally restricted by soil nutrient availability (Cao and Zhang Citation2001; Fu and Shen Citation2016). Therefore, nutrient recycling is of great importance for the maintenance of forage production in alpine meadow; however, little research has been conducted in this field (Fu and Shen Citation2016). Jiang et al. (Citation2012) reported that N and P resorption efficiencies in alpine plants on the northeast Qinghai-Tibet Plateau was at the high end of the global average range, because of the low N availability in alpine soils. This also suggested that further increases in atmospheric N deposition may have large impacts on nutrient resorption in alpine plants (Jiang et al. Citation2012). To a certain extent, this suggested relationship was verified in semi-arid temperate grasslands. As N addition levels in northern China increased, the N and P contents in plant senescent leaves significantly increased, but the N and P resorption efficiencies significantly decreased (Lü et al. Citation2013). These results showed that nutrient resorption is a key strategy involved in responding to changes in N availability in grasslands, and that it is especially important in N-limited alpine ecosystems. Meanwhile, plant nutrient resorption efficiency has significant impacts on the processes that occur after plant senescence, such as the chemical properties and decomposition processes in litter (Aerts et al. Citation2007). However, the evidence of the impact of climate change on nutrient resorption is still lacking. A study in peatlands in the subarctic region found that five years of warming slightly reduced plant leaf N content, but that plant species responded differently to climate warming (Aerts et al. Citation2007). The N content in Rubus chamaemorus (11.2 mg N · g−1) was approximately four times higher than that of Calamagrostis lapponica (3.0 mg N · g−1) in the control treatment, but R. chamaemorus (about 80%) had a higher N resorption efficiency than other species (about 60%) (Aerts et al. Citation2007), indicating that the effect of climate warming on nutrient resorption efficiency was species-specific. Different responses of species to climate change can lead to community structure changes, but few studies have been conducted on alpine meadows on the Qinghai-Tibet Plateau.
In recent decades, the temperature on the Qinghai-Tibetan Plateau has markedly increased (Zhang et al. Citation2013a). However, the effects of warming on alpine ecosystems may depend on the status of other factors, such as atmospheric N deposition (Chen et al. Citation2013). The N deposition rate also showed a significant increasing trend in the last several decades (Jia et al. Citation2014). The study of how alpine meadows respond to warming and N addition can help us to understand and further predict the responses of nutrient cycling to future climate change on the Qinghai-Tibet Plateau. However, to date, no estimations of nutrient resorption efficiency in response to warming and increasing N deposition have been reported in alpine ecosystems on the Qinghai-Tibetan Plateau (Fu and Shen Citation2017). Therefore, we conducted a manipulative experiment including simulated warming and N addition in an alpine meadow area starting in July 2010. Our previous results showed that warming changes the hydrothermal conditions of the alpine ecosystem, leading to an increase in evapotranspiration and a decrease in soil water content (Zong et al. Citation2013), which may have significant effects on soil N availability (Zhang et al. Citation2015). Both soil water and nutrient conditions could affect plant growth and nutrient resorption (Fu et al. Citation2018). Meanwhile, N enrichment could compensate for the decrease in soil inorganic N concentration induced by warming (Zhang et al. Citation2015; Fu and Shen Citation2017). All these changes result in significant interactions between warming and N enrichment. Therefore, we inferred that the following: (1) the soil N loss and soil water deficit induced by warming could result in an increase in nutrient resorption efficiency; (2) the soil N loss under warming conditions could be offset by N addition; therefore, warming and N addition may have compensative effects on nutrient resorption efficiency.
2. Materials and methods
2.1. Site description
This study was performed in an alpine meadow approximately 3 km north of Damxung County, Tibet Autonomous Region, China (91°05′E, 30°25′N). The site is located on the south-facing slope of the Nyainqentanglha Mountains at an altitude of 4,333 m above sea level (a.s.l.) in the mid-southern region of the Tibetan Plateau. A detailed description of the experimental site can be found in Jiang et al. (Citation2013) and Zong et al. (Citation2016). The climate at this site is characterized as the semi-arid continental type. The mean annual temperature is 1.3°C, with a minimum of −10.4°C in January and a maximum of 10.7°C in July. The annual precipitation is 477 mm, 85% of which falls between June and August. The annual potential evapotranspiration is 1725.7 mm (Shi et al. Citation2006), and the aridity index is 3.6 (annual potential evapotranspiration divided by annual precipitation). The soil is classified as Mat-Gryic Cambisol, corresponding to Gelic Cambisol, with a depth of approximately 0.3–0.5 m. The soil particle composition is 67.02% sand, 18.24% silt, and 14.74% clay (Fu et al. Citation2012). A detailed description of the soil properties can be found in the study by Zong et al. (Citation2014b). This alpine meadow is dominated by the sedges Kobresia pygmaea C.B. Clarke var. pygmaea, Carex montis-everestii, and the grass Stipa capillacea Keng. In addition, the meadow has also been invaded by the forb Anaphalis xylorhiza due to degradation resulting from overgrazing. The total atmospheric N deposition at the study site is approximately 10 kg N · ha−1 · year−1 (Zong et al. Citation2016).
2.2. Experimental design and treatment
The field manipulations consisted of two warming treatments [year-round warming (YW) and no warming (NW)], crossed with three N addition treatments [control (CK), 20 (N20) and 40 (N40) kg N · ha−1 · year−1], and the treatment combinations were organized in a randomized block design with five replicates for each treatment. N20 and N40 were roughly equal to 2 and 4 times the current N deposition rate (10 kg N· ha−1 · year−1 in the Damxung region) (Zong et al. Citation2016), respectively. N40 was designed to approximately mimic the projected atmospheric deposition rate in this region by 2050 (Galloway et al. Citation2004). We followed the methods of the International Tundra Experiment (ITEX) and applied passive warming open-top chambers (OTCs) to artificially generate warming conditions. The OTCs, with 100-cm diameter top openings, 140-cm diameter bottom openings, 40 cm in height and a bottom area of 1.54 m2, are made of 3-mmthick polycarbonate plastic. This material has high solar transmittance in visible and ultraviolet wavelengths (about 90%) (De Frenne et al. Citation2010). In the N addition plots, N was applied as ammonium nitrate (NH4NO3) in aqueous solution, two times during growing season using sprayers, with half added during the early growing season in early June and the remaining half added in early August. The water added to each plot represented a 6-mm increase in precipitation, approximately equal to 1.2% of the annual precipitation, which was well within the magnitude of inter-annual variations (Zong et al. Citation2013). The same amount of water was also sprayed onto the no N addition plots.
We set up the warming plots in early-July 2010 and synchronously monitored the warming effects every half hour on year-round air temperature, soil moisture and temperature at a depth of 5 cm by a HOBO weather station (Onset Inc., Bourne, MA, USA). The buffer distance between replicates was at least 3 m. Rainfall data were obtained from the national weather station in Damxung County (91°06′ E, 30°29′ N, 4288 m a.s.l., 3 km away from the study site) and downloaded from the China Meteorological Data Sharing Service System (http://cdc.cma.gov.cn). In 2013, the OTC warming devices increased air and soil temperature by 2.2 and 1.7°C, respectively, while they reduced soil moisture by 4.3% (v/v). There was 447.3 mm precipitation in 2013 ().
2.3. Plant materials sampling and laboratory analysis
Soil samples were collected in mid-August, 2013. After passing the samples through a 2-mm sieve to remove roots, gravel, and stones, NO3–N and NH4+-N were extracted from half of the composite soil samples using 2.0 mol· L−1 KCl, filtered, and analyzed on a continuous flow analyzer within 48 h (AA3, SEAL Analytical, Norderstedt, Germany). The other half of the soil xsamples were dried, pulverized using a ball mill and passed through a 100-mesh sieve for further analysis. Soil total nitrogen (TN) was determined by the Kjeldahl method and total phosphorus (TP) was digested by sulfuric acid (H2SO4) and perchloric acid (HClO4) and analyzed on a continuous flow analyzer (AA3, SEAL Analytical, Norderstedt, Germany).
A representative set of mature leaves of the three dominant plant species were sampled from all plots in mid-August 2013, i.e., the fourth year of the warming treatment. In each plot, we randomly collected about 5 g of mature and fully expanded live leaves of each dominant plant species from three functional groups, the sedge K. pygmaea C.B. Clarke var. pygmaea, the grass S. capillacea Keng, and the forb A. xylorhiza. These three species accounted for more than 75% of total aboveground biomass in this alpine meadow; therefore, changes to these three species can represent changes to the whole (Zong et al. Citation2016). In late September 2013, senesced but still attached plant leaves were collected from the same plots (Jiang et al. Citation2012). The samples were oven dried at 65°C in the laboratory for at least 48 h until they reached to a constant weight. The additional analysis methods were the same as those used for the soil samples.
2.4. Data calculation and statistical analysis
The nitrogen resorption efficiency (NRE) is calculated as follows (Aerts et al. Citation2007):
where Nmature and Nsenesced are the N content in mature and senesced leaves, respectively. P resorption efficiency (PRE) was calculated by the same method.
Statistical analyses were performed using the SPSS 16.0 software package (SPSS, Chicago, IL, USA). Three-way ANOVAs followed by Duncan’s multiple comparison tests were applied to assess the effects of warming, N addition and plant species on N and P contents and the N/P ratio in mature plant leaves, as well as plant NRE and PRE. Linear regression analyses were performed to test the dependence of plant N and P and the N/P ratio on N addition levels. We also analyzed the association of plant leaf and soil nutrient content with NRE and PRE. The value of P < 0.05 was considered statistically significant. All the figures were produced using Origin Pro 8.0 (OriginLab Corporation, Northampton, MA, USA).
3. Results
3.1. Nitrogen and phosphorus content in plant leaves
There were significant differences in N and P contents and the N/P ratio among different dominant species (). P content was higher in A. xylorhiza leaves than in K. pygmaea and S. capillacea leaves (, while the N/P ratio was higher in K. pygmaea leaves than in S. capillacea and A. xylorhiza leaves. Meanwhile, the responses of N and P contents and the N/P ratio to warming and N addition differed by species ().
Table 1. Statistical analysis on the effects of warming (W), N addition (N), and species (S) on the N and P resorption efficiency in plant leaves.
Figure 1. Effects of warming and N addition on the N (A–C) and P (E–G) and N/P (I–K) content in mature leaves of three plant species, and the responses of N (D), P (H), and the N/P ratio (L) in plant leaves to N addition levels. Only significant differences were showed between warming treatments. Ns, *, **, *** represent P ≥ 0.05, P < 0.05, and P < 0.01, P < 0.001 among warming treatments under the same N treatment. Different lowercase letters represent significant differences among N levels under the same warming treatment. NW and YW represent no-warming and year-round warming treatments, respectively. Below was the same.
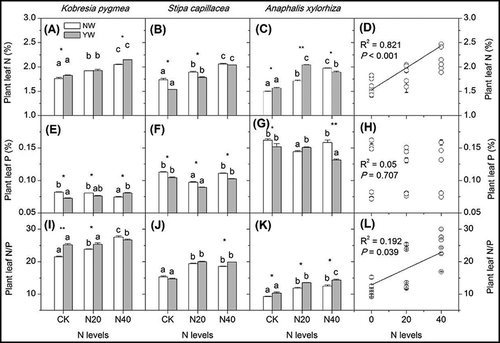
N addition significantly increased N content in plant leaves (, P < 0.05), but the P content in plant leaves did not show a consistent pattern (). N addition did not change the community P content (). The N/P ratio in individual species showed a tendency to increase gradually with increasing levels of N addition (, indicating that P limitation of plant growth was aggravated by N addition.
Warming significantly increased the N content in plant leaves in K. pygmaea and A. xylorhiza leaves, while S. capillacea showed an opposite trend in the CK and N20 treatment (); this indicated that the three plant species responded differently to the warming treatment. But warming had no effect on the plant community N and P contents (). The effects of warming on the P content in plant leaves displayed a different tendency, as warming significantly decreased the P content in these three species (. Therefore, the N/P ratio was increased by warming in most species and with most N addition treatments.
3.2. Soil nitrogen and phosphorus content
The effects of N addition on soil nutrient content varied in different warming treatments. N addition significantly increased soil total N and inorganic N as well as the N/P ratio, especially at the high N addition level (N40) with no warming, while N addition did not change soil N content or the N/P ratio with warming (. In addition, N addition did not change soil P content regardless of the warming treatment ().
Figure 2. Effects of warming and N addition on soil total N and P contents, inorganic N as well as the N/P ratio. The significance and abbreviations can be found in .
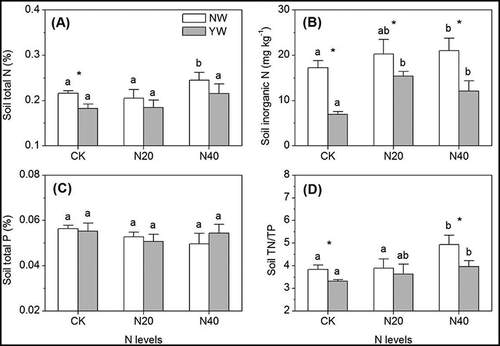
Warming reduced soil total N and inorganic N content, as well as the N/P ratio, but the difference was significant only for soil total N in the control (). However, warming did not change soil total P content regardless of the N addition level ().
3.3. Plant nitrogen and phosphorus resorption efficiencies
The effects of N addition on NRE and PRE varied in the different plant species (, ). The moderate N level (N20) increased the plant NRE, while the high N level (N40) had no effect on NRE of K. pygmaea on that of A. xylorhiza (). N addition significantly increased the PRE in S. capillacea leaves (). However, N addition did not change the community NRE and PRE ().
Table 2. Statistical analysis on the effects of warming (W), N addition (N), and plant species (S) on the N and P resorption efficiency in plant leaves. Leaf NRE and PRE represent the N and P resorption efficiency.
Figure 3. Effects of warming and N addition on leaf N (A–C) and P (D–F) resorption efficiency in different plants. The significance and abbreviations can be found in .
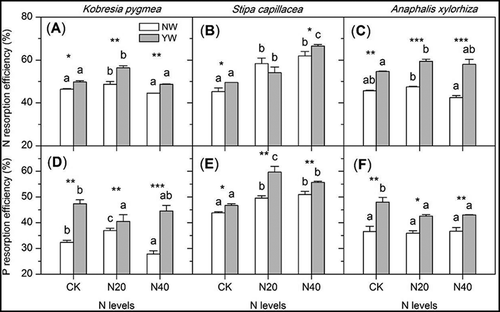
Warming significantly increased the NRE and the PRE in all plant species at most N addition levels (). However, warming did not change the community NRE and PRE under moderate N addition conditions (N20) ().
There were significant differences in NRE and PRE among the different dominant species (, P < 0.001). Both the NRE and PRE of S. capillacea were higher than those of the other two dominant species, especially with the high levels of N addition (N40). This indicated that the changes in nutrient resorption and utilization were species-specific, which may lead to community structure change.
3.4. Relationships between plant and soil nutrient content and N and P resorption efficiencies
Regression analysis showed that both the NRE and the PRE in plant leaves were influenced by P content in the mature plant leaves (, indicating that the P content in plant leaves after the exogenous addition of N was more important than the N content in regulating nutrient resorption. However, neither NRE nor PRE were significant correlated with soil total N and P contents (, while both NRE and PRE showed tendencies to decrease with increasing soil inorganic N content (, indicating that the plant NRE and PRE were mainly affected by soil available N rather than soil total nutrient content. We also concluded that high levels of N addition are not beneficial for the maintenance of plant nutrient resorption capacity.
Figure 4. Dependence of plant N (NRE) and P (PRE) resorption efficiencies on plant leaf nutrient content. The correlation curves represent significant relationships between nutrient resorption efficiency and plant leaf nutrient content. Open and solid circles represent NW and YW treatments, respectively.
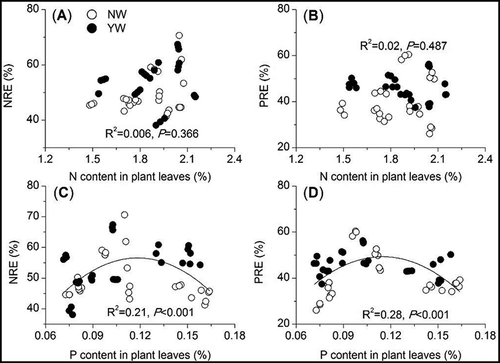
4. Discussion
4.1. Effects of warming and N addition on nutrient stoichiometry
N and P are two important elements that limit plant growth and development in natural ecosystems. The N/P ratio is not only important factor for determining the structure and function of plant communities but also an indicator of the nutrient conditions (Chen et al. Citation2007; Lü et al. Citation2013). Generally, a higher N/P ratio means that N is abundant while P is relatively deficient, which suggests that plant growth is limited by P availability; a lower N/P ratio means that P is abundant while N is relatively deficient, which suggests that plant growth is limited by the availability of N (Gusewell Citation2004; Gusewell et al. Citation2003; Koerselman and Meuleman Citation1996). A previous study reported that plant growth is mainly limited by N availability when N/P < 14 and limited by P when N/P > 16 (Gusewell et al. Citation2003), but these thresholds may vary in different ecosystems. This study found that the leaf N content increased with increasing N addition, and that the leaf N/P ratio also showed a tendency to increase (). Under the CK and N40 treatments, the N/P ratio was 15.3 and 18.5 in S. capillacea; 21.5 and 27.6 in K. pygmaea; and 9.2 and 12.5 in A. xylorhiza, respectively, indicating that (1) N addition caused the growth of S. capillacea and K. pygmaea to be more restricted by P and (2) A. xylorhiza was more tolerant for high N availability. The effects of N addition on A. xylorhiza were weaker than on the other species, and the growth was still restricted by N availability. These effects were consistent with those observed in the Songnen meadow in northeast China (Zhang et al. Citation2013b).
In this study, N addition increased the plant and soil N/P ratios under in the absence of warming. Generally, increasing N uptake by plants would simultaneously increase P uptake because plants always maintain a relatively stable N/P ratio. However, continuous N addition would lead to excessive consumption of soil P and an increase in the plant N/P ratio, resulting in P limitation for plant production (Limpens et al. Citation2004; Menge and Field Citation2007). Soil P changes due to N addition are dependent on the balance between the soil supply and the plant uptake, which is attributed to the increase of the soil organic matter conversion rate (Treseder and Vitousek Citation2001), changes in phosphatase levels (Marklein and Houlton Citation2012; Olander and Vitousek Citation2000) and mycorrhizal activity (Rowe et al. Citation2008). This can explain the observations that exogenous N input can only enhance plant growth within a certain range (Bowman et al. Citation2006; Zong et al. Citation2016).
Our study revealed that warming increased plant N content (), which was attributed to the increase in soil N availability. Due to the high altitude, low temperatures generally limit soil microorganism decomposition processes and restrict soil organic matter mineralization; therefore, soil N release is very slow, and plant growth is restricted by N availability in alpine ecosystems (Bowman et al. Citation1993; Cao and Zhang Citation2001). In the growing season when there are frequent rainfall events, warming generally enhances the soil N mineralization rate, and the increase of soil N availability subsequently improves the plant leaf N content. In this study, warming tended to decrease the plant P content, especially for S. capillacea (); consequently, the N/P ratio was higher in the warming treatments than that in no-warming treatments. The N/P ratios were 15.3 and 14.7 in S. capillacea with N addition under NW and YW, respectively, while in the N40 treatment, the N/P ratios were 18.6 and 19.9 in NW and YW, respectively. The leaf N/P ratio increased in the warming treatment, which indicated that warming led to stronger P limitation because high N mineralization rate in the warming treatment resulted in excessive consumption of P (Zhang et al. Citation2013b). Increases in the soil nutrient availability could promote plant growth and thus dilute the P content and increase the N/P ratio.
4.2. Effects of warming and N addition on nutrient resorption efficiency
Due to leaf shrinkage and mass loss during the process of plant leaf senescence, the calculation of nutrient resorption efficiency has some shortcomings. A previous study reported that leaf shrinkage and mass loss may lead to an underestimation of nutrient resorption. Leaf shrinkage could lead to an average underestimation of 6% compared to when area-based nutrient content was used to calculate nutrient resorption efficiency (van Heerwaarden et al. Citation2003b), which suggests that the nutrient resorption efficiency in alpine plants could be even higher than measured in this study. Our previous study showed that the size of the leaves during the process of senescence may not change much in arid and semiarid climates (Zhao et al. Citation2017). Furthermore, the unit mass of nutrient content that was used in this study rather than the unit area is also favorable for reducing the impact of leaf shrinkage. Therefore, this study may not lead to much underestimation of nutrient resorption.
This study showed that the N and P resorption efficiencies in these three dominant plant species had ranges of 45.3‒46.3% and 32.4‒43.8%, respectively (), which were close to the global average (Aerts Citation1996; Killingbeck Citation1996). On a large spatial scale, the nutrient resorption efficiency reflects the soil nutrient conditions of the local environment (Kobe et al. Citation2005) and the plant adaptation strategies to the nutrient conditions in different environments (Jiang et al. Citation2012). Meanwhile, we found that the nutrient resorption efficiency was different among plants from different functional types. The N and P resorption efficiencies of S. capillacea were higher than those of sedges and forbs. This was also verified by the nutrient use efficiencies. As one of dominant functional groups in this semi-arid alpine meadow, grasses always have higher nutrient use efficiencies than other species (Walker et al. Citation1994; Zong et al. Citation2014a). A higher nutrient resorption efficiency is an effective strategy for the successful competition of this functional group with the other coexistent species in a nutrient-poor environment (Aerts Citation1996). Grasses are very sensitive to N addition. As an active nutrient, N can easily be absorbed and utilized in a short time, thus increasing plant biomass. In recent decades, due to the influence of human activities, atmospheric N deposition has significantly increased, and nitrophilous grasses have a stronger competitive advantage over other species, which leads to plant community structure changes in alpine meadows. At the same time, we found that N and P resorption efficiencies in K. pygmaea did not increase continuously with the increasing N addition rate (). Although Kobresia is a typical colonization functional group in the alpine region, its N use efficiency is not especially high (Walker et al. Citation1994). The high N addition level alone had harmful effects on this functional group, while the addition of the combination of N and P significantly increased the aboveground productivity of sedges (Zong et al. Citation2014a).
Regression analysis showed that both the NRE and the PRE in plant leaves were mainly influenced by the P content in plant mature leaves, indicating that the P availability changed by the exogenous N addition was more important in regulating plant nutrient resorption. Although soil P content was not affected by the addition of N, the N/P ratios in individual species and in the soil showed tendencies to increase with increasing N levels; this indicated that P limitation for plant growth was aggravated by the addition of N, which was consistent with the findings of another study (Zhang et al. Citation2013b). Because N addition facilitates plant growth and plants need to maintain relatively stable N/P ratios, the absorption and utilization of soil P are enhanced (Lilleskov et al. Citation2002; Menge and Field Citation2007). Furthermore, P in ecosystems is difficult to decompose, and absorbed P cannot be quickly released into the soil and re-used by plants. Therefore, N availability was sufficient after the addition of N, and P availability became more important in regulating plant nutrient resorption.
Exogenous N addition significantly increased the soil nutrient availability, and plants could directly absorb the available soil nutrients and reduce their dependence on endogenous nutrient resorption (Lü et al. Citation2013). Most studies found that N fertilization reduced nutrient resorption efficiencies in marsh (van Heerwaarden et al. Citation2003a), tundra (Soudzilovskaia et al. Citation2007), tropical savanna (Kozovits et al. Citation2007), temperate steppe (Lü et al. Citation2013), and forest (Li et al. Citation2010) ecosystems. However, another study found that N fertilization did not significantly change plant nutrient resorption efficiency (Huang et al. Citation2008). Our study found that the NRE and the PRE tended to decrease with increasing soil inorganic N content (). This was consistent with previous results (Huang et al. Citation2008; Lü et al. Citation2013). The N levels in this experiment were significantly lower than those in Huang et al. (Citation2008) and Lü et al. (Citation2013). The maximum N levels were 640 and 560 kg N ha−1 · year−1 in those two studies, respectively, while the maximum N level in this study was only 40 kg N ha−1 · year−1. Despite low N levels compared with previous studies, the nutrient resorption efficiency still presented an inverse association with soil inorganic N content. Alpine ecosystems are strongly restricted by soil N availability due to the high altitude and climatic factors, and alpine ecosystems are more sensitive to N addition. Our previous study estimated that the critical load of N addition in this alpine meadow was 8.8–12.7 kg N ha−1 · year−1 (Zong et al. Citation2016), lower than those in other grasslands. N addition above this critical load would significantly affect the ecosystem primary production. A high level of N addition could change the soil physio-chemical properties, such as soil acidification, cation loss, buffering capacity decline, and Al3+ release (Stevens et al. Citation2010; Yang et al. Citation2012), which could negatively affect plant production and soil microbial activities. Therefore, high levels of N addition could reduce plant nutrient resorption efficiencies, as observed in the above two studies.
5. Conclusions
In this study, we quantified the effects of warming and N addition on N and P contents and the nutrient resorption efficiency of three dominant species in an alpine meadow. With increasing N addition levels, the N content and the N/P ratio in plant leaves gradually increased, which indicated that N addition aggravated P limitation for alpine plants. We inferred that combined addition of N and P could be a better choice for the restoration of degraded alpine meadow in this semi-arid area. As it is accompanied by the excessive consumption of soil P, warming can lead to soil N loss and may have large effects on ecosystem functioning and stability. Species-specific responses to warming and N addition as well as different resorption efficiencies among various dominant species could result in plant community structure changes under future global climate change scenarios. In addition, N and P resorption efficiencies in plant leaves tended to decrease with increasing soil N availability, demonstrating that high levels of N addition are not conducive to the maintenance of ecosystem stability or the increase of plant productivity. Soil N loss resulting from warming was the main cause for the increase in plant nutrient resorption in this alpine meadow.
Supplementary_materials.docx
Download MS Word (130.4 KB)Acknowledgments
We are grateful to the stuff in Damxung grassland station for their help in maintenance of experimental instruments. We also express our thanks to the experts from American Journal Experts (https://www.aje.com/#) to improve the language of this manuscript. This work was jointly supported by the National Key Research and Development Program (Grant No. 2016YFC0501803), the West Light Foundation of the Chinese Academy of Sciences (2015), the National Natural Science Foundation of China (Grant No. 41703079 and 31470506) and the Youth Innovation Research Team Project (Grant No. LENOM2016Q0004).
Additional information
Funding
References
- Aerts R 1996: Nutrient resorption from senescing leaves of perennials: are there general patterns? J. Ecol. 84, 597–608. doi:10.2307/2261481
- Aerts R, Cornelissen JH, van Logtestijn RS, Callaghan TV 2007: Climate change has only a minor impact on nutrient resorption parameters in a high-latitude peatland. Oecologia. 151, 132–139. doi:10.1007/s00442-006-0575-0
- Bowman WD, Gartner JR, Holland K, Wiedermann M 2006: Nitrogen critical loads for alpine vegetation and terrestrial ecosystem response: are we there yet? Ecol. Appl. 16, 1183–1193. doi:10.1890/1051-0761(2006)016[1183:NCLFAV]2.0.CO;2
- Bowman WD, Theodose TA, Schardt JC, Conant RT 1993: Constraints of nutrient availability on primary production in two alpine tundra communities. Ecology. 74, 2085–2097. doi:10.2307/1940854
- Cao GM, Zhang JX 2001:Soil Nutrition and Substance Cycle of Kobresia Meadow, Beijing, Science Press.
- Chen F, Hu X, Ge G 2007: Leaf N:P stoichiometry and nutrient resorption efficiency of Ophiopogon japonicus in Nanchang City. Acta Prataculturae Sinica. 16, 47–54.
- Chen H, Zhu Q, Peng C et al. 2013: The impacts of climate change and human activities on biogeochemical cycles on the Qinghai-Tibetan Plateau. Global Change Biol. 19, 2940–2955. doi:10.1111/gcb.12277
- De Frenne P, De Schrijver A, Graae BJ, Gruwez R, Tack W, Vandelook F, Hermy M, Verheyen K 2010: The use of open-top chambers in forests for evaluating warming effects on herbaceous understorey plants. Ecol. Res. 25, 163–171. doi:10.1007/s11284-009-0640-3
- Elser JJ, Bracken ME, Cleland EE, Gruner DS, Harpole WS, Hillebrand H, Ngai JT, Seabloom EW, Shurin JB, Smith JE 2007: Global analysis of nitrogen and phosphorus limitation of primary producers in freshwater, marine and terrestrial ecosystems. Ecol. Lett. 10, 1135–1142. doi:10.1111/j.1461-0248.2007.01113.x
- Fu G, Shen Z 2016: Response of alpine plants to nitrogen addition on the Tibetan Plateau: a meta-analysis. J. Plant Growth Regul. 35, 974–979. doi:10.1007/s00344-016-9595-0
- Fu G, Shen Z 2017: Response of alpine soils to nitrogen addition on the Tibetan Plateau: a meta-analysis. Appl. Soil Ecol. 114, 99–104. doi:10.1016/j.apsoil.2017.03.008
- Fu G, Shen Z, Sun W, Zhong Z, Zhang X, Zhou Y 2015: A meta-analysis of the effects of experimental warming on plant physiology and growth on the Tibetan Plateau. J. Plant Growth Regul. 34, 57–65. doi:10.1007/s00344-014-9442-0
- Fu G, Shen Z, Zhang X 2018: Increased precipitation has stronger effects on plant production of an alpine meadow than does experimental warming in the Northern Tibetan Plateau. Agri. Forest Meteor. 249, 11–21. doi:10.1016/j.agrformet.2017.11.017
- Fu G, Shen Z, Zhang X, Zhou Y, Zhang Y 2012: Response of microbial biomass to grazing in an alpine meadow along an elevation gradient on the Tibetan Plateau. Eur. J. Soil Biol. 52, 27–29. doi:10.1016/j.ejsobi.2012.05.004
- Galloway JN, Dentener FJ, Capone DG et al. 2004: Nitrogen cycles: past, present, and future. Biogeochemistry. 70, 153–226. doi:10.1007/s10533-004-0370-0
- Gruber N, Galloway JN 2008: An earth-system perspective of the global nitrogen cycle. Nature. 451, 293–296. doi:10.1038/nature06592
- Gusewell S 2004: N: P ratios in terrestrial plants: variation and functional significance. New Phytol. 164, 243–266. doi:10.1111/j.1469-8137.2004.01192.x
- Gusewell S, Koerselman W, Verhoeven JTA 2003: Biomass N: P ratios as indicators of nutrient limitation for plant populations in wetlands. Ecol. Appl. 13, 372–384. doi:10.1890/1051-0761(2003)013[0372:BNRAIO]2.0.CO;2
- Huang J, Zhu X, Yuan Z, Song S, Li X, Li L 2008: Changes in nitrogen resorption traits of six temperate grassland species along a multi-level N addition gradient. Plant Soil. 306, 149–158. doi:10.1007/s11104-008-9565-9
- IPCC 2007: Intergovernmental panel on climate change (IPCC), Climate change. The physical science basis. The Fourth Assessment Report of Working Group.
- Jia Y, Yu G, He N, Zhan X, Fang H, Sheng W, Zuo Y, Zhang D, Wang Q 2014: Spatial and decadal variations in inorganic nitrogen wet deposition in China induced by human activity. Sci. Rep. 4, 4763.
- Jiang C, Yu G, Li Y, Cao G, Yang Z, Sheng W, Yu W 2012: Nutrient resorption of coexistence species in alpine meadow of the Qinghai-Tibetan Plateau explains plant adaptation to nutrient-poor environment. Ecol. Eng. 44, 1–9. doi:10.1016/j.ecoleng.2012.04.006
- Jiang J, Zong N, Song M, Shi P, Ma W, Fu G, Shen Z, Zhang X, Ouyang H 2013: Responses of ecosystem respiration and its components to fertilization in an alpine meadow on the Tibetan Plateau. Eur. J. Soil Biol. 56, 101–106. doi:10.1016/j.ejsobi.2013.03.001
- Killingbeck KT 1996: Nutrients in senesced leaves: keys to the search for potential resorption and resorption proficiency. Ecology. 77, 1716–1727. doi:10.2307/2265777
- Kobe RK, Lepczyk CA, Iyer M 2005: Resorption efficiency decrease with increasing green leaf nutrients in a global data set. Ecology. 86, 2780–2792. doi:10.1890/04-1830
- Koerselman W, Meuleman AFM 1996: The vegetation N:P ratio: a new tool to detect the nature of nutrient limitation. J. Appl. Ecol. 33, 1441–1450. doi:10.2307/2404783
- Kozovits AR, Bustamante MMC, Garofalo CR, Bucci S, Franco AC, Goldstein G, Meinzer FC 2007: Nutrient resorption and patterns of litter production and decomposition in a Neotropical Savanna. Funct. Ecol. 21, 1034–1043. doi:10.1111/j.1365-2435.2007.01325.x
- Lamarque JF, Hess P, Emmons L, Buja L, Washington W, Granier C 2005: Tropospheric ozone evolution between 1890 and 1990. J. Geophys. Res-Atmos. 110(D8), 1–15. doi:10.1029/2004jd005537.
- LeBauer DS, Treseder KK 2008: Nitrogen limitation of net primary productivity in terrestrial ecosystems is globally distributed. Ecology. 89, 371–379. doi:10.1890/06-2057.1
- Li X, Zheng X, Han S, Zheng J, Li T 2010: Effects of nitrogen additions on nitrogen resorption and use efficiencies and foliar litterfall of six tree species in a mixed birch and poplar forest, northeastern China. Can. J. Forest Res. 40, 2256–2261. doi:10.1139/X10-167
- Lilleskov EA, Fahey TJ, Horton TR, Lovett GM 2002: Belowground ectomycorrhizal fungal community change over a nitrogen deposition gradient in Alaska. Ecology. 83, 104–115. doi:10.1890/0012-9658(2002)083[0104:BEFCCO]2.0.CO;2
- Limpens J, Berendse F, Klees H 2004: How phosphorus availability affects the impact of nitrogen deposition on Sphagnum and vascular plants in bogs. Ecosystems. 7, 793–804. doi:10.1007/s10021-004-0274-9
- Lü X, Reed S, Yu Q, He N, Wang Z, Han X 2013: Convergent responses of nitrogen and phosphorus resorption to nitrogen inputs in a semiarid grassland. Global Change Biol. 19, 2775–2784. doi:10.1111/gcb.12235
- Marklein AR, Houlton BZ 2012: Nitrogen inputs accelerate phosphorus cycling rates across a wide variety of terrestrial ecosystems. New Phytol. 193, 696–704. doi:10.1111/j.1469-8137.2011.03967.x
- Menge DNL, Field CB 2007: Simulated global changes alter phosphorus demand in annual grassland. Global Change Biol. 13, 2582–2591. doi:10.1111/j.1365-2486.2007.01456.x
- Olander LP, Vitousek PM 2000: Regulation of soil phosphatase and chitinase activity by N and P availability. Biogeochemistry. 49, 175–190. doi:10.1023/A:1006316117817
- Rowe EC, Smart SM, Kennedy VH, Emmett BA, Evans CD 2008: Nitrogen deposition increases the acquisition of phosphorus and potassium by heather Calluna vulgaris. Environ. Pollut. 155, 201–207. doi:10.1016/j.envpol.2007.12.008
- Shi P, Sun X, Xu L, Zhang X, He Y, Zhang D, Yu G 2006: Net ecosystem CO2 exchange and controlling factors in a steppe-Kobresia meadow on the Tibetan Plateau. Sci. China Ser. D-Earth Sci. 49, 207–218. doi:10.1007/s11430-006-8207-4
- Soudzilovskaia NA, Onipchenko VG, Cornelissen JHC, Aerts R 2007: Effects of fertilisation and irrigation on ‘foliar afterlife’ in alpine tundra. J. Veget. Sci. 18, 755–766. doi:10.1111/j.1654-1103.2007.tb02591.x
- Stevens CJ, Dupre C, Dorland E et al. 2010: Nitrogen deposition threatens species richness of grasslands across Europe. Environ. Pollut. 158, 2940–2945. doi:10.1016/j.envpol.2010.06.006
- Treseder KK, Vitousek PM 2001: Effects of soil nutrient availability on investment in acquisition of N and P in Hawaiian rain forests. Ecology. 82, 946–954. doi:10.1890/0012-9658(2001)082[0946:EOSNAO]2.0.CO;2
- van Heerwaarden LM, Toet S, Aerts R 2003a: Nitrogen and phosphorus resorption efficiency and proficiency in six sub-arctic bog species after 4 years of nitrogen fertilization. J. Ecol. 91, 1060–1070. doi:10.1046/j.1365-2745.2003.00828.x
- van Heerwaarden LM, Toet S, Aerts R 2003b: Current measures of nutrient resorption efficiency lead to a substantial underestimation of real resorption efficiency: facts and solutions. Oikos. 101, 664–669. doi:10.1034/j.1600-0706.2003.12351.x
- Vitousek PM, Aber JD, Howarth RW, Likens GE, Matson PA, Schindler DW, Schlesinger WH, Tilman DG 1997: Human alteration of the global nitrogen cycle: sources and consequences. Ecol. Appl. 7, 737–750.
- Walker MD, Webber PJ, Arnold EH, Ebert-May D 1994: Effects of interannual climate variation on aboveground phytomass in alpine vegetation. Ecology. 75, 393–408. doi:10.2307/1939543
- Wedin DA, Tilman D 1996: Influence of nitrogen loading and species composition on the carbon balance of grasslands. Science. 274, 1720–1723. doi:10.1126/science.274.5293.1720
- Yang Y, Ji C, Ma W, Wang S, Wang S, Han W, Mohammat A, Robinson D, Smith P 2012: Significant soil acidification across northern China’s grasslands during 1980s-2000s. Global Change Biol. 18, 2292–2300. doi:10.1111/j.1365-2486.2012.02694.x
- Zhang G, Zhang Y, Dong J, Xiao X 2013a: Green-up dates in the Tibetan Plateau have continuously advanced from 1982 to 2011. Proc. Natl. Acad. Sci. 110, 4309–4314. doi:10.1073/pnas.1210423110
- Zhang N, Guo R, Song P, Guo J, Gao Y 2013b: Effects of warming and nitrogen deposition on the coupling mechanism between soil nitrogen and phosphorus in Songnen Meadow Steppe, northeastern China. Soil Biol. Biochem. 65, 96–104. doi:10.1016/j.soilbio.2013.05.015
- Zhang X, Shen Z, Fu G 2015: A meta-analysis of the effects of experimental warming on soil carbon and nitrogen dynamics on the Tibetan Plateau. Appl. Soil Ecol. 87, 32–38. doi:10.1016/j.apsoil.2014.11.012
- Zhao G, Shi P, Wu J, Xiong D, Zong N, Zhang X 2017: Foliar nutrient resorption patterns of four functional plants along a precipitation gradient on the Tibetan Changtang Plateau. Ecol Evol. 1–12. doi:10.1002/ece3.3283
- Zong N, Shi P, Jiang J, Song M, Xiong D, Ma W, Fu G, Zhang X, Shen Z 2013: Responses of ecosystem CO2 fluxes to short-term experimental warming and nitrogen enrichment in an alpine meadow, northern Tibet Plateau. Sci. World J. Article ID 415318. doi:10.1155/2013/415318
- Zong N, Shi P, Niu B, Jiang J, Song M, Zhang X, He Y 2014a: Effects of nitrogen and phosphorous fertilization on community structure and productivity of degraded alpine meadows in northern Tibet, China. Chin. J. Appl. Ecol. 25, 3458–3468.
- Zong N, Shi P, Song M, Zhang X, Jiang J, Chai X 2016: Nitrogen critical loads for an alpine meadow ecosystem on the Tibetan Plateau. Environ. Manage. 57, 531–542. doi:10.1007/s00267-015-0626-6
- Zong N, Song M, Shi P, Jiang J, Zhang X, Shen Z 2014b: Timing patterns of nitrogen application alter plant production and CO2 efflux in an alpine meadow on the Tibetan Plateau, China. Pedobiologia. 57, 263–269. doi:10.1016/j.pedobi.2014.08.001