ABSTRACT
Inoculation with arbuscular mycorrhizal fungi (AMF) can increase the growth of host plants, especially under condition of low phosphate (P) availability. Although this effect is shown relatively easily in simplified systems such as pot experiments, it is often hard to show in the field because of complicating factors such as competition with indigenous AMF. We conducted an AMF inoculation experiment with three Japanese soybean cultivars (Enrei, Misuzudaizu, and Akishirome) in an allophanic (Umbric Silandic) Andosol field under the long-term selective application of major nutrients (NPK and -P) and bare fallow. In the inoculation plots, introduced AMF were well colonized in soybean roots at flowering stage. In the -P plots, inoculation tended to increase the shoot dry weight of all the three soybean cultivars; this effect remained until harvest. Although a significant difference is not recognized, there was a tendency of residual effect on Enrei in the following year. In the NPK plots, inoculation did not significantly increase the shoot dry weight. We thought that in the -P plots, the long-term selective application of N and K and the long-term maintenance of bare fallow created the soil conditions of low P availability and poor native AMF. Thus, introduced AMF can benefit soybean growth and yield in the soil with low competitor AMF density and low phosphate availability.
1. Introduction
Phosphorus (P) is a crucial nutrient for plant growth, but its availability from roots is usually limited. Arbuscular mycorrhizal fungi (AMF) are widespread root fungal symbionts of the vast majority of land plants, including many crops. The symbiosis plays an important role in enhancing the growth of host plants by supporting P uptake through a hyphal network that emerges from the host roots. As well as P, the hyphal networks take in various nutrients, e.g., nitrogen (N), potassium (K), and some micronutrients, so AMF symbiosis is important in crop production (Azaizeh et al. Citation1995; Clark and Zeto Citation2000; Smith and Read Citation2008).
It is well known that AMF inoculation can increase the growth of host plants, especially under conditions of low P availability (Smith and Read Citation2008). Successful pot experiments are numerous. Although the effects of AMF inoculation under field conditions have previously been evaluated (Pellegrino et al. Citation2011; Ortas Citation2012), they are not clear, likely because factors such as native AMF (Klironomos Citation2003; Fernández et al. Citation2011), soil P availability (Mosse et al. Citation1973), soil type (Daft and Hacskaylo Citation1977), and cultivar (Khalil et al. Citation1994; Khalil et al. Citation1999) all influence inoculation effectiveness in the field.
Field experiments could be complicated by competition with native AMF that are better adapted to the soil environment than introduced AMF. Klironomos (Citation2003) reported that the native AMF negated any benefits of introduced AMF. In addition, the typical application of P fertilizer in excess of crop requirements results in the accumulation of P in the soil, which can disrupt the symbiotic relationship between crops and AMF (Martensson and Carlgren Citation1994; Thingstrup et al. Citation1998).
In this study, we conducted an AMF inoculation experiment with soybeans in NPK and -P plots in an experimental field managed with the long-term selective application of major nutrients and bare fallow. We expected that the conditions without host plants for a long-time period would create a low density of native AMF in the soil, and long-term -P treatment would be appropriate for the experiment from the point of view of nutrient status. In the case of legumes including soybeans, AMF symbiosis can promote not only plant growth but also nitrogen fixation in P-deficient soils (Chalk et al. Citation2006). As the effectiveness of AMF symbiosis is highly dependent on host plant genotype (Tawaraya Citation2003), we grew three soybean cultivars which showed mid-to-high responsiveness to AMF by our previous pot experiment (Hayashi et al. unpublished data). To evaluate the inoculum effect on these cultivars, we measured AMF colonization and temporal change of plant growth. We also examined residual effects of the previous year’s inoculation to evaluate the survival of AMF and maintenance of the effects taking the cost-effectiveness into consideration.
2. Materials and methods
2.1. Experimental design
We grew three soybean (Glycine max (L.) Merrill) cultivars, Enrei, Misuzudaizu, and Akishirome, in an experimental field managed by the long-term selective application of major nutrients (NPK and -P) from 1981 with bare fallow (no plants of any kind from 1981) at the Central Region Agricultural Research Center, NARO, Tsukuba, Japan (36°01ʹN, 140°06ʹE). The soil properties (0–10°cm in depth) of the field at the start of the experiment are shown in . The soil is classified as a Haplic allophanic Andosol according to Obara et al. (Citation2011) and as an Umbric Silandic Andosol according to IUSS Working Group (Citation2014). Annual mean temperature and annual precipitation of the experimental field were 15.2°C and 1256.0 mm in 2015, respectively, and 14.8°C and 1567.0 mm in 2016, respectively. The NPK plots received 70 kg N ha−1, 140 kg P2O5 ha−1, and 100 kg K2O ha−1 every autumn and another 30 kg N ha−1 every spring. The -P plots received the same except for P. The plots were rotary tilled before sowing. Enrei seeds were sown on 8 June in 2015, and all three cultivars seeds were sown on 21 June in 2016 in the experimental field. We sprayed herbicides in the experimental field after sowing, and weeding and agrochemical spraying for insect pest control were performed appropriately. The experimental fields in 2015 and 2016 were continuous. In 2015, we dug a hole (~5 cm deep) before sowing to apply 5 g of AMF material (Glomus sp. R10, Idemitsu Kosan Co., Ltd., Tokyo, Japan, hereafter, we describe this inoculum as R10.) per hill in inoculation plots or 5 g of carrier (Idemitsu Kosan Co., Ltd., Tokyo, Japan) in control plots. In 2016, we dug a ditch (5–10 cm deep) along the row and applied 110 g m−1 (approximately 20 g per hill) of inoculum or carrier in a band, expecting a bigger effect than in 2015. After filling the ditch, we sowed seeds. In each year, each hill had two plants, and the space between rows was 60 cm with a 20-cm distance between hills. In 2016, Enrei was grown in the same plots as in 2015 to determine any residual effect. Replication of each plot was two.
Table 1. Soil characteristics at the plots used in this study.
2.2. Plant sampling and measurement of AMF colonization
Eight plant samples in four hills were collected at flowering and seed maturation (except in the residual effect plots), and 32 plant samples in 16 hills in 2015 and 20 plant samples in 10 hills in 2016 were collected at harvest stages. In Enrei, flowering stage was on 21 July in 2015 and on 1 August in 2016, and seed maturation stage was on 24 August in 2015 and on 29 August in 2016, and harvest stage was on 6 October in 2015 and on 20 October in 2016. In regards to Misuzudaizu and Akishirome grown in 2016, flowering stage was on 9 August, and seed maturation stage was on 15 September, and harvest stage was on 27 October. At flowering stage, whole-plant samples were separated into shoots and roots. The roots were washed and cut into 1-cm pieces. Some were stained with 3,3′-diaminobenzidine (DAB) (Kobae and Ohtomo Citation2016), and the rest were used for DNA extraction. AMF colonization was measured according to the grid line intersect method of Giovannetti and Mosse (Citation1980). The shoots were oven-dried at 70°C and weighed. They were then ground and digested with concentrated H2SO4 and hydrogen peroxide, and P concentrations of the digests were determined colorimetrically by the vanadomolybdate method. N concentration in the ground sample was determined by Sumigraph NC220F (Sumika Chemical Analysis Service, Ltd., Osaka, Japan). At harvest, we evaluated dry matter yield, number of pods, and seed yield according to the test guidelines of the Ministry of Agriculture, Forestry and Fisheries of Japan (http://www.hinsyu.maff.go.jp/info/sinsakijun/botanical_taxon.html).
2.3. DNA extraction, PCR amplification, and sequencing
Freeze-dried root samples were ground in 3-mL tubes with a metal cone at 3000 rpm for 45 s at room temperature in a Multi-Beads Shocker (Yasui Kikai, Osaka, Japan). DNA was extracted from 10–20 mg of the ground samples with a DNeasy Plant Mini Kit (Qiagen, Tokyo, Japan) according to the manufacturer’s instructions and stored at −30°C. The large subunit ribosomal RNA gene (LSU rDNA) was amplified with the forward primer Miseq3 (5ʹ-GTG AAA TTG TTG AAA GGG AAA CG-3ʹ) which is specific to the region between divergent domains 1 (D1) and D2 in LSU rDNA and the fungus-specific reverse primer FLR2 (Trouvelot et al. Citation1999). In a preliminary experiment, we confirmed that the AMF compositions of the products amplified with these primers were similar to those of the products amplified with the conventional forward primer LR1 (Van Tuinen et al. Citation1998) and FLR2 (data not shown). PCR mixtures consisted of the Expand High-Fidelity PLUS PCR System enzyme mix (Roche Diagnostics, Tokyo, Japan), 0.5 nmol µL–1 each of the forward and reverse primers, and the DNA extract at five different volumes (0.01, 0.02, 0.1, 0.5, and 1.0 µL) in a total of 25 µL. The DNA region was amplified in a C1000 Touch Thermal Cycler (BIO-RAD, Tokyo, Japan) with the following program: initial denaturation at 94°C for 2 min; 30 cycles of denaturation at 94°C for 15 s, annealing at 48°C for 40 s, and extension at 72°C for 1 min; and a final extension at 72°C for 10 min. All PCR products obtained from the dilution series of the same DNA extract were combined, purified in an Agencourt AMPure XP PCR Purification system (Beckman Coulter, Tokyo, Japan), and amplified by 2nd PCR for indexing with a Nextera XT Index Kit v2 (Illumina K.K., Tokyo, Japan) according to the 16S Metagenomic Sequencing Library Preparation protocol (https://support.illumina.com/downloads/16s_metagenomic_sequencing_library_preparation.html). The libraries were pooled, adjusted to 4 nM DNA, denatured with NaOH, and then diluted with hybridization buffer to a final concentration of 6 pM DNA. Paired-end sequencing was carried out on an Illumina MiSeq platform using the MiSeq Reagent Kit v3 (600 cycles) (Illumina K.K., Tokyo, Japan). Nucleotides with a quality value of <30 at the 3ʹ terminus and the adapter-index sequence at the 5ʹ terminus were trimmed from the MiSeq reads in PRINSEQ v0.20.4 software (Schmieder and Edwards Citation2011). After quality filtering, the paired reads were merged to construct overlapping fragments in COPE v1.1.3 software (Liu et al. Citation2012). The merged sequence reads were used in BLASTN searches against the GenBank database, and only those that showed the highest similarity to an AMF sequence, with an E-value of −100 or less, ≥95% nucleotide identity, and alignment length of ≥330 bp, were assigned as AMF. The fungal sequences were clustered at 95% similarity in CD-HIT software (Fu et al. Citation2012), and representative sequences were defined as AMF operational taxonomic units (OTUs).
2.4. DNA tracking markers for inoculum R10
To identify DNA tracking markers for R10 before field trials, we grew Allium fistulosum, cultivar, Motokura in sterilized soil inoculated with R10 at 50 g kg−1 in nursery pots for 42 days in a greenhouse and extracted the DNA from the roots. The D2 region in LSU rDNA was amplified, sequenced, and used in BLASTN searches. Seven R10 OTUs corresponding to Rhizophagus irregularis OTUs were used as tracking markers.
2.5. Statistical analysis
Statistical analysis was performed in SPSS v. 17.0 software (SPSS Inc., Chicago, IL, USA). Means of shoot dry weight at flowering or seed maturation stage, N and P uptake at flowering stage, dry matter yield, number of pods, and seed yield in each combination of cultivar and nutrient treatment were analyzed by t-test with AMF treatment as a factor.
3. Results
3.1. AMF colonization in soybean roots
AMF colonization rates tended to be higher in the inoculation plots than in the non-inoculation (control) plots at flowering stage (). In the inoculation plots, the colonization rate was higher in 2016 (when approximately 20 g of inoculum was applied per hill) than in 2015 (when 5 g was applied per hill). In both years, inoculation increased the relative read abundance of R10-type OTUs (), indicating that introduced R10 colonized in the roots would be well. In roots of Akishirome in the -P and control plots, the relative read abundance of R10-type OTUs increased for no obvious reason. In the residual-effect plots, the colonization rate increased in plots both with and without inoculation in 2016, but it was higher in the inoculation plots than in the control plots (). There were no particular differences in AMF colonization between the -P and the NPK plots.
Figure 1. AMF colonization of soybean roots with or without R10 inoculum at flowering stage. A line graph shows means of the colonization rates. Bars indicate standard deviation of the means. Stacked column charts show means of relative read abundance of R10-type and other indigenous AMF OTUs. En_15, Enrei examined in 2015; En_res., residual effect of AMF inoculum in 2015 on Enrei examined in 2016; En, Enrei examined in 2016; Mi, Misuzudaizu examined in 2016; Ak, Akishirome examined in 2016.
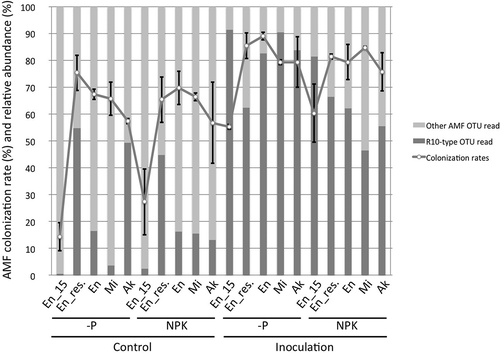
3.2. Effects of AMF inoculation at flowering and seed maturation stages
In the -P plots, inoculation tended to increase the shoot dry weight of all three cultivars at flowering and seed maturation stages (, ) and significantly increased that of Misuzudaizu at flowering stage. The previous year’s inoculation showed a slight residual effect in Enrei, but it was not significantly different. In the NPK plots, however, inoculation did not significantly increase shoot dry weight at either stage. In addition, it tended to increase N and P uptake in the -P plots but not in the NPK plots except for P uptake of Akishirome (, ).
Figure 2. R10 inoculum effect on soybeans at flowering and seed maturation stages. Means of shoot dry weight at flowering stage (A) and seed maturation stage (B). Means of N uptake (C) and P uptake (D) at flowering stage. Bars indicate standard deviation of the means. Significant differences between inoculation and control treatments identified by t-test were indicated with asterisk(s) (*P < 0.05, **P < 0.01). Shoot dry weight in the plots of the residual effect on Enrei (En_res.) at seed maturation stage (B) was not determined. En_15, Enrei examined in 2015; En_res., residual effect of AMF inoculum in 2015 on Enrei examined in 2016; En, Enrei examined in 2016; Mi, Misuzudaizu examined in 2016; Ak, Akishirome examined in 2016.
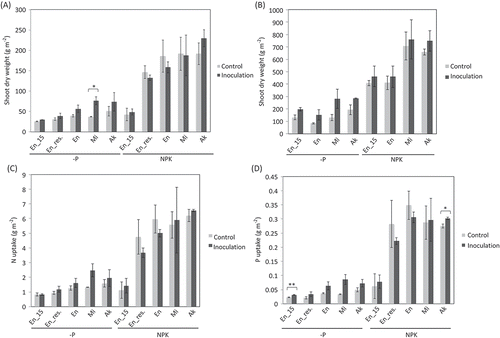
3.3. Effects of AMF inoculation at harvest
In the -P plots, inoculation significantly increased the dry matter yield of Misuzudaizu and Akishirome and significantly increased the number of pods of Enrei and Akishirome (, ). Inoculation of Enrei tended to be more effective in 2016 than in 2015, and there was a tendency of residual effect on the number of pods, especially. Although we could not accurately evaluate the effect on seed yield on account of severe insect damage in 2016, inoculation tended to increase it in the -P plots (). In the NPK plots, in contrast, inoculation did not significantly increase any yields.
Figure 3. R10 inoculum effect on soybeans at harvest. Means of dry matter weight (A), number of pods per 10 plants (B), and seed yield (C). Seed yield were reduced by severe insect damage in particular 2016. Bars indicate standard deviation of the means. Significant differences between inoculation and control treatments identified by t-test were indicated with asterisk (*P < 0.05). En_15, Enrei examined in 2015; En_res., residual effect of AMF inoculum in 2015 on Enrei examined in 2016; En, Enrei examined in 2016; Mi, Misuzudaizu examined in 2016; Ak, Akishirome examined in 2016.
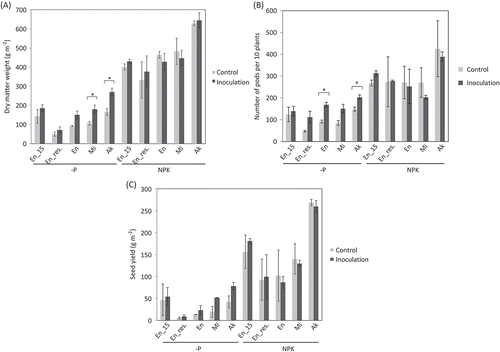
4. Discussion
The success of the inoculation depends on many factors, including the competition with indigenous AMF (Verbruggen et al. Citation2013) and the accumulation of available P in soil (Mosse et al. Citation1973). Douds Jr. et al. (Citation2011) demonstrated that enforced bare fallow strongly diminished AMF propagule density with the passage of time and that inoculation was effective for plant growth in the AMF-depleted soil by the enforced bare fallow though inoculation was noneffective in cropped soil. As the field in our study was maintained under the long-term bare fallow, and therefore there were no host plants to support AMF for a long time, we thought that there would be few indigenous AMF to compete with the introduced R10. The AMF colonization and the relative read abundance of R10-type OTUs were similarly increased by AMF inoculum both in the -P and NPK plots (), which might indicate that inoculated R10 successfully colonized to soybean roots irrespective of fertilizer treatments in this study. However, the effect of AMF inoculum on soybean growth was only observed in -P plots, and the effect was not prominent in NPK plots.
Available P in soil is known to influence the effect of AMF inoculum on plant growth (Mosse et al. Citation1973). Andosols tend to have very low concentration of available P and the high phosphate absorption coefficient of the soil, and soil available P in the -P plots was extremely low and that in the NPK plots was still low in this study (). P is suggested to be the most influential element in AMF colonization, which decreases with increasing P concentration in soil (Smith and Read Citation2008); however, reduction in AMF colonization and relative read abundance of R10-type OTUs were not observed in the NPK plots in comparison with the -P plots (). In this study, inoculum effect was not clear in the NPK plots. Since it was suggested that Truog-P tends to underestimate available P in allophanic Andosols (Ito et al. Citation2011), and since growth of soybean in the NPK plots was much greater than that in the -P plots, available P in the NPK plots might be enough for soybean to take up through its roots directly.
In this study, the AMF inoculum tended to increase not only shoot dry weight, N uptake, and P uptake (, , ), but also P, Zn, and Cu concentration (mg g−1 dry weight of shoots) in Misuzudaizu at flowering stage (data not shown). This may indicate that growth promotion observed in Misuzudaizu was brought by increase in nutrient uptake through inoculated AMF, since AMF has been suggested to increase plant P, Zn, and Cu uptake (Marschner and Dell Citation1994).
AMF colonization rates in 2016 tended to be higher than those in 2015 in the control plots. This might be due to contamination of both native and introduced AMF propagated in the experimental fields in 2015 and native AMF propagated in an adjacent cropped field by several rotary tilling and/or different environmental conditions, e.g., weather in 2015 and 2016. The relative read abundance of R10-type OTUs increased in the control residual-effect plots (). We thought that it might be caused by contamination for the reasons mentioned above. Nevertheless, the colonization rate was higher and the relative read abundance of R10-type OTUs was greater in the inoculated plots, indicating that inoculation in 2015 contributed to the colonization of soybean roots in 2016.
In our preliminary pot experiment, we confirmed that the colonization rate rose with increase in inoculum dose up to 20 g per pot (data not shown). Consequently, we increased the inoculum dose from 5 g to approximately 20 g per hill and then extended the inoculation area from a planting hole to a ditch in 2016. We considered that by adopting this inoculation strategy, in the -P plots, the colonization rate of Enrei increased in 2016 than that of in 2015 (), and accordingly, the inoculation for Enrei in 2016 was more effective than that in 2015 (, and , ).
In addition to P availability and AMF competitors, an important factor in the success of AMF inoculation is the genotype of the host plant (Rengel Citation2002). By comparing the mycorrhizal dependency between modern Triticum aestivum cultivars and their ancestors, Hetric et al. (Citation1993) proposed that modern breeding reduced the responsiveness to AMF. On the other hand, improved oat cultivars were more responsive to AMF than wild oats (Koide et al. Citation1988). Our evaluation of three soybean cultivars suggested that each cultivar showed inoculum effects under the above field conditions.
In this study, it was suggested that the inoculation of AMF was effective on soybean, and the tendency of its effect remained until the harvest () under the field conditions in which soil P level and competitor AMF population were not high at least. The soybean plants in the -P plots showed P deficiency symptom, and their growth was inferior to those in the NPK plots. We considered that the growth would be improved by the application of less P fertilizer in combination with the AMF inoculum. Our results might help in the development of strategies to reduce the need for P fertilizer, to use P fertilizer more efficiently, and to reclaim abandoned or degraded land.
Acknowledgments
We thank the Kyushu Okinawa Agricultural Research Center, NARO, Japan, the Hiroshima Prefectural Technology Research Institute’s Agricultural Technology Research Center, Japan, and the Nagano Vegetable and Ornamental Crops Experiment Station, Japan, for providing soybean seeds; Dr. Takumi Sato and Prof. Keitaro Tawaraya (Yamagata University) for providing Welsh onion seedlings; Ms. Miho Ishitsuka, Ms. Noriko Iioka, and Ms. Noriko Tayama (Central Region Agricultural Research Center, NARO, Japan) for their technical assistance; and Mr. Katsumi Sakamoto, Mr. Kiyoshi Yokotsuka, and Mr. Ichiro Ameta (Headquarter, NARO, Japan) for their management of the field.
Additional information
Funding
References
- Azaizeh HA, Marschner H, Römheld V, Wittenmayer L 1995: Effects of a vesicular-arbuscular mycorrhizal fungus and other soil microorganisms on growth, mineral nutrient acquisition and root exudation of soil-grown maize plants. Mycorrhiza, 5, 321–327. doi:10.1007/BF00207404
- Chalk PM, Souza RDF, Urquiaga S, Alves BJR, Boddey RM 2006: The role of arbuscular mycorrhiza in legume symbiotic performance. Soil Biol. And Biochem., 38, 2944–2951. doi:10.1016/j.soilbio.2006.05.005
- Clark RB, Zeto SK 2000: Mineral acquisition by arbuscular mycorrhizal plants. J. Plant Nutr., 23, 867–902. doi:10.1080/01904160009382068
- Daft MJ, Hacskaylo E 1977: Growth of endomycorrhizal and nonmycorrhizal red maple seedlings in sand and anthracite spoil. Forest Sci., 23, 207–216.
- Douds DD Jr, Nagahashi G, Wilson DO, Moyer J 2011: Monitoring the decline in AM fungus populations and efficiency during a long term bare fallow. Plant Soil, 342, 319–326. doi:10.1007/s11104-010-0697-3
- Fernández MC, Boem FHG, Gerardo Rubio G 2011: Effect of indigenous mycorrhizal colonization on phosphorus-acquisition efficiency in soybean and sunflower. J. Plant Nutr. Soil Sci., 174, 673–677. doi:10.1002/jpln.201000109
- Fu LM, Niu BF, Zhu ZW, Wu ST, Li WZ 2012: CD-HIT: accelerated for clustering the next-generation sequencing data. Bioinformatics, 28, 3150–3152. doi:10.1093/bioinformatics/bts565
- Giovannetti M, Mosse B 1980: An evaluation of techniques for measuring vesicular-arbuscular mycorrhizal infection in roots. New Phytol., 84, 489–500. doi:10.1111/j.1469-8137.1980.tb04556.x
- Hetric BAD, Wilson GWT, Cox TS 1993: Mycorrhizal dependence of modern wheat cultivars and ancestors: a synthesis. Can. J. Bot., 71, 512–518. doi:10.1139/b93-056
- Ito T, Kikawa N, Saigusa M 2011: Phosphorus sorption and bioavailability in allophanic and: non-allophanic Andosols. Pedologist., 55, 84–88. (in Japanese).
- IUSS Working Group 2014: World reference base for soil resources 2014: international soil classification system for naming soils and creating legends for soil maps. In World Soil Resources Reports, Vol. 106, pp. 1–116. FAO, Rome.
- Khalil S, Loynachan TE, Tabatabai MA 1994: Mycorrhizal dependency and nutrient-uptake by improved and unimproved corn and soybean cultivars. Agron. J., 86, 949–958. doi:10.2134/agronj1994.00021962008600060005x
- Khalil S, Loynachan TE, Tabatabai MA 1999: Plant determinants of mycorrhizal dependency in soybean. Agron. J., 91, 135–141. doi:10.2134/agronj1999.00021962009100010021x
- Klironomos JN 2003: Variation in plant response to native and exotic arbuscular mycorrhizal fungi. Ecology., 84, 2292–2301. doi:10.1890/02-0413
- Kobae Y, Ohotomo R 2016: An improved method for bright-field imaging of arbuscular mycorrhizal fungi in plant roots. Soil Sci. Plant Nutr., 62, 27–30. doi:10.1080/00380768.2015.1106923
- Koide R, Li M, Lewis J, Irby C 1988: Role of mycorrhizal infection in the growth and reproduction of wild vs. cultivated plants. I. Wild vs. cultivated oats. Oecologia. 77, 537–543. doi:10.1007/BF00377271
- Liu BH, Yuan JY, Yiu SM et al. 2012: COPE: an accurate k-mer-based pair-end reads connection tool to facilitate genome assembly. Bioinformatics, 28, 2870–2874. doi:10.1093/bioinformatics/bts563
- Marschner H, Dell B 1994: Nutrient uptake in mycorrhizal symbiosis. Plant Soil, 159, 89–102. doi:10.1007/BF00000098
- Mårtensson AM, Carlgren K 1994: Impact of phosphorus fertilization on VAM diaspores in two Swedish long-term field experiment. Agric. Ecosyst. Environ., 47, 327–334. doi:10.1016/0167-8809(94)90099-X
- Mosse B, Hayman DS, Arnold DJ 1973: Plant growth response to vesicular-arbuscular mycorrhiza. V. Phosphate uptake by three plant species from P-deficient soils labelled with 32P. New Phytol., 72, 809–815. doi:10.1111/j.1469-8137.1973.tb02056.x
- Obara H, Ohkura T, Takata Y, Kohyama K, Maejima Y, Hamazaki T 2011: Comprehensive soil classification system of Japan first approximation. Bulletin of National Institute for Agro-Environmental Sciences, 29, 1–73 (in Japanese with English summary).
- Ortas I 2012: The effect of mycorrhizal fungal inoculation on plant yield, nutrient uptake and inoculation effectiveness under long-term field conditions. Field Crop Res., 125, 35–48. doi:10.1016/j.fcr.2011.08.005
- Pellegrino E, Bedini S, Avio L, Bonari E, Giovannetti M 2011: Field inoculation effectiveness of native and exotic arbuscular mycorrhizal fungi in Mediterranean agricultural soil. Soil Biol. Biochem., 43, 367–376. doi:10.1007/s00572-014-0600-9
- Rengel Z 2002: Breeding for better symbiosis. In Food Security in Nutrient-Stressed Environments: Exploiting Plants’ Genetic Capabilities, Ed. Adu-Gyamfi JJ, pp. 245–260. Kluwer Academic Publishers, Dordrecht. doi:10.1007/978-94-017-1570-6_27
- Schmieder R, Edwards R 2011: Quality control and preprocessing of metagenomic datasets. Bioinformatics, 27, 863–864. doi:10.1093/bioinformatics/btr026
- Smith SE, Read DJ 2008: Mycorrhizas in agriculture, horticulture and forestry. In Mycorrhizal Symbiosis, Eds. Smith SE, Read DJ, 3rd edition. pp. 611–636. Academic Press, London.
- Tawaraya K 2003: Arbuscular mycorrhizal dependency of different plant species and cultivars. Soil Sci. Plant Nutr., 49, 655–668. doi:10.1080/00380768.2003.10410323
- Thingstrup I, Rubaek G, Sibbensen E, Jakobsen I 1998: Flax (Linum usitatissimum L.) depends on arbuscular mycorrhizal fungi for growth and P uptake at intermediate but not high soil P levels in the field. Plant Soil, 203, 37–46. doi:10.1023/A:1004362310788
- Trouvelot S, Van Tuinen D, Hijri M, Gianinazzi-Pearson V 1999: Visualization of ribosomal DNA loci in spore interphasic nuclei of glomalean fungi by fluorescence in situ hybridization. Mycorrhiza, 8, 203–206. doi:10.1007/s005720050235
- Van Tuinen D, Jacquot E, Zhao B, Gollotte A, Gianinazzi-Pearson V 1998: Characterization of root colonization profiles by a microcosm community of arbuscular mycorrhizal fungi using 25S rDNA-targeted nested PCR. Mol. Ecol., 7, 879–887. doi:10.1046/j.1365-294x.1998.00410.x
- Verbruggen E, van der Heijden MGA, Rillig MC, Kiers ET 2013: Mycorrhizal fungal establishment in agricultural soils: factors determining inoculation success. New Phytol., 197, 1104–1109. doi:10.1111/j.1469-8137.2012.04348.x