ABSTRACT
Winter cover crops increase the amount of indigenous arbuscular mycorrhizal fungi (AMF) in the soil, providing beneficial effects such as enhancement of phosphorus uptake by the subsequent crop. However, its impact on the AMF community structure is not well understood. In the present study, we aimed to reveal the effect of winter wheat cover cropping with no-till cultivation on the AMF community structures in soil and roots of the subsequent soybean. For this purpose, we conducted a field experiment consisting of two treatments, no-till soybean cultivation after winter wheat cover cropping (NTWC) and conventional soybean cultivation after winter fallow management as a control (CONT). At the flowering stage of soybean, higher AMF colonization of soybean roots was observed in the NTWC plots compared with the CONT plots. Additionally, aboveground biomass and phosphorus uptake of soybean in the NTWC plots were significantly higher than those in the CONT plots. Molecular community analyses based on PCR-denaturing gradient gel electrophoresis (DGGE) of AMF 18S rRNA genes indicated that the AMF community structures in the soil and soybean root of the NTWC plots were clearly different from those of the CONT plots. The DGGE profiles showed that the wheat cover cropping preferentially increased some phylotypes belonging to Glomeraceae and Claroideoglomeraceae. In addition, most of the phylotypes were characteristically observed in the subsequent soybean root of the NTWC plots, strongly suggesting that these phylotypes colonizing the cover crop wheat were taken over by the subsequent soybean. Our study revealed the significant effect of winter cover cropping with no-till cultivation on the structure of AMF community colonizing the subsequent soybean.
KEY WORDS:
1. Introduction
Most agricultural crops exhibit symbiotic relationships with arbuscular mycorrhizal fungi (AMF). AMF facilitate the uptake of nutrients particularly phosphorus from the soil by the host plant roots (Smith and Read Citation2008) and increase the plants’ tolerance to various environmental stresses such as drought (Augé Citation2001) and plant pathogens (Azcón-Aguilar and Barea Citation1996). Utilizing these beneficial functions of AMF is one of the promising options to develop resource-saving and sustainable agricultural system.
Two main strategies are employed to utilize AMF in the field. The first strategy is to increase AMF colonization of host plants by inoculating the soil with exotic AMF strain(s). There have been many reports on the inoculation effect of AMF (Berruti et al. Citation2016), and a number of AMF inoculants are commercially available (Tarbell and Koske Citation2007). However, because AMF are obligate symbionts, large-scale production systems for host plants are generally required to produce large amounts of AMF inoculants, making them expensive (Berruti et al. Citation2014). In addition, establishment of exotic AMF in inoculated soil is strongly affected by their compatibility with the surrounding environment (Verbruggen et al. Citation2012). Thus, the use of exotic AMF strains is not always a practical strategy. The second strategy is to enhance the function of indigenous AMF via AMF-promoting agricultural practices. For example, winter cover-cropping with AMF host plants such as wheat (Triticum aestivum L.) or hairy vetch (Vicia villosa) has been demonstrated to increase the AMF inoculum in the soil and promote AMF colonization of the subsequent crop (Galvez et al. Citation1995; Boswell et al. Citation1998). Similarly, intercropping and use of living mulch systems are also known to promote AMF colonization of cash crops (Deguchi et al. Citation2007; Karasawa and Takebe Citation2012). Although this strategy is an indirect approach compared with direct inoculation of exotic AMF strains, it can avoid the problem in adaptability of the inoculated strains to the environment. More importantly, the strategy has an advantage in facility to apply larger field with lower cost than the inoculation approach. Thus, the latter strategy can be thought as a more practical choice especially for land-intensive crops such as soybean.
Winter cover cropping has been known to provide various agricultural benefits such as preventing soil erosion, weed management, and improvement soil fertility, besides increasing AMF colonization of the subsequent crops (Clark Citation2007). To maximize these benefits, the subsequent crops after winter cover crops are often cultivated without tillage. On the other hand, conventional cultivation of field crops in Japan usually involves tillage practices before sowing. Tillage has been known to have negative impacts on indigenous AMF because it destructs their external hyphal network (Boswell et al. Citation1998; Kabir et al. Citation1999; Usuki et al. Citation2007). Thus, no-till cultivation can be thought as an important factor for maintaining indigenous AMF functions enhanced by mycorrhizal winter cover cropping.
Many studies have shown that mycorrhizal winter cover cropping promoted AMF colonization of the subsequent crop roots, thereby improving its phosphorus uptake and growth (Boswell et al. Citation1998; Kabir and Koide Citation2002; Shimazaki et al. Citation2008; Uchida et al. Citation2011; Isobe et al. Citation2014; Njeru et al. Citation2014; Higo et al. Citation2018). This effect has been investigated mainly by quantifying AMF inoculum potential in soil or AMF colonization rate of roots, and in most cases, composition of AMF communities has not been assessed. On the other hand, it has been reported that the effect of AMF on host plants varies with the AMF species (Smith et al. Citation2004; Jansa et al. Citation2008). Therefore, when considering the AMF-promoting effects of winter cover cropping, it is important to understand the impact of the winter cover cropping not only on AMF abundance, but also on AMF community composition. Higo et al. (Citation2013) reported that the AMF community composition in the soil after winter wheat cover cropping was clearly different from that in the soil after winter fallow management. On the other hand, in another report based on a 5-year field experiment, they indicated that different winter cover crops or winter fallow managements did not affect significantly the AMF community structure in the roots of subsequent soybean (Higo et al. Citation2014). Since few studies have investigated the AMF communities in soil and roots of winter cover crops and the subsequent crop in parallel, further investigations are still needed to better understand the impact of this agricultural practice on indigenous AMF community structure.
Here, we aimed to reveal the effect of winter wheat cover cropping with no-till cultivation on the AMF community colonizing the subsequent soybean root and soil. We conducted a field experiment to test the hypotheses that the community structure of AMF colonizing the no-till soybean cultivated after winter wheat cover cropping would be different from that of the soybean cultivated with conventional tillage after winter fallow management. For this purpose, the AMF community structures in the root and soil samples collected from the field were analyzed by PCR-denaturing gradient gel electrophoresis (DGGE) of partial AMF 18S rRNA genes.
2. Materials and methods
2.1. Field management and experimental design
Experiments were conducted at the Tohoku Agricultural Research Center, Fukushima, Japan (37°42′43′′ N and 140°23′15′′ E). The soil type of the field was Haplic Andosols and the soil texture was clay loam (sand, 43.6%; silt, 27.0%; and clay, 29.4%). The preceding crop in the field was wild oat (Avena fatua cv. Hay-Oats), which was plowed down as green manure on 15 August 2011. Physicochemical properties of the soil at the beginning of the field experiment (17 October 2011) were as follows: pH 6.2; total C, 44.1 g kg−1; total N, 3.2 g kg−1; available soil P2O5, 142 mg kg−1; exchangeable K2O, 590 mg kg−1; exchangeable CaO, 4313 mg kg−1; exchangeable MgO, 782 mg kg−1; CEC, and 35.3 cmolc kg−1.
The field experiment consisted of two treatments, no-till soybean cultivation after winter wheat cover cropping (NTWC) and conventional soybean cultivation after winter fallow management as a control (CONT), arranged in a randomized block design with three replications. The dimensions of each plot were 9 m × 4.2 m. In the NTWC plots, seeds of the winter wheat cultivar Yukichikara were sown at the rate of 10 g m−1 on 17 October 2011. The row-to-row spacing was alternated between 30 and 40 cm. Meanwhile, the CONT plots were kept fallow until the next spring. On 12 June 2012, the winter wheat in the NTWC plots was cut down by a hammer-knife mower to cover soil surface with the wheat residue, then soybean was sown without tillage between the wheat rows spaced 40 cm apart, resulting in 70-cm row-to-row spacing for soybean. The resultant planting density of soybean was 14.3 plants m−2. In the CONT plots, soybean was sown after conventional tillage using a rotary tiller, at the same planting density as in the NTWC plots. The NTWC and CONT plots were fertilized at sowing with 2, 8, and 8 g m−1 of N, P2O5, and K2O, respectively. Soybean was harvested from all plots on 7 November 2012.
2.2. Sampling of soil and plants
During the experimental period, soil was sampled a total of three times: on 17 October 2011 (at the sowing of winter wheat), on 12 June 2012 (at the sowing of soybean), and on 31 July 2012 (at the flowering of soybean). At each sampling time point, five soil samples (0–15 cm depth) were collected using an auger from different locations in a plot, and later combined to form one sample representing each plot. The soil samples were then passed through a 2-mm sieve and stored at −30°C until further analysis.
In the NTWC plots, after cutting down the winter wheat on 12 June 2012, wheat roots along a 20-cm row were sampled at three points per plot and pooled. The root samples were washed with tap water to remove the attached soil and stored at −30°C until further analysis.
On 31 July 2012, four soybean plants including roots per plot were sampled from different points per plot and pooled. The samples were divided into aboveground and underground parts. The aboveground tissue was for the measurement of biomass and phosphorus uptake, whereas the underground tissue was washed with tap water to remove excess soil and used for the measurement of AMF colonization and DNA extraction.
Soybeans were harvested on 2 November 2012. The harvested soybeans were used to calculate soybean yield and 100-seed weight. Twenty soybean plants randomly selected per plot were used to measure shoot length, branch number, and pod number.
2.3. Analysis of phosphorus concentration of soybean plants
Aboveground soybean tissues sampled at the flowering stage were dried at 70°C for 3 days and ground in a Waring blender. A 0.5-g sample of ground tissue was digested in nitric acid at 100°C for 1 h. Phosphorus in the digested samples was analyzed using the Vista-MPX Inductively Coupled Plasma Optical Emission Spectrometer (ICP-OES, Varian Inc., Palo Alto, CA, USA).
2.4. Measurement of AMF colonization on soybean root
The AMF colonization of soybean roots was measured according to the magnified intersections method using trypan blue staining (Oba et al. Citation2006). Briefly, soybean roots were cut into <1 cm fragments, dispersed in 10% KOH, and boiled at 140°C for 15 min on a hot plate. The root samples were then washed with tap water and acidified with 2% HCl. The acidified samples were stained with 0.05% trypan blue in lacto-glycerol. The rate of AMF colonization was determined on the basis of microscopic observations of more than 150 intersections per sample.
2.5. DNA extraction from soil and plant roots
DNA extraction from the soil was performed using FastDNA SPIN Kit for Soil (QBiogene, Irvine, CA, USA) with slight modifications. Briefly, 0.4 g of the soil sample was suspended in the extraction buffer supplied with the kit. To prevent DNA adsorption on Andosols, 80 µL of 20% (w/v) skim milk solution was added to the mixture (Hoshino and Matsumoto Citation2004). Subsequently, the mixture was disrupted using a FastPrep FP100A (QBiogene) for 30 s at a speed setting of 5.5. Extracted DNA was further purified using DNA Clean & Concentrator-25 Kit (Zymo Research, Orange, CA, USA) to thoroughly remove humic contaminants and eluted in an 80-µL volume.
DNA from soybean and wheat roots was extracted using FastDNA Kit (QBiogene) according to the manufacturer’s instructions. Root samples were cut into approximately 1-mm pieces. Approximately 0.2 g (fresh weight) of each sample was disrupted using the FastPrep FP100A for 45 s at a speed of 6.0. Extracted DNA was further purified and concentrated using the DNA Clean & Concentrator-25 kit (Zymo Research), and eluted in a 50-µL volume.
2.6. PCR amplification of partial 18S rRNA genes of AMF
For community analysis of AMF by PCR-DGGE, specific primers for AMF 18S rRNA genes, GC-AMV4.5NF (5′-CGCCCGCCGCGCGCGGCGGGCGGGGCGGGGGCACGGGGGGAAGCTCGTAGTTGAATTTCG-3′, underlined nucleotides indicate a 40-bp GC clamp) (Sato et al. Citation2005) and AMVR (5′-CTGACAATTGAATACTAATGCC-3′) were used. The reverse primer (AMVR) was designed in this study because our preliminary experiments showed that the original reverse primer, AMDGR (Sato et al. Citation2005), amplified genes from some non-AMF species and failed to obtain AMF profiles of some samples obtained in this study (data not shown). The annealing site of AMVR is adjacent to that of AMDGR (Fig. S1). The specificity of new primer combination for AMF 18S rRNA genes was verified using NCBI Primer-Blast (http://www.ncbi.nlm.nih.gov/tools/primer-blast/) and was determined to be superior to that of the original primer combination.
All PCRs were performed in a GeneAmp 9700 system (Applied Biosystems, Foster City, CA, USA) with KOD-Plus- DNA polymerase (TOYOBO, Osaka, Japan). PCRs were carried out in a 50-µL volume comprising 5 µL of 10× PCR buffer for KOD-Plus-, 0.2 mM of each dNTP, 1.0 mM of MgSO4, 1 µL of bovine serum albumin solution (20 mg mL−1; Takara Bio, Otsu, Japan), 0.4 µM of each primer (GC-AMV4.5NF and AMVR), 1U of KOD-Plus- DNA polymerase, and 2 µL of DNA template (ca. 100–200 ng of DNA). The PCR conditions were as follows: initial denaturation at 94°C for 2 min followed by 45 cycles of denaturation at 94°C for 15 s, annealing at 56°C for 30 s, and extension at 68°C for 30 s. Amplified PCR products (ca. 320 bp) were purified using QIAquick PCR Purification Kit (Qiagen, Hilden, Germany) and quantified using a NanoDrop ND-1000 spectrophotometer (ThermoFisher Scientific, Waltham, MA, USA).
2.7. DGGE analysis
A DCode universal mutation detection system (Bio-Rad Laboratories, Hercules, CA, USA) was used for DGGE analysis of the PCR products. Approximately 150 ng of each PCR product and DGGE marker IV (Nippon Gene, Tokyo, Japan) were loaded on an 8% polyacrylamide gel with 25–40% denaturing gradient and electrophoresed at 50 V for 16 h at 60°C. The gel was stained with SYBR® Green I Nucleic Acid Gel Stain (Takara Bio) and digital images were captured using a GelDoc XR system (Bio-Rad Laboratories) with Quantity One software (Bio-Rad Laboratories). The digitized banding pattern was subjected to statistical analysis (described below). In addition, major DGGE bands were excised on a blue LED transilluminator using a disposable scalpel for sequencing analysis. A small piece of the excised band was rinsed with sterilized distilled water and directly used as a template for PCR with the same conditions as those for the original PCR, with the exceptions that the initial denaturation step was extended to 5 min and the cycle number was reduced to 36. Each re-amplified product was purified as described above and subjected to DGGE again to check its homogeneity and band position. Then, the products were directly sequenced from both ends using the BigDye Terminator v3.1 Cycle Sequencing Kit (Applied Biosystems) with AMV4.5NF (GC-AMV4.5NF without the GC-clamp) and AMVR primers on an ABI310 Genetic Analyzer (Applied Biosystems). Phylogenetic analysis of the DNA sequences was carried out using MEGA6 (Tamura et al. Citation2013).
2.8. Statistical analysis
Statistically significant differences between the NTWC and CONT treatments were evaluated with the two-tailed Welch’s t-test using the JMP12 software (SAS Institute Japan Ltd., Tokyo, Japan). The Shannon diversity index (Hʹ) was calculated from the digitized DGGE profiles of the AMF 18S rRNA genes according to the equation: Hʹ = − Σ Pi ln(Pi), where Pi is the importance probability of the DGGE bands in each lane and is calculated as the relative intensity of band. The PC-ORD6 software (MjM Software Design, Gleneden Beach, USA) was used for the following multivariate analyses based on the Bray–Curtis dissimilarity distance matrix calculated from presence/absence of each DGGE band. A hierarchical cluster analysis using the group average method was performed to show the relative relationship between the AMF community structures of all samples. Non-metric multidimensional scaling (NMDS) was used to display a two-dimensional ordination of the AMF communities in soybean root and soil at the flowering stage. In addition, to evaluate the significance of the effects of treatment (NTWC and CONT) and sample type (root and soil) on the AMF community structure at the soybean flowering stage, we performed two-way permutation-based multivariate analysis of variance (two-way PerMANOVA) with 4999 permutations.
2.9. Nucleotide sequence accession numbers
Nucleotide sequences obtained in this study were deposited in the DNA Data Bank of Japan (DDBJ: http://www.ddbj.nig. ac.jp/search/top-e.html) under the accession numbers LC274501 to LC274511.
3. Results
3.1. Properties of soybean
At the flowering stage, aboveground biomass, phosphorus concentration, phosphorus uptake, and AMF root colonization of soybean grown in the NTWC plots were compared to that grown in CONT plots (). Data revealed that aboveground biomass and phosphorus concentrations of soybean grown in NTWC plots were significantly higher than those grown in the CONT plots. Consequently, phosphorus uptake of soybean in the NTWC plots was approximately 1.5 times higher than that in the CONT plots. Additionally, significantly higher AMF colonization of soybean root was observed in the NTWC plots in comparison to the CONT plots. At the harvesting stage, shoot length and yield of soybean in the NTWC plots were significantly higher than those in the CONT plots (). However, no significant differences were detected in the number of branches and pods per plant, and 100-seed weight between the CONT and NTWC treatments.
Table 1. Aboveground biomass, phosphorus (P) concentration, P uptake, and AMF colonization of soybean at the flowering stage†.
Table 2. Soybean grain yield and yield components at the harvest stage†.
3.2. AMF community analysis based on the PCR-DGGE profiles
Partial sequences of AMF 18S rRNA genes amplified from the soil and root DNA were subjected to DGGE analysis (). Shannon diversity indices (Hʹ) calculated from the DGGE profiles are shown in . Although no significant differences were detected in Hʹ until the sowing of soybean (12 June 2012), those of soil and soybean root at the flowering stage of soybean (31 July 2012) were significantly higher in the NTWC plots than in the CONT plots ().
Figure 1. DGGE profiles of AMF 18S rRNA genes amplified from the soil and plant roots. Bands indicated with arrows were sequenced, and those with the same alphabet had an identical sequence. The subscript numbers of each band are omitted in other figures. M, DGGE marker IV (Nippon Gene, Tokyo, Japan); CONT, control; NTWC, no-till cultivation after winter wheat cover cropping.
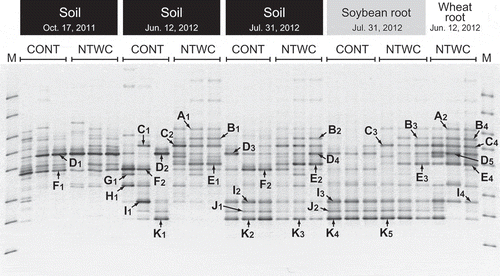
Figure 2. Shannon diversity indices based on the DGGE profiles of soil and root samples. Error bars represent standard errors of triplicate plots. Statistically significant differences were determined using Welch’s t-test and are indicated with **P < 0.01. ns, not significant; CONT, control; NTWC, no-till cultivation after winter wheat cover cropping.
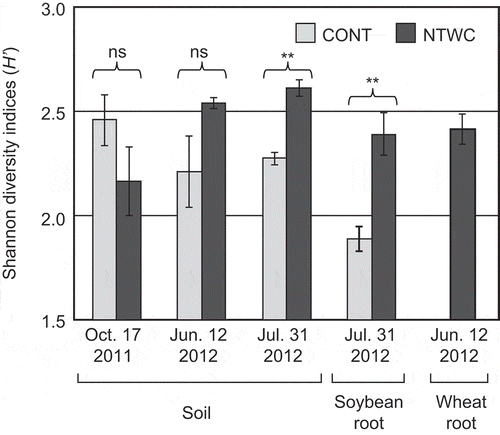
The DGGE profiles of the CONT and NTWC plots showed a different temporal change. Several bands (labeled A, B, C, and E in ) detected in the wheat root samples were characteristically observed in the NTWC soils at soybean sowing, and most of which were retained in the profiles of soil and soybean root of the NTWC plots at the flowering stage. By contrast, with a few exceptions, these bands did not appear in the soil and soybean root samples of the CONT plots; instead, other bands (labeled I, J, and K) were characteristically observed in the CONT soil and soybean root samples (). The cluster analysis showed the differences in the AMF community structures between samples more clearly (). The AMF community structures of soil and root samples (both of cover crop wheat and subsequent soybean) from the NTWC plots, except those before treatment (31 October 2011), formed a cluster characterized by the presence of several bands such as A, B, C, and E. On the other hand, those of soil and soybean root samples from the CONT plots at the flowering stage formed a different cluster.
Figure 3. Cluster analysis based on the DGGE profiles of soil and root samples. The Bray–Curtis dissimilarity coefficient calculated from band presence/absence data and the group average method were employed to construct the dendrogram. The rows and columns of heat map represent samples and DGGE bands, respectively. Names of the sequenced bands are indicated on the columns with the closest related family. CONT, control; NTWC, no-till cultivation after winter wheat cover cropping.
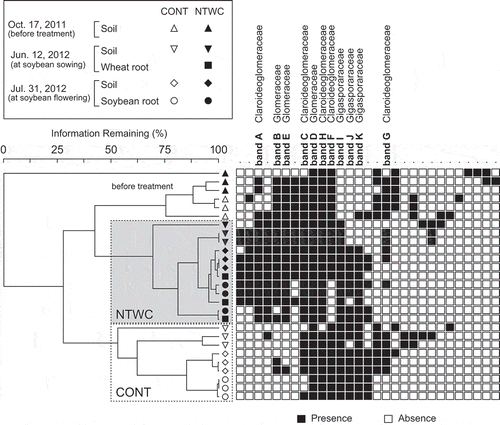
The two-dimensional ordination of the AMF community structures at the soybean flowering stage is shown in . The small stress value (2.74%) of NMDS suggested that the ordination provides a good representation of the true distances in the original multidimensional space (Kruskal Citation1964). The ordination plots were roughly divided by axes 1 and 2. The plots of the NTWC were located in quadrants I and IV, whereas those of the CONT were located in quadrants II and III. Similarly, the plots of soil samples, with one exception, were located in quadrants I and II, whereas those of soybean roots were located in quadrants III and IV. Two-way PerMANOVA revealed that both treatment (pseudo-F1,8 = 61.49, P = 0.0002) and sample type (pseudo-F1, 8 = 12.66, P = 0.003) significantly affected the AMF community structure in the soil and soybean root at the flowering stage. The interaction effect between treatment and sample type was also significant (pseudo-F1,8 = 5.98, P = 0.027).
Figure 4. Non-metric multidimensional scaling (NMDS) ordination of AMF communities in soil and soybean root samples collected at the flowering stage (31 July 2012). The NMDS was based on the Bray–Curtis dissimilarity matrix calculated from band presence/absence data of the DGGE profiles. The stress value represents the goodness of fit for the ordination in the reduced dimension (Kruskal Citation1964). CONT, control; NTWC, no-till cultivation after winter wheat cover cropping.
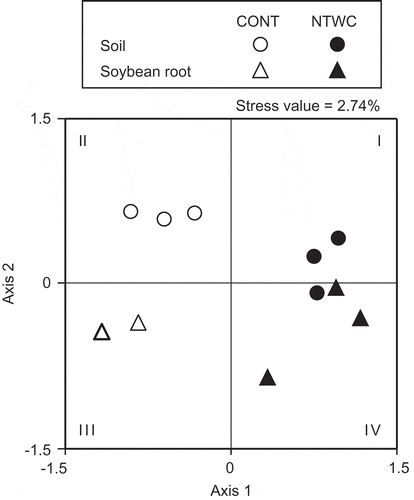
3.3. Sequencing and phylogenetic analysis of characteristic DGGE bands
The characteristic DGGE bands (A–K; ) were sequenced and subjected to phylogenetic analysis. Neighbor-joining tree was constructed using the sequences of the bands and reference sequences obtained from the DNA database (). All sequenced bands clustered with the subphylum Glomeromycotina. Among the bands characteristic of the NTWC plots, bands A and C showed high nucleotide sequence identities with Glomus sp. BSLA-7 (99.6%) and Claroideoglomus etunicatum W3808 (99.6%), respectively. Other characteristic bands of the NTWC plots, bands B and E, were closely related to each other and exhibited 99.6 and 100% identity with Glomus sp. Glo4, respectively. Bands D and F, which dominated the soil samples at the beginning of the experiment, showed 100% identity with Funneliformis mosseae BEG12 and Glomus sp. MSTA-3, respectively. Bands G and H, which were inconspicuous in all samples except a few CONT soil samples collected at soybean sowing (12 June 2012; ), were 100% identical to Glomus sp. BS06-1 and Glomus sp. ZJ, respectively. Bands I, J, and K, which composed prominent bands especially in the soil and soybean root samples of the CONT plots at the flowering stage, were closely related to each other and clustered with the family Gigasporaceae. Bands J and K showed 99.1% and 100% identities with Gigaspora margarita MAFF520054, respectively, whereas band I was 100% identical to Racocetra castaneae BEG1.
Figure 5. Neighbor-joining tree based on the partial 18S rRNA gene sequences (approximately 240 bp) obtained from the major DGGE bands and from public DNA databases. Names of the DGGE bands are indicated by letters without the subscript number. Numbers on nodes indicate the bootstrap values of 100 trials. The accession number of each sequence is indicated in parentheses. The tree is rooted with the sequence from Rhizopus oryzae (AF113440) as an outgroup.
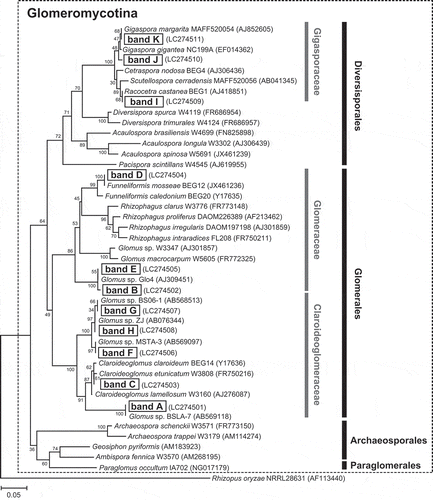
4. Discussion
Our results showed that the winter wheat cover-cropping significantly promoted AMF colonization of the subsequent no-till soybean root and improved the phosphorus uptake, growth, and yield of soybean. Though many previous studies have already reported such beneficial effects of mycorrhizal winter cover crops on the subsequent crops, most studies have not evaluated the impact of winter cover cropping on the AMF community structure (Boswell et al. Citation1998; Kabir and Koide Citation2002; Shimazaki et al. Citation2008; Uchida et al. Citation2011; Isobe et al. Citation2014; Njeru et al. Citation2014). In the present study, molecular community profiling by PCR-DGGE and multivariate analyses revealed that the AMF community structures in soil and root samples of the NTWC plots were clearly different from those of the CONT plots, supporting our hypothesis. In addition, the cluster analysis showed high similarities between the AMF community structures in the roots of winter wheat cover crop at the soybean sowing and those in the soybean roots in the NTWC plots at the flowering stage. This result strongly suggests that the AMF communities enhanced by winter wheat cover cropping were taken over by the subsequent no-till soybean. The notable feature of this study is that we demonstrated the effect of winter wheat cover cropping on the subsequent no-till soybean not only via increase in AMF colonization of the soybean roots but also via the succession of AMF community structure.
The diversity indices (Hʹ) calculated from the DGGE profiles of the NTWC plots at the flowering stage were significantly higher than those of the CONT plots. Higher diversity of AMF is thought to be beneficial for the host plants as it improves the probability of establishing mycorrhiza with various AMF partners, consequently enhancing the versatility of mycorrhizal function against changes in environmental conditions and growth stage of the host. On the other hand, some AMF species have been known to reduce host growth (Smith et al. Citation2004; Hart et al. Citation2013). We detected several characteristic bands related to Glomeraceae and Claroideoglomeraceae from the DGGE profiles of roots of the winter wheat cover crop. Previous studies also reported that phylotypes related to these families were specifically detected from roots of winter wheat or soil after winter wheat cultivation (Higo et al. Citation2011, Citation2013, Citation2016). Thus, the winter wheat cover cropping is thought to increase some phylotypes of Glomeraceae and Claroideoglomeraceae preferentially. Some of these phylotypes were characteristically detected also from the subsequent soybean roots and soil in the NTWC plots. Since the effects of different AMF phylotypes on soybean have not been revealed yet, it is uncertain whether the AMF phylotypes enhanced by the winter wheat cover cropping were actually beneficial for the soybean or not. However, given our results that the winter wheat cover cropping significantly improved growth, phosphorus uptake, and yield of the subsequent soybean along with the increase of AMF colonization rate, the community change with the winter wheat cover cropping was likely to be favorable for soybean production.
Oka et al. (Citation2010) reported that AMF colonization, phosphorus uptake, growth, and yield of soybean were increased when the preceding crop was an AMF host plant, such as wheat or sunflower (Helianthus annuus), compared with a non-host plant such as Brassica spp. Additionally, phosphorus fertilization of the soybean cultivated following AMF host plants was able to be reduced without compromising soybean yield in their study. The experimental field used in the current study had been previously cultivated with oat (Avena sativa) during summer 2011. Given the ability of oat to increase the mycorrhizal inoculum in soil (Lehman et al. Citation2012), the inoculum potential of indigenous AMF was expected to be relatively high even in the CONT plots. Indeed, the rate of AMF colonization of soybean roots in the CONT plots was over 50%, which was comparable to that of flowering-stage soybean cultivated after AMF host plants in the previous study (Oka et al. Citation2010). Nevertheless, significant increases in phosphorus uptake, growth, and yield of the soybean with the winter wheat cover cropping were observed in the present study. This suggests that the winter wheat cover cropping is useful even when AMF host plants were cultivated in the previous summer season.
Generally, tillage has been reported to reduce AMF colonization due to the physical destruction of the hyphal network (Boswell et al. Citation1998; Kabir et al. Citation1999; Usuki et al. Citation2007). In addition, since the ability of AMF to recover from damaged hyphae varies with species, tillage also affects the AMF community structure (Jansa et al. Citation2002, Citation2003; Brito et al. Citation2012). For instance, some AMF belonging to Glomeraceae and Claroideoglomeraceae have been predominantly found in intensively tilled soil because they can easily recover from physical disturbance owing to their high sporulation rate and ability to colonize via fragmented hyphae or mycorrhizal root, whereas Gigasporaceae species have been known to be highly sensitive to such disturbance (Douds et al. Citation1995; Daniell et al. Citation2001; Oehl et al. Citation2003; Verbruggen and Kiers Citation2010). However, the results of this study contradicted those of previous studies. While several phylotypes related to Glomeraceae and Claroideoglomeraceae were specifically detected from the no-tilled NTWC plots, phylotypes related to Gigasporaceae were prominently detected from the tilled CONT plots. Thus, it is possible that the negative impact of tillage on Gigasporaceae was not so severe in the CONT plots, and the winter wheat cover cropping might have a greater impact in preferentially enhancing some phylotypes of Glomeraceae and Claroideoglomeraceae in the NTWC plots. In addition, the prominence of Gigasporaceae phylotypes in the CONT plots may reflect the high affinity between this family and soybean because previous studies have also detected Gigasporaceae as a dominant AMF associated with soybean (Saito and Ronald Citation1991; An et al. Citation1993; Higo et al. Citation2014).
As described above, although our study showed high similarities between the AMF community structures in the roots of winter wheat cover crop and the subsequent soybean, Turrini et al. (Citation2016) have reported contrasting results. They investigated AMF communities in different winter cover crops (hairy vetch, berseem clover, crimson clover, lacy phacelia, and Avena sp.) and subsequent maize roots, and revealed that the composition of AMF communities was clearly different between the preceding cover crops and the subsequent maize. Their results indicate the significance of host preference as a driver of AMF community dynamics and suggest that AMF communities associated with preceding cover crops are not necessarily taken over by the subsequent crop. One possible reason for this discrepancy is that the host preferences of wheat and soybean were compatible at least under the condition of our study. Such compatibility could affect the effectiveness of winter cover crop, and our results suggest the suitability of wheat as a winter cover crop for promoting AMF colonization of soybean roots. Another possible reason is the difference in tillage management before sowing the subsequent crop. We sowed the soybean without tillage after winter wheat cover cropping, whereas the soil was physically disturbed by disc harrow before sowing the subsequent maize in the study by Turrini et al. (Citation2016). Thus, in our study, the AMF community colonizing the winter wheat roots could be taken over by the subsequent soybean more easily.
In the present study, we revealed that the winter cover cropping with no-till cultivation significantly affected the composition of AMF community colonizing the subsequent soybean root and soil. This result is consistent with a previous study by Higo et al., who indicated that the AMF community composition in the soil after winter wheat cover cropping was clearly different from that after winter fallow (Higo et al. Citation2013). However, in contrast to these results, a 5-year field experiment in their another report demonstrated that different winter cover crops including winter wheat and winter fallow managements did not have a significant impact on the AMF community structure in the subsequent soybean roots (Higo et al. Citation2014). They showed that environmental factors, such as precipitation and temperature, have a greater influence on the AMF communities than the winter cover cropping. Considering their results, the effect of winter wheat cover cropping on AMF community in subsequent soybean roots may vary depending on site or climatic conditions. In addition, our results indicated that the sample type (soil or soybean root) besides the treatment significantly affected the AMF community structure, and the interaction effect between them was also significant. Therefore, the effect of the winter cover cropping on the AMF community structure might be different between the subsequent crop roots and soil, although further investigation is necessary to reveal the cause of the difference.
Supplemental Material
Download EPS Image (1.5 MB)Acknowledgment
The authors thank Dr. Norikuni Oka, Dr. Ryo Ohtomo and Dr. Yoshihiro Kobae for critical comments and useful suggestions to polish this manuscript.
Supplementary material
Supplemental data for this article can be accessed here.
References
- An ZQ, Hendrix JW, Hershman DE, Ferriss RS, Henson GT 1993: The influence of crop rotation and soil fumigation on a mycorrhizal fungal community associated with soybean. Mycorrhiza., 3, 171–182.
- Augé RM 2001: Water relations, drought and vesicular-arbuscular mycorrhizal symbiosis. Mycorrhiza., 11, 3–42.
- Azcón-Aguilar C, Barea JM 1996: Arbuscular mycorrhizas and biological control of soil-borne plant pathogens – an overview of the mechanisms involved. Mycorrhiza., 6, 457–464.
- Berruti A, Borriello R, Orgiazzi A, Barbera AC, Lumini E, Bianciotto V 2014: Arbuscular mycorrhizal fungi and their value for ecosystem management. In Biodiversity - the Dynamic Balance of the Planet, Ed. Grillo O, pp. 159–191. InTech, Rijeka.
- Berruti A, Lumini E, Balestrini R, Bianciotto V 2016: Arbuscular mycorrhizal fungi as natural biofertilizers: let’s benefit from past successes. Front. Microbiol., 6, 1559.
- Boswell EP, Koide RT, Shumway DL, Addy HD 1998: Winter wheat cover cropping, VA mycorrhizal fungi and maize growth and yield. Agric. Ecosyst. Environ., 67, 55–65.
- Brito I, Goss MJ, De Carvalho M, Chatagnier O, Van Tuinen D 2012: Impact of tillage system on arbuscular mycorrhiza fungal communities in the soil under Mediterranean conditions. Soil Till. Res., 121, 63–67.
- Clark A 2007: Managing cover crops profitably. 3rd edn. Sustainable Agriculture Research and Education Program, MD, USA.
- Daniell TJ, Husband R, Fitter AH, Young JPW 2001: Molecular diversity of arbuscular mycorrhizal fungi colonising arable crops. FEMS Microbiol. Ecol., 36, 203–209.
- Deguchi S, Shimazaki Y, Uozumi S, Tawaraya K, Kawamoto H, Tanaka O 2007: White clover living mulch increases the yield of silage corn via arbuscular mycorrhizal fungus colonization. Plant Soil., 291, 291–299.
- Douds DD, Galvez L, Janke RR, Wagoner P 1995: Effect of tillage and farming system upon populations and distribution of vesicular-arbuscular mycorrhizal fungi. Agric. Ecosyst. Environ., 52, 111–118.
- Galvez L, Douds DD, Wagoner P, Longnecker LR, Drinkwater LE, Janke RR 1995: An overwintering cover crop increases inoculum of VAM fungi in agricultural soil. Am. J. Altern. Agric., 10, 152–156.
- Hart MM, Forsythe J, Oshowski B, Bücking H, Jansa J, Kiers ET 2013: Hiding in a crowd—does diversity facilitate persistence of a low-quality fungal partner in the mycorrhizal symbiosis? Symbiosis., 59, 47–56.
- Higo M, Isobe K, Drijber RA et al. 2014: Impact of a 5-year winter cover crop rotational system on the molecular diversity of arbuscular mycorrhizal fungi colonizing roots of subsequent soybean. Biol. Fertil. Soils., 50,913–926.
- Higo M, Isobe K, Maekawa T, Ishii R 2011: Community structure of arbuscular mycorrhizal fungi colonized in various winter crop roots. Soil Microorg., 65, 3–10.
- Higo M, Isobe K, Miyazawa Y, Matsuda Y, Drijber RA, Torigoe Y 2016: Molecular diversity and distribution of indigenous arbuscular mycorrhizal communities colonizing roots of two different winter cover crops in response to their root proliferation. J. Microbiol., 54, 86–97.
- Higo M, Isobe K, Yamaguchi M, Drijber RA, Jeske ES, Ishii R 2013: Diversity and vertical distribution of indigenous arbuscular mycorrhizal fungi under two soybean rotational systems. Biol. Fertil. Soils., 49, 1085–1096.
- Higo M, Takahashi Y, Gunji K, Isobe K 2018: How are arbuscular mycorrhizal associations related to maize growth performance during short-term cover crop rotation? J. Sci. Food Agric., 98, 1388–1396.
- Hoshino YT, Matsumoto N 2004: An improved DNA extraction method using skim milk from soils that strongly adsorb DNA. Microbes Environ., 19, 13–19.
- Isobe K, Higo M, Kondo T, Sato N, Takeyama S, Torigoe Y 2014: Effect of winter crop species on arbuscular mycorrhizal fungal colonization and subsequent soybean yields. Plant Prod. Sci., 17, 260–267.
- Jansa J, Mozafar A, Anken T, Ruh R, Sanders IR, Frossard E 2002: Diversity and structure of AMF communities as affected by tillage in a temperate soil. Mycorrhiza., 12, 225–234.
- Jansa J, Mozafar A, Kuhn G, Anken T, Ruh R, Sanders IR, Frossard E 2003: Soil tillage affects the community structure of mycorrhizal fungi in maize roots. Ecol. Appl., 13, 1164–1176.
- Jansa J, Smith FA, Smith SE 2008: Are there benefits of simultaneous root colonization by different arbuscular mycorrhizal fungi? New Phytol., 177, 779–789.
- Kabir Z, Koide RT 2002: Effect of autumn and winter mycorrhizal cover crops on soil properties, nutrient uptake and yield of sweet corn in Pennsylvania, USA. Plant Soil., 238, 205–215.
- Kabir Z, O’Halloran IP, Hamel C 1999: Combined effects of soil disturbance and fallowing on plant and fungal components of mycorrhizal corn (Zea mays L.). Soil Biol. Biochem., 31, 307–314.
- Karasawa T, Takebe M 2012: Temporal or spatial arrangements of cover crops to promote arbuscular mycorrhizal colonization and P uptake of upland crops grown after nonmycorrhizal crops. Plant Soil., 353, 355–366.
- Kruskal JB 1964: Multidimensional scaling by optimizing goodness of fit to a nonmetric hypothesis. Psychometrika., 29, 1–27.
- Lehman RM, Taheri WI, Osborne SL, Buyer JS, Douds DD Jr 2012: Fall cover cropping can increase arbuscular mycorrhizae in soils supporting intensive agricultural production. Appl. Soil Ecol., 61, 300–304.
- Njeru EM, Avio L, Sbrana C, Turrini A, Bocci G, Bàrberi P, Giovannetti M 2014: First evidence for a major cover crop effect on arbuscular mycorrhizal fungi and organic maize growth. Agron. Sustain. Dev., 34, 841–848.
- Oba H, Saito K, Fujiyoshi M 2006: Methods in arbuscular mycorrhizal research (2): observtion of arbuscular mycorrhizal fungi colonizing root. Soil Microorg., 60, 57–61.
- Oehl F, Sieverding E, Ineichen K, Mäder P, Boller T, Wiemken A 2003: Impact of land use intensity on the species diversity of arbuscular mycorrhizal fungi in agroecosystems of Central Europe. Appl. Environ. Microbiol., 69, 2816–2824.
- Oka N, Karasawa T, Okazaki K, Takebe M 2010: Maintenance of soybean yield with reduced phosphorus application by previous cropping with mycorrhizal plants. Soil Sci. Plant Nutr., 56, 824–830.
- Saito M, Ronald V 1991: Vesicular-arbuscular mycorrhizal fungi in some humus-rich Ando soils of Japan. Soil Microorg., 38, 3–15.
- Sato K, Suyama Y, Saito M, Sugawara K 2005: A new primer for discrimination of arbuscular mycorrhizal fungi with polymerase chain reaction-denature gradient gel electrophoresis. Grassland Sci., 51, 179–181.
- Shimazaki Y, Uchida T, Kobayashi H 2008: Winter barley as a cover crop affects the arbuscular mycorrhizal colonization of no-tillage soybeans. Jpn. J. Crop Sci., 77, 395–402.
- Smith SE, Read DJ, 2008: Mycorrhizal Symbiosis. 3rd ed. Academic Press Ltd, London, UK.
- Smith SE, Smith FA, Jakobsen I 2004: Functional diversity in arbuscular mycorrhizal (AM) symbioses: the contribution of the mycorrhizal P uptake pathway is not correlated with mycorrhizal responses in growth or total P uptake. New Phytol., 162, 511–524.
- Tamura K, Stecher G, Peterson D, Filipski A, Kumar S 2013: MEGA6: molecular evolutionary genetics analysis version 6.0. Mol. Biol. Evol., 30, 2725–2729.
- Tarbell TJ, Koske RE 2007: Evaluation of commercial arbuscular mycorrhizal inocula in a sand/peat medium. Mycorrhiza., 18, 51–56.
- Turrini A, Sbrana C, Avio L, Njeru EM, Bocci G, Bàrberi P, Giovannetti M 2016: Changes in the composition of native root arbuscular mycorrhizal fungal communities during a short-term cover crop-maize succession. Biol. Fertil. Soils., 52, 643–653.
- Uchida T, Kobayashi H, Yoshino N 2011: Effects of arbuscular mycorrhizal colonization on soybean nutrient uptake during ripening period with barley cover cropping. Jpn. J. Crop Sci., 80, 277–283.
- Usuki K, Yamamoto H, Tazawa J 2007: Effects of previous cropping and tillage system on growth of maize and symbiotic association with arbuscular mycorrhizal fungi in central region of Japan. Jpn. J. Crop Sci., 76, 394–400.
- Verbruggen E, Kiers ET 2010: Evolutionary ecology of mycorrhizal functional diversity in agricultural systems. Evol. Appl., 3, 547–560.
- Verbruggen E, Van Der Heijden MGA, Rillig MC, Kiers ET 2012: Mycorrhizal fungal establishment in agricultural soils: factors determining inoculation success. New Phytol., 197, 1104–1109.