ABSTRACT
The frequency and severity of drought is predicted to rise in many parts of the world. Considering that drought is the main constraint on rain-fed wheat crop production, both agronomic and genetic measures have been taken to minimize yield losses under drought. Beyond its role as a micronutrient, chloride also acts as an osmoticum, implicated in the regulation of stomatal aperture. This study explores the potential for chloride fertilization of Australian bread wheat (Triticum aestivum L.) to minimize grain yield losses caused by drought stress. For this, two drought-tolerant commercial genotypes (Mace and Gladius) and a well-studied drought-tolerant genotype used in wheat breeding (RAC875) were treated with ammonium chloride, potassium chloride, or ammonium bicarbonate, the latter two treatments served as controls for chloride and ammonium, respectively. Plants were grown under either a watered or water-restricted (drought) regime. The genotype RAC875 was found to accumulate leaf chloride at a significantly higher level than the other genotypes under optimal growth conditions. Under drought conditions, top-dressing RAC875 plants with ammonium chloride resulted in up to a 2.5-fold increase in grain number and this effect was not seen when plants were top-dressed with either of the control fertilizers. The ammonium chloride treatment also minimized losses of grain yield in RAC875 plants grown under drought. Treatment effects were accompanied by an increase in stomatal conductance. These results collectively suggest that the compound fertilizer ammonium chloride can improve drought tolerance of wheat.
1. Introduction
Drought has a major impact on agricultural productivity around the world, and the frequency and severity of drought events are projected to increase globally (Sheffield and Wood Citation2008; Torres et al. Citation2013). Plant growth and yield are affected by severe drought, such as impaired germination, inhibition of cell elongation, reduction in plant height, stem diameter, the number and size of leaves, fresh and dry biomass, induction of senescence, and an overall reduction of yield by decreasing leaf gas exchange capacity and photosynthesis (Harris et al. Citation2002; Hussain et al. Citation2008; Zhao et al. Citation2006; Khan et al. Citation2001; Farooq et al. Citation2009). Drought can be complicated by the simultaneous occurrence of water stress, high temperature, and high irradiation, as well as nutrient deficiency (Fleury et al. Citation2010). As a result, plants have developed complex strategies to combat drought, including completing their life cycle before the onset of severe water deficit, increasing water uptake, reducing transpiration rate by reducing stomatal conductance, using osmotic adjustments to maintain tissue turgor and plant growth, and inducing other drought resistance mechanisms (Izanloo et al. Citation2008). However, drought tolerance is a quantitative trait, controlled by multiple genes (Fleury et al. Citation2010). Therefore, improving a single aspect of drought tolerance, e.g., water use efficiency (WUE), may not select for high-yielding crop varieties under drought conditions (Blum Citation2005). Therefore, the focus on drought tolerance is currently shifting from plant survival to increasing yield under water-deficient conditions (Fleury et al. Citation2010).
Cl– is an essential micronutrient and plays an important role in photosynthesis, enzyme activation, nutrient transport, disease suppression, transpiration, and osmotic regulation (Engel et al. Citation1994; Freeman et al. Citation2006; Christensen et al. Citation1981; Díaz-Zorita et al. Citation2004; Lamond et al. Citation1999; Li et al. Citation2017). Glycophytes, which grow well in soil with low concentrations of salt, require between 100 and 200 µg (~2.8–5.5 µmol) g−1 shoot dry weight (DW) to avoid chlorosis, although a higher Cl– concentration may be required by halophytes (Johnson et al. Citation1957; Marschner Citation1995). Chloride is taken up mainly by active transport in the roots, and its root to shoot translocation is controlled by calcium ions and abscisic acid (ABA) (Gilliham and Tester Citation2005). Cl– is ultimately transported to the shoot, where it is involved in the oxygen-evolving complex of PSII (Kawakami et al. Citation2009) and the excess is stored inside chloroplasts (Robinson and Downton Citation1984). However, in excessive quantity, Cl– can illicit toxicity in plants, by reducing growth and inhibition of photosynthetic machinery (Wege et al. Citation2017), although the toxicity of Na+ has not been separated from that of Cl– in soybean and barley (Tavakkoli et al. Citation2010, Citation2011).
Because Cl– is a mobile element, it is prone to being leached from soil. Inland areas, where precipitation does not deliver enough Cl– to the soil, may develop Cl– deficiency (Freeman et al. Citation2006). Engel et al. (Citation1998) have established that ≥33 kg of Cl– ha−1 in the 0–60 cm soil profile is adequate for winter wheat growth, while 7.5–32 kg and <7.5 kg of Cl– ha−1 are considered to be in the deficient and critical ranges, respectively. Chloride (Cl–) fertilization in the form of NH4Cl, CaCl2, and KCl has significantly improved grain yields in winter wheat grown in Cl–-deficient soil in the Great Plains of the US (Graham et al. Citation2017; Freeman et al. Citation2006; Christensen et al. Citation1981; Díaz-Zorita et al. Citation2004; Lamond et al. Citation1999; Engel et al. Citation1994, Citation1998; Ruiz Diaz et al. Citation2012). Additionally, yield and quality improvements with KCl or potash supply to winter wheat on soils abundant in available potassium (K+) were demonstrated in the Pacific Northwest of the US, suggesting that this was a response to Cl– supply (Schaff and Skogley Citation1982; Zubriski et al. Citation1970). However, the yield responses of spring wheat to Cl– application are less clear with reports of variability at different sites, years, and cultivars (Mohr Citation1992; Engel and Grey Citation1991).
In addition to acting as a micronutrient, Cl– may act as an osmoticum. Cl– import into the vacuoles of stomatal guard cells via the activity of a vacuolar Cl– channel AtALMT9 can regulate stomatal aperture in Arabidopsis; the knock-out mutant was found to have reduced stomatal aperture, reduced transpiration rates, and improved drought tolerance (De Angeli et al. Citation2013). A recent study has indicated that foliar application of CaCl2 three times a day for 3 days alleviated drought stress in zoysiagrass (Zoysia japonica) by increasing aboveground biomass and belowground biomass, chlorophyll content, photosynthetic rate, and antioxidant responses (Xu et al. Citation2013). A similar positive effect on the growth and drought stress management of sunflower (Helianthus annuus L.) plants by foliar application of 10 mM CaCl2 was also demonstrated (Ibrahim et al. Citation2016). However, in many of these studies, the positive effect of Cl– was not distinguished from that of the cation (i.e., calcium) due to a lack of adequate controls.
In Japan and China, the use of compound fertilizers such as ammonium chloride (NH4Cl) is widespread (Zhu and Chen Citation2002; Ishikawa et al. Citation2015), although it is predominantly used as a source of nitrogen rather than Cl–. Although nitrate (NO3–) concentrations are generally 10 times higher than ammonium (NH4+) in agricultural soils, NH4+ is always present in small amounts (Marschner and Marschner Citation2012). The addition of low amounts of NH4+ relative to nitrate has been shown to stimulate growth of maize and wheat (George et al. Citation2016; Cox and Reisenauer Citation1973). The uptake and assimilation of NH4+ conserves energy when compared to the high-energy requirements for NO3– uptake and assimilation. However, when supplied at high concentrations, NH4+ can be toxic to plants, affecting their growth and development. Despite the use of these compound fertilizers, there is limited research into the role of NH4Cl application as a possible agrochemical in soils with sufficient Cl– levels to alleviate drought stress.
The overarching hypothesis of this experiment is that Cl– fertilization of wheat plants minimizes yield loss caused by short-term (cyclic) drought stress. To test this hypothesis, three drought-tolerant genotypes (RAC875, Mace and Gladius) were fertilized prior to the application of drought treatment with either of NH4Cl, KCl, or NH4HCO3. KCl was used to assess Cl–-specific effects and NH4HCO3 was used to assess NH4+-specific effects. The drought was applied at late booting, which is a critical developmental stage influencing crop yield (Schneider et al. Citation1969) and applied for 11 days before measurements were taken. The effect of top dressing wheat with NH4Cl was then assessed by studying plant growth, grain yield, photosynthetic rate, stomatal conductance, carbon isotope discrimination, as well as by leaf elemental (Cl–, K+, C, and N) analysis.
2. Materials and methods
2.1. Plant material
Two Australian bread wheat (T. aestivum L.) genotypes grown in Southern Australia (Gladius and Mace) and a breeding line from the Roseworthy Agricultural Campus (South Australia) with high grain quality, RAC875 (RAC655/3/Sr21/4*LANCE//4*BAYONET) (AGT (Australian Grain Technologies) Citation2017; Izanloo et al. Citation2008) were evaluated for growth responses to NH4Cl fertilization. The three genotypes were selected based on either extensive former studies demonstrating their drought tolerance or their current widespread commercial growth. For instance, the genotype Gladius ((DH)RAC-875/KRICHAUFF//EXCALIBUR/KUKRI/3/RAC-875/KRICHAUFF/4/RAC-875//EXCALIBUR/KUKRI[3794]) was selected by breeders for grain yield maintenance under drought stress in South Australia and released in 2007. RAC875 is a high-yielding genotype under water stress with both drought and heat tolerance traits (Fleury et al. Citation2010), although this genotype was never commercially released as a cultivar. Mace was derived from a cross between Wyalkatchem as the major parent and Stylet as the minor parent and was released in 2014. Mace is high yielding under rain-fed conditions (GRDC (Grains Research and Development Corporation) Citation2016).
2.2. Plant growth conditions
Seeds were sown into a potting mix consisting of 50% (v/v) University of California mix [50% (v/v) Waikerie river sand: 50% (v/v) peatmoss], 35% (v/v) peat mix and 15% (v/v) clay loam with slow release macronutrients (Osmocote Exact Mini 3–4 M) as calcium nitrate and single superphosphate. A total of 3.70 g of nitrogen (N), 0.80 g of phosphorous (P), and 1.89 g of potassium (K) per 9 kg of soil was adjusted to a pH of 6.0 with lime and supplied to three plants per pot (w × h; 25 cm × 23 cm, 10 kg pot capacity). A single soil sample was taken directly after mixing and a comprehensive soil analysis () was performed by Cuming Smith British Petroleum Soil & Plant Laboratory (WA, Australia).
Table 1. Chemical and physical composition of the soil used for plant growth.
The soil Cl– levels were not limiting for plant growth. Seeds were sown on 2 July 2014 and both the flag leaf and the leaf below the flag leaf harvested from the main stem of all plants at 70 DAS (heading, stages Z50–Z58). These plants minus two leaves were grown to maturity. Removal of two leaves from the main stem is likely to reduce yield of that tiller due to removal of photoassimilates; however, the yield from the remaining four to eight tillers would be unaffected. All aboveground biomass was harvested after senescence was complete. A detailed experimental time-line is provided in for clarity. Plants were grown in a glasshouse in South Australia with natural lighting (August midday light intensity range of 214 to 340 µmol m2 s−1) and controlled temperature of 22°C/18°C (day/night).
Table 2. Experimental time line and analysis over the growing period.
2.3. Experimental replication
A total of 48 pots were prepared with one seed of each genotype (RAC875, Gladius and Mace) sown together in one pot. The pots were arranged in a completely randomized design. Twelve pots were used for each of the four treatments (three fertilizer treatments and the untreated control, see Section 2.4). Six pots per treatment were designated for the dry down regime whilst the other six pots per treatment were designated for the watered regime (see Section 2.5). There were six biological plant replicates grown across six pots (n = 6).
2.4. Top-dressing with ammonium or chloride fertilizers
KCl served as a control for the Cl– effects of ammonium chloride (NH4Cl) whilst ammonium bicarbonate (NH4HCO3) served as a control for the NH4+ effects of NH4Cl. Chemical treatments were prepared to ensure that equivalent moles of NH4+ or Cl– were applied (). A 15.2 mM stock was prepared for each of NH4Cl, NH4HCO3, and KCl in reverse osmosis water. At the tillering growth stage (Z2.4), 100 mL of the appropriate fertilizer treatment was distributed evenly over the soil of a single pot, and the untreated control was supplied with 100 mL of water.
Table 3. Fertilizer treatments supplied to the soil at 47 DAS.
2.5. Watering regime
Seedlings were grown for 2 weeks with manual watering to enable optimal germination. Gravimetric soil water content (GWC) was then determined by water saturating replicate soil samples taken from pots (n = 4) after the mixture was initially prepared. Soil samples were dried at 80°C for 5 days with periodic weighting until there was no more loss in weight and GWC was calculated using Eq. (1). A soil volumetric water content (VWC) probe (Campbell Scientific, FieldScout TDR 100 Soil Moisture Meter) was used to determine VWC. Thereafter all plants were watered to weight, initially to 11% (w/v) GWC/14% VWC before applying the fertilizer treatments. Watered plants (W) were maintained at 11% GWC (w/v) by daily watering. At booting stage (all genotypes at Z41), water was withheld from dry down (D) pots until 5% (w/v) GWC/7% (w/v) VWC was reached, and then maintained by watering to this target GWC for 48 h before plant measurements were taken. Pots were rewatered to 11% (w/v) GWC/14% (w/v) VWC 4 days after measurements were taken (74 DAS).
2.6. Stomatal conductance and photosynthetic rate measurements
The photosynthetic rate and stomatal conductance of plants were measured using a portable LI-6400XT gas analysis system with a fluorescence chamber (LI-COR, Lincoln, NE, USA) set to 25°C leaf temperature, 300 µmol s−1 CO2 flow rate to the sample cell, 400 µmol CO2 mol−1 reference cell CO2, and 1500 PAR (µmol m–2 s–1). Measurements were performed on the flag leaf of the main stem from 10 AM to 1 PM over 2 days (three biological replicates per day). If the leaves were too narrow to cover the whole area of the gas exchange chamber, photosynthetic rates were corrected for actual leaf width.
2.7. Leaf chloride and potassium analysis
The leaf below the flag leaf (FL-1) was harvested from the main stem at 70 DAS to determine the concentration of Cl– and K+. Leaves were weighed (fresh weight, FW), dried at 50°C for 3 days and weighed again (DW). Dried leaves were digested with 10 mL of 1% (v/v) nitric acid at 103°C for 4 h with gentle mixing by hand every 30 min. The acid digest was analyzed for Cl– concentration using a chloride analyzer (Sherwood Scientific model 926). The leaf acid digest was diluted 1:20 for determination of K+ concentration using a Flame Photometer (Sherwood Scientific model 420) following the manufacturers’ instructions. Concentrations of Cl– and K+ were compared to measurements with known standards of NaCl and KCl, respectively.
2.8. Leaf nitrogen and carbon analysis
Dried flag leaves were ground using ball bearings (4 mm chrome steel) and a GenoGrinder (SPEX Sample Prep 2010, Metuchen, NJ, USA) at 1500 rpm until a fine powder without fibers was produced. Samples (2–2.5 mg) were weighed into tin capsules, sealed, and sent to UC Davies Stable Isotope Facility for total nitrogen, total carbon, and stable carbon isotope ratio (13C:12C) analysis via a PDZ Europa ANCA-GSL elemental analyzer interfaced to a PDZ Europa 20-20 isotope ratio mass spectrometer (Sercon Ltd., Cheshire, UK). Standards that have been previously calibrated against NIST Standard Reference Material were interspersed between plant samples. The stable carbon isotope ratio (13C:12C) of the sample was expressed relative to that of the standard UGS-41 l-glutamic acid calibrated against the international standard Vienna PeeDee Belemnite using Eq. (2). Carbon isotope discrimination (Δ) was calculated according to Hubick et al. (Citation1986) assuming an isotopic composition for the air of −8 relative to PDB:
2.9. Statistical analyses
A completely randomized design was prepared using GENSTAT (V15.0, VSN International Ltd, Hemel Hempstead, UK). Two- or three-way comparisons between genotypes and treatments (D/W, watering regime and fertilizer treatments) were made using ANOVA (GENSTAT, V15.0). All data were assessed for homoscedasticity and normality of distribution. Means were compared and letters assigned using two-way ANOVA with Bonferroni post-hoc (GENSTAT, V15.0). Graphs were prepared in GraphPad Prism version 7.0 (GraphPad Software, La Jolla, CA, USA).
3. Results
3.1. Ammonium chloride supply minimizes yield losses in RAC875 plants grown under drought
The aims here were to, firstly, compare the drought tolerance of the three genotypes and, secondly, to identify any ameliorating effects under drought when plants were top-dressed with NH4Cl as compared to the respective controls (KCl, NH4HCO3) and the untreated control. The flowering of Mace plants 77.5 days after sowing (DAS) was slightly delayed when averaged across all treatments and watering regimes as compared to RAC875 (73.5 DAS) and Gladius (73.9 DAS) (genotype effect at p < 0.05, ). Mace plants grown under either watered (W) or drought (D) conditions constitutively produced greater shoot biomass, more tillers and spikes than the other genotypes when averaged across all treatments (genotype effect at p < 0.05, ). Under W conditions, untreated Mace plants produced the highest average grain number and yield than the other genotypes (p < 0.05), whereas RAC875 and Gladius plants had similar grain numbers and yield ( and ). As expected, this was reflected in the higher thousand grain number per spike of Mace plants grown under W conditions as compared to the other genotypes when averaged across treatments (genotype effect and D/W effect at p < 0.05, ). Under D conditions, Mace also outperformed the other genotypes when averaged across all treatments; Mace plants produced the highest average grain numbers and yield (166 seeds plant−1 and 9.0 g plant−1) than RAC875 (136 seeds plant−1 and 6.5 g plant−1) and Gladius (120 seeds plant−1 and 5.8 g plant−1) (genotype effect at p < 0.05 with SED of 11.2 for grain number and 0.57 for grain yield; and e).
Table 4. Wheat growth responses to drought and fertilization at maturity, mean ± SEM (n = 6).
Figure 1. The effect of drought and fertilizer treatments on grain number and yield. Three genotypes (RAC875, Gladius and Mace) were grown to maturity and the following components measured. (a, b) Grain number, (d–e) grain yield, and (c, f) % loss of grain number and grain yield under drought (W/D*100) (n = 6, mean ± SEM). Statistical significance within a graph is indicated by different letters determined by two-way ANOVA with Bonferroni post-hoc analysis (p < 0.05).
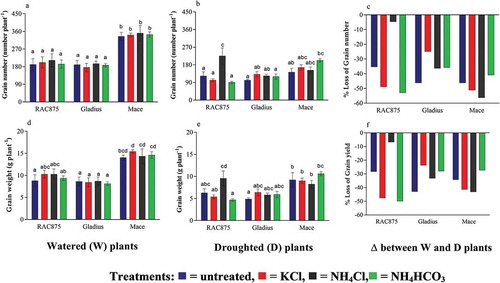
There was a 1.8- to 2.5-fold increase in the grain number of droughted RAC875 plants when treated with NH4Cl as compared to the untreated, KCl or NH4HCO3 treated plants (p < 0.05, ). The ameliorating effect of NH4Cl on grain number of RAC875 plants is also reflected in the minimization of loss of grain number (c). The grain yield of droughted RAC875 plants was significantly greater when treated with NH4Cl as compared to KCl or NH4HCO3 (c); however, it was not significantly greater than that of the untreated RAC875 plants. However, the supply of NH4Cl to droughted RAC875 clearly minimized loss of grain yield (−6.8%, Δ Grain yield, ) as compared to the untreated control (−28.4%, Δ Grain yield, ).
3.2. Ammonium chloride fertilization improves stomatal conductance of droughted wheat
Regulation of stomatal conductance is a key drought tolerance mechanism, allowing plants to reduce water loss due to transpiration while optimizing CO2 assimilation (Tombesi et al. Citation2015). In this study, the ability of plants to maintain stomatal conductivity during drought as a result of top-dressing with NH4Cl was assessed. Average stomatal conductance in the watered plants ranged between 0.41 and 0.49 mol H2O m−2 s−1 () while that in the droughted plants ranged between 0.06 and 0.18 mol H2O m−2 s−1 (), exhibiting a two to eightfold decrease under D conditions (p < 0.05). There was no significant difference in the stomatal conductance between the untreated and fertilizer-treated plants when grown under W conditions (a). However, under D conditions, there was a significant increase in the average stomatal conductance of NH4Cl-treated RAC875 plants when compared to the untreated and KCl-treated plants (p < 0.05, b). However, stomatal conductance was not significantly different between NH4Cl and NH4HCO3-treated RAC875 plants grown under D conditions.
Figure 2. The physiological effect of drought and fertilization. The flag leaf from the main stem was assessed for (a, b) stomatal conductance, (c, d) photosynthesis, and (d, e) carbon isotope discrimination (Δ13C) in three wheat genotypes (RAC875, Gladius and Mace) at 70 days after sowing (n = 6, mean ± SEM). Statistical significance within a graph is indicated by different letters determined by two-way ANOVA with Bonferroni post-hoc analysis (p < 0.05). Graphs without letters indicate that means were not significantly different.
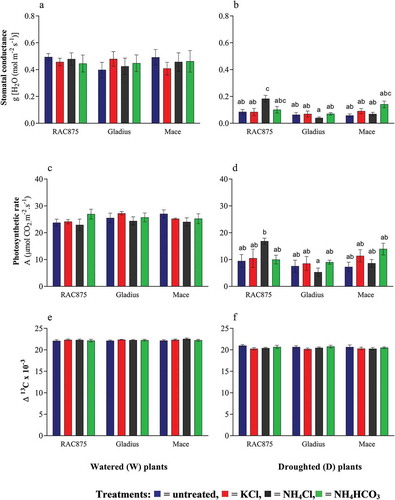
Photosynthetic assimilation may be affected by drought as a result of stomatal closure and decreased CO2 availability or indirectly as a result of oxidative damage, affecting the photosynthetic capacity of the plant (Chaves et al. Citation2009; Fischer et al. Citation1998). The average photosynthetic rate of the watered plants across all treatments (25.2 µmol CO2 m−2 s−1) was significantly higher than that of the droughted plants (9.9 µmol CO2 m−2 s−1) (p < 0.05, and d). There were no significant differences observed in the average photosynthetic rate among treatments and genotypes in the watered plants (c). Droughted RAC875 plants treated with NH4Cl had a higher photosynthetic rate than droughted Gladius treated with NH4Cl (p < 0.05, ); however, this was not significantly greater than the untreated plants (d).
Carbon isotope discrimination (Δ13C) measurements are used as an indication of the WUE of wheat genotypes (Zhu et al. Citation2008; Farquhar et al. Citation1989; Zhang et al. Citation2015). It is a time-integrated measurement of the ratio between internal and external CO2 concentrations (Corneo et al. Citation2016). Considering that NH4Cl fertilizer supply to droughted RAC875 plants resulted in greater stomatal conductance at 70 DAS, Δ13C was assessed here to determine whether this translated to improved WUE. Δ13C in plants grown under W conditions was significantly higher when averaged across treatments and genotypes (20.5 × 103, e) than that observed from plants grown under D conditions (22.3 × 103) (p < 0.05, f). However, Δ13C did not significantly vary as a result of the treatment in any of the three genotypes grown under W conditions (e). Although a trend of slightly reduced Δ13C was observed for the KCl and NH4Cl-treated RAC875 plants as compared to untreated control plants (mean differences of −0.72 × 10−3 and −0.57 × 10−3, respectively), this was not found to be significant (f).
To assess whether the NH4Cl treatment assisted with the recovery of droughted plants, water was resupplied at 74 DAS until the soil water content was equivalent between the droughted and the watered plants. Plants were assessed 52 h after rewatering (77 DAS), and the results demonstrated that rewatering short-term droughted plants enabled them to recover their photosynthetic rate and stomatal conductance to levels equivalent to plants grown under watered conditions (Fig. S1a–d). There were however no significant effects of the fertilizer treatment on stomatal conductance or photosynthetic rate 52 h after rewatering plants that were grown in either condition (Fig. S1a–d). Droughted and rewatered Mace plants had significantly higher stomatal conductance and photosynthetic rate when averaged across treatments as compared to Gladius grown under the same conditions (Fig. S1b and d, p < 0.05) but they were not significantly different from RAC875 (Fig. S1b and d).
3.3. Ammonium fertilization treatments do not alter the N nutritional status of the flag leaf
The drought treatment caused a significant decrease in flag leaf FW and DW in all genotypes examined as compared to the well-watered treatment (Fig. S2a compared to S2b, p < 0.05 and S2c compared to S2d, p < 0.05). Mace plants had a significantly smaller flag leaf than RAC875 under both W and D conditions when averaged across treatments (p < 0.05, Fig. S2c and d), which was not significantly different from Gladius (Fig. S2c and S2d). The flag leaf of droughted Mace plants treated with NH4Cl was also smaller than droughted RAC875 plants treated with NH4Cl; however within a genotype, there were no significant differences between fertilizer treatments (Fig. S2d).
In this study, the concentration of nitrogen (N) in the flag leaf at 70 DAS was analyzed to assess the nutritional effect of ammonium fertilizer addition whilst the concentration of carbon (C) was analyzed to assess whether drought stress triggered premature leaf senescence (Chen et al. Citation2015) (Fig. S3a–d). Mace and Gladius plants grown under W conditions had higher leaf C and N concentrations than watered RAC875 plants when averaged across treatments (p < 0.05, Fig. S3a and c). However, there were no genotypic differences under D conditions (Fig. S3b and d). There was also no significant effect of the fertilizers on leaf C or N concentration of any genotype under W or D conditions (Fig. S3a–d).
3.4. RAC875 plants accumulate higher leaf chloride levels
Osmolytes or solutes exert a protective function by allowing for osmotic adjustments under drought stress and by delaying dehydration related cellular damage (Ibrahim et al. Citation2016). It has been suggested that Cl– can act as an osmolyte under drought stress as well as help to regulate stomatal opening (De Angeli et al. Citation2013). The opening and closing of stomata is also regulated by fluxes of K+ and anions such as malate. The concentration of Cl– (a and b) and K+ (c and d) in the leaf below the flag leaf (FL-1) was assessed to investigate if (1) genotypic differences in Cl– concentration exist and if (ii) Cl– or K+ fertilization caused an increase in Cl– or K+ concentration above that of the control. When averaged across all treatments, RAC875 plants accumulated the highest leaf Cl– concentration when grown under the watered condition as compared to the other genotypes (p < 0.05, a). However, under D conditions, RAC875 and Mace plants accumulated equivalent levels of chloride when averaged across all treatments (p > 0.05, b), which was greater than that found in Gladius leaves (p < 0.05, b). RAC875 plants also accumulated more leaf Cl– under W conditions than under D conditions (p < 0.05). There was no significant effect of the fertilizer treatments on leaf Cl– concentration under either W or D conditions (b, which was anticipated as the Cl– supply in the soil was not deficient. Leaf K+ concentrations were similar between genotypes and fertilizer treatments. However, overall leaf K+ concentration was increased in droughted plants as compared to that in watered plants (p < 0.05, e and f).
Figure 3. The effects of drought and fertilization on flag leaf accumulation of potassium and chloride. The concentration of (a, b) chloride and (c, d) potassium in the leaf below the flag leaf (FL-1) of three wheat genotypes (RAC875, Gladius and Mace) at 70 days after sowing (n = 6, mean ± SEM). Statistical significance within a graph is indicated by different letters determined by two-way ANOVA with Bonferroni post-hoc analysis (p < 0.05). Graphs without letters indicate that means were not significantly different.
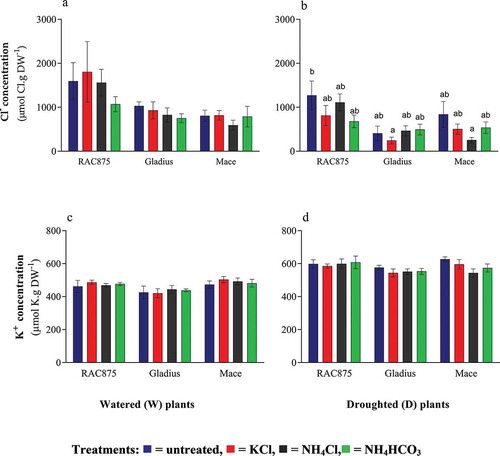
4. Discussion
Mace was the highest yielding genotype under both watered and drought conditions in this present study as supported by McDonald and Rebecca (Citation2016) irrigated and rain-fed field trials in South Australia (2011–2014) and in Australia’s National Variety Trials (GRDC (Grains Research and Development Corporation) Citation2016). Mahjourimajd et al. (Citation2016) also described Mace as high yielding in low nitrogen environments. The yield performance of Mace under both watered and drought conditions here was explained by more spikes leading to higher grain numbers.
Considering that at least half of the increase in yields of dryland-grown wheat in Australia is attributed to improved agronomic management (Turner Citation2004), we investigated whether top-dressing Australian wheat with NH4Cl could improve drought tolerance above that already afforded by breeding. RAC875 was the only genotype examined here to positively respond to NH4Cl supply under drought conditions. RAC875 is a high-yielding genotype under water stress with both drought and heat tolerance traits (Fleury et al. Citation2010). RAC875 has been described as having conservative mechanisms of drought tolerance in part due to the characteristics of low tiller number but high number of grains per tiller, thick green leaves with strong waxiness that stay green under water stress, moderate osmotic adjustment, low stomatal conductance, slower recovery in stomatal conductance, and constitutively high levels of water soluble carbohydrates (Fleury et al. Citation2010; Izanloo et al. Citation2008). Here we demonstrated that NH4Cl supply to droughted RAC875 plants minimized losses in grain yield and grain number as compared to the untreated and both the NH4+ control (NH4HCO3) and the Cl– control (KCl). Interestingly, RAC875 plants accumulated higher leaf chloride levels than Mace and Gladius plants under watered conditions, and those levels were reduced under drought in this genotype only. This may indicate that RAC875 had higher Cl– uptake efficiency under watered conditions, thereby taking up the fertilizer when it was supplied prior to the drought treatment; however, this would need to be verified with Cl– uptake studies.
Plants control water loss and CO2 uptake by regulating the opening and closing of stomatal aperture. Stomatal closure occurs when plant roots sense the drying soil that can lead to reduced transpiration and thereby water use but it also negatively affects photosynthesis and hence biomass production. In short-term drought scenarios experienced during reproductive stages of development, maintenance of stomatal conductance may allow plants to continue assimilating CO2 and remobilizing carbon to the grain. In this study, top-dressing RAC875 plants grown under water-deficit conditions with NH4Cl resulted in higher stomatal conductance at 70 DAS. Stomatal aperture is regulated by changes in the osmotic potential of guard cells by ion transport across membranes. Potassium uptake from the apoplast and accumulation in the vacuole is, for instance, critical for stomatal opening (Lebaudy et al. Citation2007). Potassium concentrations were found in this study to be higher in leaves of droughted plants than watered plants; K+ accumulation is suggested to be an osmotic adjustment mechanism adopted by plants in water-deficient conditions (Farooq et al. Citation2009). However, the positive charge in the vacuole must be balanced by the accumulation of anions such as Cl–, malate, and NO3− (Wege et al. Citation2017). Cl– flux across the vacuolar membrane of guard cells by the activity of a malate-induced Cl– channel AtALMT9 was reported to be essential for stomata opening in Arabidopsis. AtALMT9 knock-out plants had reduced drought tolerance and transpiration caused by reduced stomatal aperture and slower opening kinetics (De Angeli et al. Citation2013). Cl– also has an essential role in photosynthesis, where two Cl– ions are required in each oxygen-evolving complex of PSII (Kawakami et al. Citation2009). Top-dressing RAC875 plants grown under water deficit with NH4Cl did not however lead to improved photosynthetic rates.
Wheat varieties have been bred specifically for performance in drought-prone environments using carbon isotope discrimination (Δ13C) measurements as an indirect measure of transpiration efficiency (Condon et al. Citation2006). Considering that all genotypes used in the present study were selected for yield in drought-prone environments, it was not surprising that there was no genotypic variation for Δ13C. Leaf Δ13C is positively related to intracellular CO2 concentration (Ci) and Ci is determined by the ratio between stomatal conductance and the rate of photosynthesis (Condon et al. Citation1992). Δ13C is thereby negatively associated with WUE (Ehdaie et al. Citation1991). In this study, NH4Cl supply to RAC875 led to significant increases in stomatal conductance and slight but nonsignificant increases in photosynthesis, which may explain why the treatment effect on Δ13C effect was not significant. Due to the design of this current experiment (three genotypes per pot), improvement in WUE for individual genotypes could not be confirmed. The effect of NH4Cl supply on stomatal aperture and WUE of RAC875 should be further explored.
It was encouraging from the present study that even a small amount of Cl– supplied in the form of NH4Cl could improve stomatal conductance and photosynthesis of wheat plants grown in Cl–-sufficient soils above that of the untreated controls. Cl– influx across the plasma membrane of roots can occur passively via Cl– channels under saline conditions (Skerrett and Tyerman Citation1994); or via proton (H+)/Cl– symporters in low salinity conditions, the latter is more relevant to the soil Cl– rates supplied in this study. The fertilizer treatments supplied 7.2 × 10−3 mg Cl– per kg soil (0.203 × 10−3 mmoles Cl– per kg soil), which was 0.005% of the basal Cl– levels (155.7 mg Cl– per kg soil/4.39 mmoles Cl– per kg soil). Flag leaf analysis at 70 DAS showed that droughted but untreated plants accumulated 4.3–8.9 µmol Cl– per gram DW, which is within the sufficiency range for this micronutrient (Marschner and Marschner Citation2012). Therefore, improvements from top-dressing RAC875 seedlings with NH4Cl were unrelated to any Cl– limitations or deficiencies. We suggest that the positive effects on wheat could be due to the role of Cl– ions and their transport into the vacuoles of stomatal guard cells.
Since roots are in direct contact with drying soil, it has been suggested that roots produce a transmittable chemical signal to regulate stomatal aperture. One candidate for that signal is ABA, a well-known regulator of stomatal conductance (Mittelheuser and Van Steveninck Citation1969) that is reported to accumulate in response to soil water deficit in wheat (Wright Citation1969) and maize (Bahrun et al. Citation2002). ABA also regulates Cl– efflux to the xylem; in vitro patch clamp experiments with extracellular ABA supply and intracellular calcium supply reduced passive Cl– and NO3– efflux from root stellar protoplasts in barley (Gilliham and Tester Citation2005), effectively indicating reduced xylem loading of Cl–. Cl– efflux from xylem parenchyma and pericyclic cells to the root xylem apoplast is a key rate limiting step and is facilitated by SLAH1/SLAH3 (Slow-type Anion Channel-associated proteins), (Wege et al. Citation2017). At this stage, it is not known how Cl– efflux into the xylem is affected by drought, nor the role of root to shoot signals in amplifying this response. Additionally, interactions between the root distributions of the three genotypes grown within the one pot must also be considered here, particularly since frequent irrigation to maintain soil water deficit could enhance the dependence of plant water relations on root distribution (Puértolas et al. Citation2017). Schoppach et al. (Citation2014) suggested that the water conservation phenotype of RAC875 was related to its lower root hydraulic conductivity when compared to a drought-sensitive genotype Kukri. However, the root distribution and hydraulic conductivity of RAC875 has yet to be compared to Gladius or Mace.
Considering that the stimulation of stomatal conductance was above that of the untreated control and the Cl– control (KCl) but not NH4+ control (NH4HCO3), the effects of Cl– cannot be separated from that of NH4+. Top-dressing with the fertilizers supplied 5.58 mg N as NH4+ (0.398 mmol N per kg soil), which was only 8.1% of the total N supplied in the form of calcium nitrate prior to seed germination (109 mg N per kg of soil/4.92 mmol N per kg soil). However, we know that small amounts of NH4+ supplied to plants are taken up readily and preferentially to NO3– due to the lower energetic costs of transporting NH4+ (George et al. Citation2016; Cox and Reisenauer Citation1973). Top-dressing with this small amount of NH4+ containing fertilizer may provide some N-related nutritional benefits for the plant above that of the untreated controls although only leaf N concentration rather than total plant N was determined.
Although reports have shown that Cl– fertilization improves grain yields in Cl–-deficient soil (Graham et al. Citation2017; Freeman et al. Citation2006; Christensen et al. Citation1981; Díaz-Zorita et al. Citation2004; Lamond et al. Citation1999; Engel et al. Citation1994), the effect of Cl– supply on the performance of plants grown in conditions of Cl– sufficiency is poorly understood. More recent papers have demonstrated some positive effects of Cl– supply on plant growth (Xu et al. Citation2013; Ibrahim et al. Citation2016), although the effect of the cation in the compound fertilizers supplied could not be separated from that of Cl–. In this study, despite the presence of adequate controls, the effect of Cl– on improved drought tolerance of bread wheat could not be easily separated from that of NH4+. Although in an agronomic setting a compound fertilizer such as ammonium chloride would be advantageous as it is already utilized in some countries as a source of nitrogen.
This research demonstrates that top-dressing the wheat genotype RAC875 with NH4Cl can mitigate yield losses under drought and this in itself justifies further exploration of the agronomic potential of NH4Cl fertilizers on wheat performance in water-limited environment. A large number of genotypes should be screened in both controlled and field conditions to explore and explain the interaction between genotypes and the NH4Cl fertilizer treatment.
Supporting_Information.docx
Download MS Word (720.8 KB)Acknowledgments
F.K. is supported by Commonwealth Government Research Training Program Domestic (RTPd) Fee Offset and MF & MH Joyner Scholarship in Science.
Disclosure statement
The authors declare that no financial interests or benefit have arisen from the direct application of this research.
Supplementary material
Supplemental data for this article can be accessed here.
Additional information
Funding
Notes on contributors
Farzana Kastury
S.H. and V.M. conceived and designed the experiments and supervised F.K, performed the preliminary experiments. V.E. and A.E. performed the final experiments; F.K. and V.M. analyzed the data and co-wrote the paper; V.M., M.O. and S.H. edited the manuscript.
References
- AGT (Australian Grain Technologies). 2017. “Our varieties. Wheat.” http://www.agtbreeding.com.au/varieties/wheat#mace.
- Bahrun A, Jensen CR, Asch F, Mogensen VO 2002: Drought‐induced changes in xylem pH, ionic composition, and ABA concentration act as early signals in field‐grown maize (Zea mays L.). Journal of Experimental Botany. 53(367), 251–263.
- Blum A 2005: Drought resistance, water-use efficiency, and yield potential - are they compatible, dissonant, or mutually exclusive? Australian Journal of Agricultural Research. 56(11), 1159–1168. doi:10.1071/AR05069
- Chaves MM, Flexas J, Pinheiro C 2009: Photosynthesis under drought and salt stress: regulation mechanisms from whole plant to cell. Annals of Botany. 103(4), 551–560. doi:10.1093/aob/mcn125
- Chen D, Wang S, Xiong B, Cao B, Deng X 2015: Carbon/nitrogen imbalance associated with drought-induced leaf senescence in Sorghum bicolor. PloS One. 10(8), e0137026. doi:10.1371/journal.pone.0137026
- Christensen NW, Taylor RG, Jackson TL, Mitchell BL 1981: Chloride effects on water potentials and yield of winter wheat infected with take-all root rot. Agronomy Journal. 73(6), 1053–1058. doi:10.2134/agronj1981.00021962007300060035x
- Condon AG, Richards RA, Farquhar GD 1992: The effect of variation in soil water availability, vapour pressure deficit and nitrogen nutrition on carbon isotope discrimination in wheat. Australian Journal of Agricultural Research. 43(5), 935–947. doi:10.1071/AR9920935
- Condon AG, Farquhar GD, Rebetzke GJ, Richards RA 2006: The application of carbon isotope discrimination in cereal improvement for water-limited environments. Drought Adaptation in Cereals. 6, 171–219.
- Corneo PE, Suenaga H, Kertesz MA, Dijkstra FA 2016: Effect of twenty four wheat genotypes on soil biochemical and microbial properties. Plant and Soil. 404(12), 141–155. doi:10.1007/s11104-016-2833-1
- Cox WJ, Reisenauer HM 1973: Growth and ion uptake by wheat supplied nitrogen as nitrate, or ammonium, or both. Plant and Soil. 38(2), 363–380. doi:10.1007/BF00779019
- De Angeli A, Zhang J, Meyer S, Martinoia E 2013: AtALMT9 is a malate-activated vacuolar chloride channel required for stomatal opening in Arabidopsis. Nature Communications. 4, 1804. doi:10.1038/ncomms2815
- Díaz-Zorita M, Duarte GA, Barraco M 2004: Effects of chloride fertilization on wheat (Triticum aestivum L.) productivity in the Sandy Pampas Region, Argentina. Agronomy Journal. 96(3), 839–844. doi:10.2134/agronj2004.0839
- Ehdaie B, Hall AE, Farquhar GD, Nguyen HT, Waines JG 1991: Water-use efficiency and carbon isotope discrimination in wheat. Crop Science. 31(5), 1282–1288. doi:10.2135/cropsci1991.0011183X003100050040x
- Engel RE, Bruckner PL, Eckhoff J 1998: Critical tissue concentration and chloride requirements for wheat. Soil Science Society of America Journal. 62(2), 401–405. doi:10.2136/sssaj1998.03615995006200020016x
- Engel RE, Grey WE 1991: Chloride fertilizer effects on winter wheat inoculated with Fusarium culmorum. Agronomy Journal. 83(1), 204–208. doi:10.2134/agronj1991.00021962008300010048x
- Engel RE, Eckhoff J, Berg RK 1994: Grain yield, kernel weight, and disease responses of winter wheat cultivars to chloride fertilization. Agronomy Journal. 86(5), 891–896. doi:10.2134/agronj1994.00021962008600050025x
- Farooq M, Wahid A, Kobayashi N, Fujita D, Basra SMA 2009: Plant drought stress: effects, mechanisms and management. Agronomy for Sustainable Development. 29(1), 185–212. doi:10.1051/agro:2008021
- Farquhar GD, Hubick KT, Condon AG, Richards RA 1989: Carbon Isotope Fractionation and Plant Water-Use Efficiency. In: Rundel P.W., Ehleringer J.R., Nagy K.A. (eds) Stable Isotopes in Ecological Research. Ecological Studies (Analysis and Synthesis), vol. 68. Springer, New York.
- Fischer RA, Rees D, Sayre KD, Lu Z-M, Condon AG, Larque Saavedra A 1998: Wheat yield progress associated with higher stomatal conductance and photosynthetic rate, and cooler canopies. Crop Science. 38(6), 1467–1475. doi:10.2135/cropsci1998.0011183X003800060011x
- Fleury D, Jefferies S, Kuchel H, Langridge P 2010: Genetic and genomic tools to improve drought tolerance in wheat. Journal of Experimental Botany. 61(12), 3211–3222. doi:10.1093/jxb/erq152
- Freeman KW, Girma K, Mosali J, Teal RK, Martin KL, Raun WR 2006: Response of winter wheat to chloride fertilization in sandy loam soils. Communications in Soil Science and Plant Analysis. 37(1314), 1947–1955. doi:10.1080/00103620600767231
- George J, Holtham L, Sabermanesh K, Heuer S, Tester M, Plett D, Garnett T 2016: Small amounts of ammonium (NH4+) can increase growth of maize (Zea mays). Journal of Plant Nutrition and Soil Science. 179(6), 717–725. doi:10.1002/jpln.201500625
- Gilliham M, Tester M 2005: The regulation of anion loading to the maize root xylem. Plant Physiology. 137(3), 819–828. doi:10.1104/pp.104.054056
- Graham C, Woodard H, Bly A, Fixen P, Gelderman R 2017: Chloride fertilizers increase spring wheat yields in the Northern Great Plains. Agronomy Journal. 109(1), 327–334. doi:10.2134/agronj2016.04.0205
- GRDC (Grains Research and Development Corporation) 2016: Long term MET yield reporter. https://dev.app.nvtonline.com.au/
- Harris D, Tripathi RS, Joshi A 2002: On farm seed priming to improve crop establishment and yield in dry direct seeded rice. In Direct Seeding: research Strategies and Opportunities, edited by, Pandey S, Mortimer M, Wade L, Tuong TP, Lopes K, Hardy B. pp. 231–240, International Research Institute, Manila, Philippines.
- Hubick KT, Farquhar GD, Shorter R 1986: Correlation between water-use efficiency and carbon isotope discrimination in diverse peanut (Arachis) germplasm. Functional Plant Biology. 13(6), 803–816.
- Hussain M, Malik MA, Farooq M, Ashraf MY, Cheema MA 2008: Improving drought tolerance by exogenous application of glycinebetaine and salicylic acid in sunflower. Journal of Agronomy and Crop Science. 194(3), 193–199. doi:10.1111/j.1439-037X.2008.00305.x
- Ibrahim MFM, Faisal A, Shehata SA 2016: Calcium chloride alleviates water stress in sunflower plants through modifying some physio-biochemical parameters. American-Eurasian J. Agric. & Environ. Sci. 16(4), 677–693.
- Ishikawa N, Ishioka G, Yanaka M, Takata K, Murakami M 2015: Effects of ammonium chloride fertilizer and its application stage on cadmium concentrations in wheat (Triticum aestivum L.) grain. Plant Production Science. 18(2), 137–145. doi:10.1626/pps.18.137
- Izanloo A, Condon AG, Langridge P, Tester M, Schnurbusch T 2008: Different mechanisms of adaptation to cyclic water stress in two South Australian bread wheat cultivars. Journal of Experimental Botany. 59(12), 3327–3346. doi:10.1093/jxb/ern199
- Johnson CM, Stout PR, Broyer TC, Carlton AB 1957: Comparative chlorine requirements of different plant species. Plant and Soil. 8, 337–353. doi:10.1007/BF01666323
- Kawakami K, Umena Y, Kamiya N, Shen JR 2009: Location of chloride and its possible functions in oxygen-evolving photosystem II revealed by X-ray crystallography. Proceedings of the National Academy of Sciences. 106(21), 8567–8572. doi:10.1073/pnas.0812797106
- Khan MB, Hussain N, Iqbal M 2001: Effect of water stress on growth and yield components of maize variety YHS 202. Journal of Research (Science). 12(1), 15–18.
- Lamond RE, Roberson DD, Rector K 1999: Chloride fertilization bumps wheat yields, profits. Fluid Journal. 7(27), 14–15.
- Lebaudy A, Véry A-A, Sentenac H 2007: K+ channel activity in plants: genes, regulations and functions. FEBS Letters. 581(12), 2357–2366. doi:10.1016/j.febslet.2007.03.058
- Li B, Tester M, Gilliham M 2017: Chloride on the Move. Trends in Plant Science. 22(3), 236–248. doi:10.1016/j.tplants.2016.12.004
- Mahjourimajd S, Taylor J, Sznajder B, Timmins A, Shahinnia F, Rengel Z, Khabaz-Saberi H, Kuchel H, Okamoto M, Langridge P 2016: Genetic basis for variation in wheat grain yield in response to varying nitrogen application. PloS One. 11(7), e0159374. doi:10.1371/journal.pone.0159374
- Marschner H 1995: Mineral Nutrition of Higher Plants, 2nd ed. Elsevier, Amsterdam.
- Marschner H, Marschner P 2012: Marschner’s Mineral Nutrition of Higher Plants, 3rd. Elsevier Academic Press, London.
- McDonald G, Rebecca O 2016: Characterising Varietal Responses of Wheat to Drought Tolerance, Grains Research Update, Grains Research and Development Corporation, Australia.
- Mittelheuser CJ, Van Steveninck RFM 1969: Stomatal closure and inhibition of transpiration induced by (RS)-abscisic acid. Nature. 221(5177), 281–282. doi:10.1038/221281a0
- Mohr RM. 1992. “The effect of chloride fertilization on growth and yield of barley and spring wheat.” Thesis, The University of Manitoba, https://mspace.lib.umanitoba.ca/handle/1993/17473.
- Puértolas J, Larsen EK, Davies WJ, Dodd IC 2017: Applying ‘drought’to potted plants by maintaining suboptimal soil moisture improves plant water relations. Journal of Experimental Botany. 68(9), 2413–2424. doi:10.1093/jxb/erx116
- Robinson SP, Downton WJ 1984: Potassium, sodium, and chloride content of isolated intact chloroplasts in relation to ionic compartmentation in leaves. Archives of Biochemistry and Biophysics. 228, 197–206. doi:10.1016/0003-9861(84)90061-4
- Ruiz Diaz DA, Mengel DB, Lamond RE, Duncan SR, Whitney DA, Maxwell TM 2012: Meta-analysis of winter wheat response to chloride fertilization in Kansas. Communications in Soil Science and Plant Analysis. 43(18), 2437–2447. doi:10.1080/00103624.2012.708077
- Schaff BE, Skogley EO 1982: Diffusion of potassium, calcium, and magnesium in Bozeman silt loam as influenced by temperature and moisture. Soil Science Society of America Journal. 46(3), 521–524. doi:10.2136/sssaj1982.03615995004600030015x
- Schneider AD, Musick JT, Dusek DA 1969: Efficient wheat irrigation with limited water. Transactions of the ASAE. 12(1), 23–0026. doi:10.13031/2013.38752
- Schoppach R, Wauthelet D, Jeanguenin L, Sadok W 2014: Conservative water use under high evaporative demand associated with smaller root metaxylem and limited trans-membrane water transport in wheat. Functional Plant Biology. 41(3), 257–269. doi:10.1071/FP13211
- Sheffield J, Wood EF 2008: Projected changes in drought occurrence under future global warming from multi-model, multi-scenario, IPCC AR4 simulations. Climate Dynamics. 31(1), 79–105. doi:10.1007/s00382-007-0340-z
- Skerrett M, Tyerman SD 1994: A channel that allows inwardly directed fluxes of anions in protoplasts derived from wheat roots. Planta. 192(3), 295–305. doi:10.1007/BF00198563
- Tavakkoli E, Fatehi F, Coventry S, Rengasamy P, McDonald GK 2011: Additive effects of Na+ and Cl– ions on barley growth under salinity stress. Journal of Experimental Botany. 62, 2189–2203. doi:10.1093/jxb/err025
- Tavakkoli E, Rengasamy P, McDonald GK 2010: High concentrations of Na+ and Cl– ions in soil solution have simultaneous detrimental effects on growth of faba bean under salinity stress. Journal of Experimental Botany. 61, 4449–4459. doi:10.1093/jxb/erp353
- Tombesi S, Andrea N, Tommaso F, Marta S, Daniela F, Stefano P, Alberto P 2015: Stomatal closure is induced by hydraulic signals and maintained by ABA in drought-stressed grapevine. Scientific Reports. 5, 12449.
- Torres GM, Lollato RP, Ochsner TE 2013: Comparison of drought probability assessments based on atmospheric water deficit and soil water deficit. Agronomy Journal. 105(2), 428–436. doi:10.2134/agronj2012.0295
- Turner NC 2004: Sustainable production of crops and pastures under drought in a Mediterranean environment. Annals of Applied Biology. 144(2), 139–147. doi:10.1111/j.1744-7348.2004.tb00327.x
- Wege S, Gilliham M, Henderson SW 2017: Chloride: not simply a ‘cheap osmoticum’, but a beneficial plant macronutrient. Journal of Experimental Botany. 68(12), 3057–3069. doi:10.1093/jxb/erx050
- Wright STC 1969: An increase in the “inhibitor-β” content of detached wheat leaves following a period of wilting. Planta. 86(1), 10–20. doi:10.1007/BF00385299
- Xu C, Xuemei L, Zhang L 2013: The effect of calcium chloride on growth, photosynthesis, and antioxidant responses of Zoysia japonica under drought conditions. PloS One. 8(7), e68214. doi:10.1371/journal.pone.0068214
- Zhang G, Aiken R, Martin TJ 2015: Relationship between carbon isotope discrimination and grain yield of rainfed winter wheat in a semi-arid region. Euphytica. 204(1), 39–48. doi:10.1007/s10681-014-1335-6
- Zhao TJ, Sun S, Liu Y, Liu JM, Liu Q, Yan YB, Zhou HM 2006: Regulating the drought responsive element (DRE) mediated signaling pathway by synergic functions of trans-active and transinactive DRE binding factors in Brassica napus. Journal of Biololical Chemistry. 281, 10752–10759. doi:10.1074/jbc.M510535200
- Zhu L, Liang ZS, Xu X, Li SH 2008: Relationship between carbon isotope discrimination and mineral content in wheat grown under three different water regimes. Journal of Agronomy and Crop Science. 194(6), 421–428.
- Zhu ZL, Chen DL 2002: Nitrogen fertilizer use in China – contributions to food production, impacts on the environment and best management strategies. Nutrient Cycling in Agroecosystems. 63(2), 117–127. doi:10.1023/a:1021107026067
- Zubriski JC, Vasey EH, Norum EB 1970: Influence of nitrogen and potassium fertilizers and dates of seeding on yield and quality of malting barley. Agronomy Journal. 62(2), 216–219. doi:10.2134/agronj1970.00021962006200020012x