ABSTRACT
In this study, the effects of penconazole (PEN) and calcium (Ca) on growth amelioration and quality of seed oil in canola (Brassica napus L.) under drought stress were investigated. Drought stress reduced the growth parameters (fresh weight and dry weight) in canola; however the application of PEN and Ca improved these parameters under drought condition. Inducing effect of Ca on protein content was more prominent than PEN. Proline content increased under drought stress and PEN and Ca treatment caused more induction it under drought. PEN and Ca alleviated the negative effects of drought stress in canola by inducing antioxidant defense. The application of PEN and Ca caused a significant reduction in lipid peroxidation and hydrogen peroxide and mitigation of the drought induced oxidative stress. Drought stress induced protein content, total phenol, flavonol content, soluble sugar, palmitic acid and palmitoloic acid, and reduced flavonoid content, oleic acid, linolenic acid, and linolonoic acid in canola seed. PEN and Ca increased palmitic acid, linoleic acid (Omega-6), linolenic acid (Omega-3), oleic acid, protein, and soluble sugar. Our results indicated that application of PEN and Ca enables canola plants to withstand the deleterious impact of drought stress and caused improvement of antioxidant capacity, essential fatty acids (linolenic acid and linolonoic acid) and oil quality in canola seed.
KEYWORDS:
1. Introduction
Among the various abiotic stresses, drought stress is a more severe constraint that reduces the yield and quality of crop plants (Yamaguchi-Shinozaki et al. Citation2002; Hongbo et al. Citation2005). Reduce the product by drought has been reported in many crop species, which depends on the severity and duration of the stress period. There is an urgent need to improve the compatibility of crop species to drought stress to maintain high yield and quality (Kaczmarek et al. Citation2017).
Among the major food crops, Brassica crops are more susceptible to drought and salinity because they grow mainly in arid and semiarid areas. Canola (Brassica napus L.) is the third source of plant oils after Palm and soybean in the world that its seed contains about 40–44% oil (Carmody Citation2001). Remaining of canola after extraction of oil contains 38–44% high-quality protein that is used for animal nutrition (Walker and Booth Citation2007). Canola oil has potential for developing biodiesel market. Canola is relatively poorly adapted to drought stress and its yield is reduced (Wright et al. Citation1997). Seed yield reduced 19–39% as compared to control, when drought stress was imposed at reproductive stage (Gunasekara et al. Citation2006).
Penconazole (PEN) [1-(2,4-dichloro-β-propylphenethyl)-1 H-1,2,4-triazole] is a triazole compound. Triazoles are fungicides that have the characteristics of growth regulating and stress protecting (Jaleel et al. Citation2007a). Triazole compounds cause various morphological and biochemical responses in plants including stimulation of root growth, reduction in free-radical damage, and increase in antioxidant capacity (Jaleel et al. Citation2007a; Manivannan et al. Citation2007).
Calcium (Ca2+) is an essential element for plants and causes initiation of bud formation, polar growth, gas-exchange regulation, secretion, movements and light- and hormone-regulated growth and development (Hepler and Wayne Citation1985). Calcium mitigated the adverse effects of drought stress in plants (Jaleel et al. Citation2007b), and is involved in signaling responses against drought (Shao et al. Citation2008). Calcium has a central role in many defense mechanisms under drought, and Ca2+ signaling is required for drought tolerance or resistance (Cousson Citation2009).
There are many reports that show that application of PEN and Ca can play a major role in increasing plant tolerance against abiotic stresses (Merati et al. Citation2014; Upadhyaya et al. Citation2011). There are no reports regarding the effect of PEN and Ca on growth, physiological and biochemical parameters and oil fatty acid composition in canola. Thus, this work targets to investigate improvement effects of PEN and calcium on canola under drought stress. Moreover, deciphering the physiological biochemical mechanisms responsible for growth promotion and too, the effect of PEN and Ca on fatty acid composition, oil quality and different compounds in canola seed are other aims of the present study.
2. Materials and methods
2.1. Plant materials and growth parameters
Seeds of canola (B. napus L.) were obtained from the Seed and Plant Improvement Research Institute, Karaj, Iran. Seeds of canola were sown in each plastic pot containing an equal mixture of peat and perlite. After germination, the seedlings were thinned to five plants per pot. Plants were grown at average day/night temperatures of 25/18°C. The pots were irrigated with equal amount of half strength Hoagland solution for four week. Drought stress was imposed by PEG (5% (W/V) of PEG 6000) for three week. In the preliminary experiments, 5, 10, 15, and 20 mg l−1 PEN and 5, 10, 15, and 20 mM CaCl2 were used to determine the optimum concentrations. According to growth parameters and based on the studied PEN and CaCl2 concentrations, 15 mg l−1 of PEN and 15 mM of CaCl2 were selected as the optimum concentrations for further studies. To determine the effect of PEN and Ca, PEG-treated plants were supplemented with 15 mg l−1 of PEN or 15 mM of CaCl2. The treatments were as follows: (1) Control: without PEG, CaCl2 and PEN, (2) PEG, (3) PEG and PEN, (4) PEG and CaCl2. Aqueous calcium chloride solution and PEN was applied uniformly to the plants once a week for 3 weeks. Each treatment was carried out in triplicate. Three weeks after treatment, plants were collected for analyses in all the experiments. The plants were evaluated 3 weeks after drought stress in terms of fresh weight (FW) and dry weight (DW).
2.2. Protein and proline content
Protein content was measured according to Bradford (Citation1976), using bovine serum albumin (BSA) as standard. Proline content was determined following Bates et al. (Citation1973). Plant material (0.05 g) was homogenized with 5 ml of 3% sulfosalicylic acid and the homogenate was centrifuged at 13,249 × g for 20 min. The resulting solution (2 ml) was treated with 2 ml acid ninhydrin and 2 ml of concentrated glacial acetic acid, shaken vigorously, and boiled for 1 h at 100°C. The reaction mixture was extracted with 4 ml toluene, and the absorbance was measured at 520 nm by UV – visible spectrophotometer (Shimadzu UV-160, Tokyo, Japan). The content of proline was determined using L-proline as standard.
2.3. Hydrogen peroxide and malondialdehyde content
The content of hydrogen peroxide (H2O2) was estimated according to method of Velikova et al. (Citation2000). The plant material (0.5 g) was homogenized in an ice bath with 5 ml of 0.1%(w/v) trichloroacetic acid (TCA). The homogenate was centrifuged at 11,289 × g for 15 min and 0.5 ml of the supernatant was added to 0.5 ml of 10 mM potassium phosphate buffer (pH 7.0) and 1 ml of 1M of potassium iodide (KI). The absorbance of the supernatant was measured at 390 nm. The content of H2O2 was calculated by comparison with a standard curve made by using different concentrations of H2O2. The lipid peroxidation was determined based on malondialdehyde (MDA) content by Heath and Packer method (Citation1968). Plant material (0.5 g) was homogenized in 5 ml of 0.1% (w/v) trichloroacetic acid (TCA) and centrifuged at 7840 × g for 20 min. To 1 ml aliquot of the supernatant, 4 ml of 0.5% thiobarbituric acid (TBA) in 20% TCA was added. The mixture was heated at 95ºC for 30 min and quickly cooled in an ice bath. After centrifugation at 7840 × g for 15 min, the absorbance of the supernatant was recorded at 532 and 600 nm. The content of MDA was calculated using extinction coefficient of 155 mM−1cm−1.
2.4. Peroxidase, superoxide dismutase, and DPPH-radical scavenging activity
Peroxidase (POX) activity was measured according to the method of Abeles and Biles (Citation1991). The reaction mixture contained 2 ml of 0.2 M acetate buffer (pH 4.8), 0.2 ml H2O2 (3%), 0.1 ml 40 mM benzidine, and 0.1 ml enzyme extract. The increase in absorbance was recorded at 530 nm. Superoxide dismutase (SOD) activity was estimated by monitoring the inhibition of photochemical reduction of nitroblue tetrazolium (NBT) as described by Giannopolitis and Ries (Citation1977) in a reaction mixture containing 50 mM sodium phosphate buffer (pH 7.5), 13 mM methionine, 75 μM NBT, 75 μM riboflavin, 0.1 mM EDTA, and 100 μl of enzyme extract. The reaction mixture was irradiated for 16 min and absorbance was read at 560 nm against the nonirradiated blank. For estimation of DPPH (1, 1-diphenyl-2- picrylhydrazyl) radical scavenging activity used of Abe et al. (Citation1998) method. Leaf material (100 mg) was homogenized in 1 ml of 96% ethanol and then insoluble materials removed by centrifuge at 3500 × g for 5 min. 20 μl of extracting solution was mixed with 800 μl of DPPH (0.5 mM in ethanol). The absorbance of the resulting solution was measured at 517 nm after 30 min in darkness. Using the following equation the free radical scavenging activity was calculated:
2.5. Total phenol and flavonol content
For determination of total phenol content 0.1 g of seed was extracted with boiling 80% methanol for 3 h according to Conde et al. (Citation1995). Total phenol content was determined by using Folin-Ciocalteu reagent according to Akkol et al. (Citation2008). One milliliter of methanolic extract was mixed with 5 ml Folin-Ciocalteu reagent and 4 ml sodium carbonate solution 7.0%. The mixtures were allowed to stand for 2 h before its absorbance was measured at 765 nm. Gallic acid was used as a standard for the calibration curve. Flavonol content was determined according to Akkol et al. (Citation2008). One ml of methanolic extract, 3 ml of sodium acetate, and 1 ml of aluminum chloride solution were mixed and absorbance was measured at 445 nm after 2.5 h and rutin was used as a standard.
2.6. Flavonoid and anthocyanin content
Approximately 0.1 g of seed was homogenized in 3 ml of methanol. Flavonoid content was determined using aluminum chloride colorimetric method. Methanolic extract (0.5 ml) was mixed with 1.5 ml of pure methanol, 0.1 ml of 10% aluminum chloride, 0.1 ml of 1 M potassium acetate and 2.8 ml of distilled water and kept at room temperature for 30 min. The absorbance of the reaction mixture was measured at 415 nm and expressed in µg g−1 fresh weight (Chang et al. Citation2002). Anthocyanin content was determined in 0.3% HCl in methanol at 25°C using the extinction coefficient (33,000 cm2 mol−1) at 550 nm (Wagner Citation1979).
2.7. Tocopherol and soluble sugar content
Tocopherol content was estimated according to the method of Kayden et al. (Citation1973). Approximately 0.1 g of seed was homogenized in 3 ml of ethanol. One ml of the ethanol extract was pipetted in to a 4 ml glass test tube. The extract was mixed with 0.2 ml of 0.2% bathophenanthroline in ethanol and the content of each tube were homogenized. The assay proceeded rapidly from this point and care was taken not to expose the solutions to direct light. 0.2 ml of a 1 mM FeC13 solution in ethanol was added, followed by mixing with a vortex mixer. After 1 min, 0.2 ml of 1 mM H3PO4 solution in ethanol was added and the contents of the tubes were again mixed. The absorbance of the solutions was determined at 534 nm using α-tocopherol as standard. Soluble sugar content was determined based on the method of phenol sulfuric acid using glucose as a standard. The seed (0.1 g) was extracted using 3 ml deionize water by mortar and pestle. To determine soluble sugar content, 50 µl of extract was mixed with 450 µl of water and 500 µl of 5% phenol solution and 2.5 ml of concentrated sulfuric acid were added rapidly so that the solutions were well mixed and allowed to stand at room temperature for 30 min. The absorbance of the samples was measured at 485 nm (Dubois et al. Citation1956).
2.8. Fatty acid analyses
Approximately 0.1 g of seed was placed in test tubes. One milliliter of transesterification reagent (methanol/acetyl chloride, 20:1 v/v) was added to each tube. The tubes were heated at 100°C for 1 h for the transmethylation, being shaken every 10–15 min. The mixture was cooled to room temperature, and 1 ml each of water and hexane were added. The tubes were shaken and centrifuged. The upper one (hexane) was transferred to another tube (Rodriguez-Ruiz et al. Citation1998) and fatty acid analyzed by gas liquid chromatography. Calculations were also made for oleic desaturation ratio (ODR), linoleic desaturation ratio (LDR), and oleic/linoleic acid ratio (OLR) (Pleines and Friedt Citation1988).
2.9. Statistical analysis
Each experiment was repeated three times and the data were analyzed by using one -way analysis of variance (ANOVA) using SPSS (version 21) and means were compared by Duncan’s test at the 0.05 level of confidence. PCA (principal component analysis) and HCA (Hierarchical cluster analysis) were used for evaluating correlation between each pair of variables and performed using XLSTAT (2016) and online CIMminner software, respectively.
3. Results
Growth parameters (FW and DW) significantly decreased under drought (). FW and DW decreased 36.44% and 36.59% as compared to control, respectively. PEN and Ca treatment increased FW and DW in canola under drought stress. PEN and Ca caused an increase of 39.67% and 39.43% in DW, respectively.
Figure 1. Fresh weight (A) and Dry weight (B) in canola plant and protein content (C) and soluble sugar content (D) in canola seed under drought, PEN, and Ca treatment. Vertical bars indicate Means ± SE based on three replicates. Different letters above columns indicated significant (P < 0.05) differences.
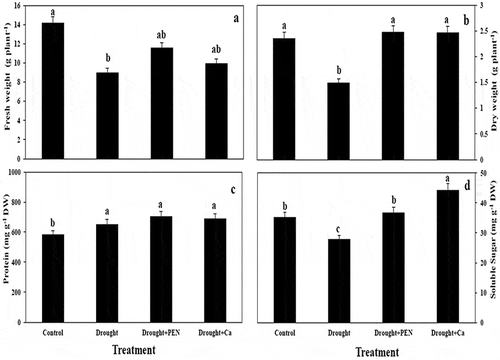
Drought caused no significant change in protein content, but PEN and Ca treatment increased it. Inducing effect of Ca on protein content was more prominent than PEN. Drought stress increased (49.25%) in proline content as compared to control. PEN and Ca treatment caused more induction proline content under drought. Drought significantly increased H2O2 content. By contrast, the application of PEN and Ca decreased this parameter by 29.9% and 60.69%, respectively, compared to drought-stressed plants. MDA content was enhanced by drought stress, but PEN treatment decreased it, compared to drought-stressed plants. Drought and Ca did not induce any changes in POX activity as compared to control. PEN treatment increased POX activity in canola under drought. Drought increased SOD activity, but PEN and Ca treatment decreased it. DPPH radical scavenging activity decreased under drought stress. PEN and Ca treatment induced DPPH radical scavenging activity, but this induction was higher by Ca ().
Table 1. Effects of PEN and Ca on different parameters in canola leaf under drought.
Drought, PEN and Ca treatment increased protein content in canola seed. In contrast to drought, PEN and Ca treatment increased soluble sugars content in canola seed and too, increasing effect of Ca was higher (,).
Total phenol content was enhanced in seed by drought. Ca treatment increased total phenol content in seed but PEN treatment decreased it under drought stress. Drought stress significantly increased (44.40%) favonol content in canola seed, but application of PEN and Ca reduced this parameter. Drought and Drought in combination to PEN caused a significant reduce in flavonoid content in canola seed, but Ca treatment induced it. Anthocyanin content in canola seed was showed any significant change by drought and PEN. Application of Ca decreased anthocyanin content in canola seed ().
Figure 2. The effect of drought stress, PEN, and Ca on total phenol (A), flavonol (B), flavonoid (C), and anthocyanin (D) in canola seed. Vertical bars indicate mean ± SE of three replicates. Different letters indicate significant differences at P < 0.05.
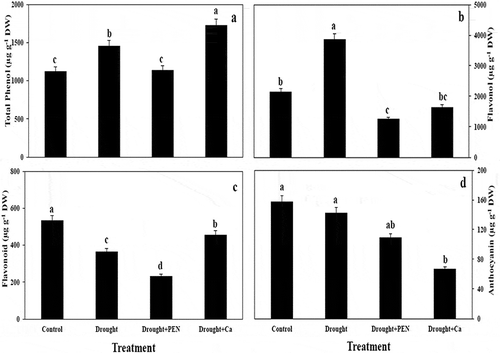
The application of Ca increased tocopherol content in canola seed by 68.47% compared to control. All treatment (drought, PEN, and Ca) caused non-significant change in DPPH radical scavenging activity in canola seed (,).
Figure 3. The effect of PEN, Ca, and PEG on tocopherol content (A), inhibition of DPPH radical (B), palmitic acid (C), and palmitoloic acid (D) in canola seed. Vertical bars indicate mean ± SE of three replicates. Different letters indicate significant differences at P < 0.05.
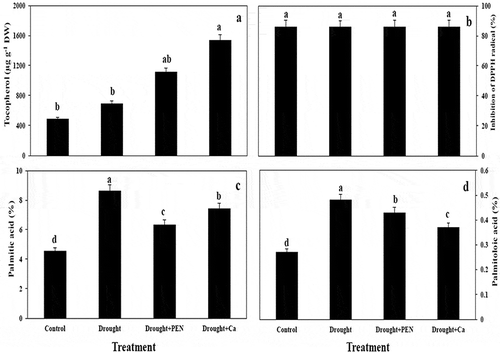
Drought, PEN, and Ca treatment enhanced palmitic acid (C16) and palmitoloic acid (C16:1) in canola seed as compared to control. Stearic acid (C18) in seed was not showed a significant change under all treatment. In contrast to drought, PEN and Ca treatment increased oleic acid (C18:1). Drought stress reduced linoleic acid (C18:2) in canola seed, but exogenous application of PEN and Ca increased it as compared to control. PEN and Ca caused a significant induce linolenic acid (C18:3) in canola seed as compared to stressed- plants. PEN and Ca treatment increased ODR and LDR in canola seed. Drought in combination to Ca decreased OLR (,).
Figure 4. Effect of PEN and Ca on stearic acid (A), oleic acid (B), linoleic acid (C), and linolenic acid (D) in canola seed under drought. Vertical bars indicate mean ± SE of three replicates. Different letters above columns indicated significant (P < 0.05) differences.
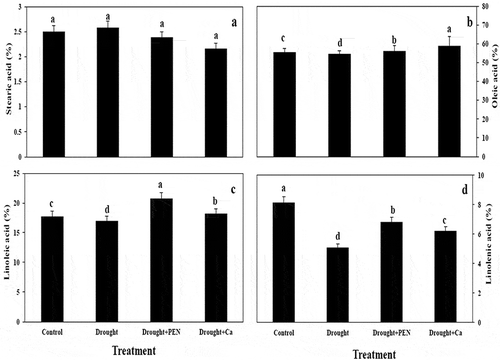
Figure 5. Impact of PEG, PEN, and Ca on ODR (A), LDR (B), and OLR (C) in canola seed. Columns indicate mean ± SE based on three replicates. Means with different letters for each cultivar indicate a significant difference at P < 0.05 using Duncan multiple range test.
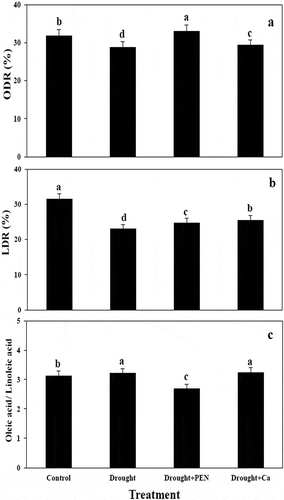
3.1. PCA analyses
According to correlation analyses based on Pearson’s coefficient in canola, DW exhibited positive correlations with POX, DPPH (Leaf), protein, and proline, but it showed a negative correlation with H2O2 and MDA. H2O2 and MDA displayed positive correlations to each other. H2O2 exhibited negative correlation with protein and DPPH (Leaf). MDA showed a negative correlation with POX and SOD. DW and FW showed a positive correlation with linoleic acid, linolenic acid, ODR, LDR, DPPH (Seed), and soluble sugar, but they showed a negative correlation with palmitic acid, palmitoloic acid, OLR, total phenol, and flavonol. Palmitic acid and palmitoloic acid displayed positive correlations to each other, but they showed a negative correlation with linolenic acid, ODR and LDR. Positive correlations were obtained between protein, soluble sugar, total phenol, tocopherol, and oleic acid in canola seed ().
4. Discussion
Water shortage is an environmental limitation for the production of plant. Reduction of yield by drought is more than reducing other factors. Growth occurs through cell division, cell enlargement and differentiation, and includes genetic, physiological, ecological and morphological events and their complex interactions. The quality and quantity of plant growth depend on these events, which are affected by water shortage. Cell growth is one of the most drought-sensitive physiological processes due to the reduction in turgor pressure (Taiz and Zeiger Citation2006). Three main mechanisms decline yield by drought stress: (i) reduced canopy absorption of photosynthetic active radiation, (ii) reduce the efficiency of radiation use, and (iii) decreased harvest index (Earl and Davis Citation2003).
The present investigation effectively confirmed that PEN and Ca could induce the drought tolerance in canola. Triazoles by change in hormonal statue cause increase dry weight under drought stress (Grossman Citation1990). Triazole enhanced the whole plant dry weight in rose (Jenks et al. Citation2001). Some studies have shown that CaCl2 can play an important role to ameliorate the negative effects of drought stress on several species. These responses could be attributed to that Ca is a crucial secondary messenger in the signaling processes to many defense mechanisms that are induced by drought stress (Xiang et al. Citation2008; Shao et al. Citation2008). Increasing plant growth as a response to CaCl2 occurs as a result of cell elongation and cell division (Tanimoto Citation1990; Hirschi Citation2004).
Plants accumulate different types of organic and inorganic solutes in the cytosol to lower osmotic potential and maintain cell turgor (Rhodes and Samaras Citation1994). Protein accumulation is used as osmotic regulation which increases plant’s tolerance to adverse effects of abiotic stresses (Ma and Turner Citation2006). The accumulation of proline regulates protein turnover and stress protective proteins and too, is involved in ROS scavenging (Ahmad et al. Citation2015). Proline can act as a signaling molecule to modulate mitochondrial functions, influence cell proliferation or cell death, and trigger specific gene expression (Szabados and Savoure Citation2009). Accumulation of protein and proline by PEN and Ca indicates the positive role of PEN and Ca in mitigating the negative impact of drought stress in canola. Triazole induces a temporary increase in ABA content. Increase of ABA content can be due to increased proline and amino acid content in triazole-treated carrot plants (Gopi et al. Citation2007). Paclobutrazol increased the proline content in Eruca sativa seedlings (Mathur and Bohra Citation1992). Calcium increased proline by breakdown of proline-rich protein and synthesis of proline and amino acids (Jun et al. Citation2000).
The various types of stresses (salt stress, drought, temperature, heavy metals, pollution, high irradiance, pathogen in fection, etc.) disturbed in balance between ROS generation and ROS scavenging. ROS react with unsaturated fatty acids and cause peroxidation of membrane lipids, leakage of cellular contents, rapid desiccation, and cell death. The ability of plants to induce enzymatic defense against uncontrolled lipid peroxidation is an important trait of the tolerance mechanisms (Jaleel et al. Citation2007a; Velikova et al. Citation2000). The scavenging of ROS is required to maintain metabolic functions under stress. ROS scavenging depends on detoxification mechanism provided by enzymatic and non-enzymatic antioxidants. SOD belongs to the family of metalloenzymes in all aerobic organisms and is the first line of defense against ROS-induced damages under abiotic stresses. The SOD catalyzes the removal of O2•− by dismutating it in to O2 and H2O2 and POX scavenge H2O2 in to H2O (Mittler Citation2002). PEN and Ca application caused an increase in DPPH-radical scavenging activity and decreased MDA and H2O2 content which suggested a protective role of PEN and Ca in mitigating oxidative stress in canola. Triazoles induced the antioxidant capacity in oxidative stressed plants as compared to control (Sankar et al. Citation2007). Increase in antioxidants cause scavenging of harmful free radicals, inhibition of electrolyte leakage and lipid peroxidation that correlated with ability of triazoles in maintaining the membrane integrity (Fletcher et al. Citation2000). This stress-ameliorating role of calcium can be attributed to the enhancement of antioxidant activity, which was shown to decrease lipid peroxidation in calcium-treated plants (Asgher et al. Citation2014).
Canola has the most phenolic compounds (about tenfold) in comparison to the other oilseeds (soybean, sunflower) (Naczk et al. Citation1998). Canola phenolic compounds are including sinapinic acid and other derivatives which have high antioxidant activity (Szydłowska-Czerniak et al. Citation2011). The main unsaponifiable components in canola oil are tocopherols and phytosterols. Tocopherols (vitamin E) are known to be very efficient natural antioxidants (Yanishlieva-Maslarova et al. Citation2001). Moreover, antioxidant compounds in canola including polyphenols, sterols, flavonoids, tocopherols etc. have an important role in prevent of some chronic diseases, such as heart, neurodegenerative, aging, cancer, and rheumatoid arthritis (Amarowicz et al. Citation2003; Kozlowska et al. Citation1983; Siger et al. Citation2004). Canola oil consists of four important fatty acids: palmitic acid, linoleic acid, linolenic acid, and oleic acid. Linolenic acid in canola oil is more than the other plant oils. Canola oil is high in oleic acid which is commonly used for food and industrial purposes and this edible oil is very healthy (Zhang et al. Citation2004). Canola is a major oil crop and also supplies proteins for the feed. Proteins showed the major category of storage compounds and their amino acid composition was found to be very close to that of canola in different conditions. In fact, oil-free canola meal contains 38–40% of crude proteins that exhibit an amino acid composition with high levels of essential sulfur containing amino acids (Nesi et al. Citation2008). Mingeau (Citation1974) showed that adverse effects of drought stress on oil biosynthesis were more than on protein biosynthesis. Jensen et al. (Citation1996) observed that, drought stress had opposing effects on oil and protein content of B. napus seeds at vegetative stage of growth. Bouchereau et al. (Citation1996) showed that drought stress, during the flowering stage, affected the oil content and fatty acid composition of canola seeds. Mekki et al. (Citation1999) also showed that drought stress increased the percentage of palmitic acid and reduced unsaturated fatty acids. Pham-Thi et al. (Citation1986) reported that water stress decreased the degree of fatty acids unsaturation which was attributed to the inhibition in the biosynthesis of polyunsaturated fatty acids and suppression in the activities of desaturases. Triazole compounds caused an increase in oil content in Helianthus annuus L. (Sunflower) under drought stress (Rabert et al. Citation2015). Exogenous of calcium mitigated the adverse effects of NaCl stress in pea plants and increased the yield components (Howladar and Rady Citation2012). Ca treatment increased total phenol, tocopherol and flavonoid in canola seed. PEN and Ca enhanced palmitic acid, linoleic acid (Omega-6), linolenic acid (Omega-3), oleic acid, protein, and soluble sugar. Overall, PEN and Ca caused an increase in antioxidant capacity, nutritional value, and improvement of quality in canola seed.
In conclusion, PEN and Ca helped to resistance of canola to drought stress and increased plant growth. Such positive effects of PEN and Ca can be due to their impact on improvement of antioxidant defense systems, accumulation of protein and proline and declined in H2O2 and MDA content. Thus, PEN and Ca caused improvement of growth and increase antioxidant capacity, nutritional value, and quality in canola seed.
Author contribution
Maryam Rezayian has contributed in the major bench experiments. Dr. Vahid Niknam and Dr. Hassan Ebrahimzadeh equally designed the experiments. All authors read and approved the manuscript.
Additional information
Funding
References
- Abe N, Murata T, Hirota A 1998: Novel 1,1- diphenyl-2-picryhy- drazyl- radical scavengers, bisorbicillin and demethyltrichodimerol, from a fungus. Biosci. Biotechnol. And Biochem., 62, 61–662.
- Abeles FB, Biles CL 1991: Characterization of peroxidases in lignifying peach fruit endocarp. Plant Physiol., 95, 269–273.
- Ahmad P, Sarwat M, Bhat NA, Wani MR, Kazi AG, Tran LS, Zhang JS 2015: Alleviation of cadmium toxicity in Brassica juncea L. (Czern. &Coss.) by calcium application involves various physiological and biochemical strategies. PLOS One, 10, e0114571. doi:10.1371/journal.pone.0114571
- Akkol EK, Goger F, Koşar M, Başer KHC 2008: Phenolic composition and biological activities of Salvia halophila and Salvia virgata from Turkey. Food Chem., 108, 942–949. doi:10.1016/j.foodchem.2007.11.071
- Amarowicz R, Naczk M, Shahidi F 2003: Antioxidant activity of crude tannins of canola and rapeseed hulls. J. Am. Oil Chem. Soc., 77, 957–961. doi:10.1007/s11746-000-0151-0
- Asgher M, Khan NA, Khan MIR, Fatma M, Masood A 2014: Ethylene production is associated with alleviation of cadmium-induced oxidative stress by sulfur in mustard types differing in ethylene sensitivity. Ecotox. Environ. Saf., 106, 54–61. doi:10.1016/j.ecoenv.2014.04.017
- Bates LS, Waldren RP, Teare ID 1973: Rapid determination of free proline for water-stress studies. J. Plant Soil, 39, 205–207. doi:10.1007/BF00018060
- Bouchereau A, Bensaoud A, Clossais-Besnard N, Renard M 1996: Water stress effects on rapeseed quality. Europ. J. Agron., 5, 19–30. doi:10.1016/S1161-0301(96)02005-9
- Bradford MM 1976: A rapid and sensitive method for the quantitation of microgram quantities of protein utilizing the principle of protein-dye binding. Anal. Biochem., 72, 248–254. doi:10.1016/0003-2697(76)90527-3
- Carmody O 2001: Why grow canola in the central grain belt. In Bulliten, Vol. 4492. Agricultural Western Australia, South Perth.
- Chang C, Yang M, Wen H, Chern J 2002: Estimation of total flavonoid content in propolis by two complementary colorimetric methods. J. Food Drug Anal., 10, 178–182.
- Conde E, Cadahia E, Garcia-Vallejo M 1995: HPLC analysis of flavonoids and phenolic acids and aldehydes in eucalyptus spp. Chromatographia, 41, 657–660. doi:10.1007/BF02688103
- Cousson A 2009: Involvement of phospholipase C-independent calcium-mediated abscisic acid signaling during Arabidopsis response to drought. Biol. Plant., 53, 53–62. doi:10.1007/s10535-009-0008-0
- Dubois M, Gilles KA, Hamilton JK, Rebers PA, Smith F 1956: Colorimetric method for determination of sugars and related substances. Annal. Chem., 28, 350–356. doi:10.1021/ac60111a017
- Earl H, Davis RF 2003: Effect of drought stress on leaf and whole canopy radiation use efficiency and yield of maize. Agron. J., 95, 688–696. doi:10.2134/agronj2003.0688
- Fletcher RA, Gilley A, Davis TD, Sankhla N 2000: Triazoles as plant growth regulators and stress protectants. Hort. Rev., 24, 55–138.
- Giannopolitis CN, Ries SK 1977: Superoxide dismutases: II. Purification and quantitative relationship with water soluble protein in seedlings. J. Plant Physiol., 59, 315–318. doi:10.1104/pp.59.2.315
- Gopi R, Abduljaleel CA, Sairam R, Lakshmanan GMA, Gomathinayagam M, Panneerselvam R 2007: Differential effects of hexaconazole and paclobutrazol on biomass, electrolyte leakage, lipid peroxidation and antioxidant potential of Daucus carota L. Colloids Surf. B. Biointerfaces, 60, 180–186. doi:10.1016/j.colsurfb.2007.06.003
- Grossman K 1990: Plant growth retardants as tools in physiological research. Physiol. Plant, 78, 640–648. doi:10.1111/ppl.1990.78.issue-4
- Gunasekara CP, Martin LD, French RJ, Siddique KHM, Walton G 2006: Genotype by environment interactions of Indian mustard (Brassica juncea L.) and canola (Brassica napus L.) in Mediterranean-type environments: I. Crop growth and seed yield. Eur. J. Agron., 25, 1–12. doi:10.1016/j.eja.2005.08.002
- Heath RL, Packer L 1968: Photoperoxidation in isolated chloroplasts: I. Kinetics and stoichiometry of fatty acid peroxidation. Arch. Biochem. Biophys., 125, 189–198.
- Hepler PK, Wayne RO 1985: Calcium and plant development. Annu. Rev. Plant Physiol., 36, 397–439. doi:10.1146/annurev.pp.36.060185.002145
- Hirschi KD 2004: The calcium conundrum. Both versatile nutrient and specific signal. Plant Physiol., 136, 2438–2442. doi:10.1104/pp.104.046490
- Hongbo S, Zongsuo L, Mingan S 2005: Changes of antioxidative enzymes and MDA content under soil water deficits among 10 wheat (Triticum aestivum L.) genotypes at maturation stage. Colloids Surf. B. Biointerfaces, 45, 7–13. doi:10.1016/j.colsurfb.2005.06.016
- Howladar SM, Rady MM 2012: Effects of calcium paste as a seed coat on growth, yield and enzymatic activities in NaCl stressed-pea plants. Afr. J. Biotechnol., 11, 14140–14145. doi:10.5897/AJB12.2190
- Jaleel CA, Gopi R, Panneerselvam R 2007a: Alterations in lipid peroxidation, electrolyte leakage, and proline metabolism in Catharanthus roseus under treatment with triadimefon, a systemic fungicide. Comptes Rendus Biol., 330, 905–912. doi:10.1016/j.crvi.2007.10.001
- Jaleel CA, Manivannan P, Sankar B, Kishorekumar A, Gopi R 2007b: Water deficit stress mitigation by calcium chloride in Catharanthus roseus: effects on oxidative stress, proline metabolism and indole alkaloid accumulation. Colloids Surf. B. Biointerfaces, 60, 110–116. doi:10.1016/j.colsurfb.2007.06.006
- Jenks MA, Andersen L, Teusink RS, Williams MH 2001: Leaf cuticular waxes of potted rose cultivars as affected by plant development, drought and paclobutrazol treatments. Physiol. Plant., 112, 62–70.
- Jensen CR, Mogensen VO, Mortensen G, Fieldsend JK 1996: Seed glucosinolate, oil and protein contents of field-grown rape (Brassica napus L.) affected by soil drying and evaporative demand. Field Crops Res., 47, 93–105. doi:10.1016/0378-4290(96)00026-3
- Jun HR, Adam LH, Rozwadowski KL, Hammerlineli JL, Keller WA, Selvaraj G 2000: Genetic engineering of glycine betain production towards enhancing stress tolerance in plants. Plant Physiol., 12, 747–756.
- Kaczmarek M, Fedorowicz-Stronska O, Głowacka K, Waskiewicz A, Sadowski J 2017: CaCl2 treatment improves drought stress tolerance in barley (Hordeum vulgare L.). Acta Physiol. Plant., 39, 41–52. doi:10.1007/s11738-016-2336-y
- Kayden HJ, Chow CK, Bjornson LK 1973: Spectrophotometric method for determination of tocopherol in red blood cells. J. Lipid Res., 14, 533–540.
- Kozlowska H, Rotkiewicz DA, Zadernowski R 1983: Phenolic acids in rapeseed and mustard. J. Am. Oil Chem. Soc., 60, 1119–1123. doi:10.1007/BF02671339
- Ma Q, Turner DW 2006: Osmotic adjustment segregates with and is positively related to seed yield in F3 lines of crosses between Brassica napus and B. juncea subjected to water deficit. Aust. J. Exp. Agric., 46, 1621–1627. doi:10.1071/EA05247
- Manivannan P, Jaleel CA, Kishorekumar A, Sankar B, Somasundaram R, Sridharan R, Panneerselvam R 2007: Changes in antioxidant metabolism of Vigna unguiculata (L.) Walp. By propiconazole under water deficit stress. Colloids Surf B Biointerfaces, 57, 69–74. doi:10.1016/j.colsurfb.2007.01.004
- Mathur R, Bohra SP 1992: Effect of paclobutrazol on aminotransferases; Protein and proline content in Eruca sativa var. T-23 seedlings. J. Phytol. Res., 5, 93–95.
- Mekki BB, EL-kholy MA, Mohamad EM 1999: Yield, oil and fatty acids contents as affected by water deficit and potassium fertilization in two sunflower cultivars. Egypt. J. Agron., 21, 67–85.
- Merati MJ, Hassanpour H, Niknam V, Mirmasoumi M 2014: Exogenous application of penconazole regulates plant growth and antioxidative responses in salt stressed Mentha pulegium L. J. Plant Interact., 9, 791–801. doi:10.1080/17429145.2014.948084
- Mingeau M 1974: Comportement du colza de printemps a la secheresse. Inf. Cetiom, 36, l–11.
- Mittler R 2002: Oxidative stress, antioxidants and stress tolerance. Trends Plant Sci., 7, 405–410.
- Naczk M, Amarowicz R, Sulivan A, Shahidi F 1998: Current research developments on polyphenolics of rapeseed/canola: a review. Food Chem., 62, 489–502. doi:10.1016/S0308-8146(97)00198-2
- Nesi N, Delourme R, Brégeon M, Falentin C, Renard M 2008: Genetic and molecular approaches to improve nutritional value of Brassica napus L. seed. C. R. Biol., 331, 763–771. doi:10.1016/j.crvi.2008.07.018
- Pham-Thi AT, Borrel-Flood C, Vieira Da Sila J, Justin AM, Mazliak P 1986: Effects of water stress on lipid metabolism in cotton leaves. Photochem., 24, 723–727.
- Pleines S, Friedt W 1988: Breeding for improved C18-fatty acid composition in rapeseed (Brassica napus L.). Fat Sci. Technol., 5, 167–171.
- Rabert GA, Rajasekar M, Manivannan P 2015: Triazole-induced drought stress amelioration on growth, yield, and pigments composition of Helianthus annuus L. (sunflower). Int. Multidiscipline Res. J., 5, 6–15.
- Rhodes D, Samaras Y 1994: Genetic Control of Osmoregulation in Plants. In Cellular and Molecular Physiology of Cell Volume Regulation, pp. 347–361. CRC Press, Boca Raton.
- Rodriguez-Ruiz J, Belarbi EL-H, Garcia Sanchez GL, Alonso DL 1998: Rapid simultaneous lipid extraction and transesterification for fatty acid analyses. Biotechnol. Tech., 12, 689–691. doi:10.1023/A:1008812904017
- Sankar B, Jaleel CA, Manivannan P, Kishorekumar A, Somasundaram R, Panneerselvam R 2007: Effect of paclobutrazol on water stress amelioration through antioxidants and free radical scavenging enzymes in Arachis hypogaea L. Colloids Surf B Biointerfaces, 60, 229–235. doi:10.1016/j.colsurfb.2007.06.016
- Shao H, Song W, Chu L 2008: Advances of calcium signals involved in plant anti-drought. C. R. Biol., 331, 587–596. doi:10.1016/j.crvi.2008.03.012
- Siger A, Nogala-Kazucka M, Lampart-Szczapa E, Hoffmann A 2004: Phenolic compound contents in new rape varieties. Rosliny Oleiste, 1, 263–274.
- Szabados L, Savoure A 2009: Proline: a multifunctional amino acid. Trends Plant Sci., 15, 89–97.
- Szydłowska-Czerniak A, Bartkowiak-Broda I, Karlovic I, Karlovits G, Szłyk E 2011: Antioxidant capacity, total phenolics, glucosinolates and colour parameters of rapeseed cultivars. Food Chem., 127, 556–563.
- Taiz L, Zeiger E 2006: Plant Physiology, 4th ed., Sinauer Associates Inc. Publishers, Massachusetts.
- Tanimoto E 1990: Gibberellin requirement for the normal growth of roots. In Gibberellins, Takahashi N, Phinney BO, MacMillan J Eds., pp. 229–240. Springer, New York.
- Upadhyaya H, Panda SK, Dutta BK 2011: CaCl2 improves post-drought recovery potential in Camellia sinensis (L) O, Kuntze. Plant Cell Rep., 30, 495–503.
- Velikova V, Yordanov I, Edreva A 2000: Oxidative stress and some antioxidant system in acid-treated bean plants: protective role of exogenous polyamines. Plant Sci., 151, 59–66.
- Wagner GJ 1979: Content and vacuole/extra vacuole distribution of neutral sugars, free amino acids and anthocyanins in protoplasts. Plant Physiol., 64, 88–93.
- Walker KC, Booth EJ 2007: Agricultural aspects of rape and other Brassica products. Eur. J. Lipid Sci. Technol., 103, 441–446.
- Wright PR, Morgan JM, Jessop RS 1997: Drought stressed mustard yields more than canola due to greater leaf turgor. Ann. Bot., 80, 313–319.
- Xiang J, Chen Z, Wang P, Yu L, Li M 2008: Effect of CaCl2 treatment on the changing of drought related physiological and biochemical indexes of Brassica napus. Front. Agric. China, 2, 423–427.
- Yamaguchi-Shinozaki K, Kasuga M, Liu Q, Nakashima K, Sakuma Y, Abe H, Shinwary ZK, Seki M, Shinozaki K 2002: Biological mechanisms of drought stress response. Jircas Working Report, 23, 1–8.
- Yanishlieva-Maslarova NV, Pokorny J, Yanishlieva N, Gordon M 2001: Antioxidants in Food: practical Applications, pp. 22–70. CRC Press, Cambridge, UK.
- Zhang H, Shi C, Wu J, Rena Y, Li C, Zhang D, Zhang Y 2004: Analysis of genetic and genotype x environment interaction effects from embryo, cytoplasm and maternal plant for oleic acid content of Brassica napus L. Plant Sci., 167, 43–48.