ABSTRACT
In order to obtain high tomato production, chemical fertilizers have been intensively applied in solar greenhouse in Northern China. This unreasonable use of chemical fertilizers always leads to soil degradation. One of the most serious problems is the loss of exchangeable base cations: calcium (Ca), magnesium (Mg), and potassium (K), and available micronutrients: iron (Fe), manganese (Mn), copper (Cu), and zinc (Zn). We collected soils with different tomato cultivation durations: 0, 5, and 20 years, to investigate the effects of different fertilizers on the dynamics of soil base cations and micronutrients which extracted by 1 M ammonium acetate and 0.005 M diethylenetriaminepentaacetic acid, respectively. Five treatments were included: no fertilizer application as control (Con), chemical nitrogen, phosphorus and potassium fertilizers (CF), rice straw (FS), chicken manure (CM), and vermicompost (VM). Our results show that soil exchangeable Ca significantly decreased with the increase of cultivation duration, whereas soil exchangeable K increased due to the fertilization of K. Soil exchangeable Ca and K exhibited decreasing trends during the growth season of tomato, but not exchangeable Mg. Long-term tomato cultivation significantly increased soil available micronutrients. Soil available Fe and Mn fluctuated sharply during the growing season of tomato plant. Except Zn, fertilizers exhibited no constant significant effect on base cations and micronutrients. After long term planting of tomato, the loss of exchangeable Ca, but not Mg and micronutrients, might potentially constrain the productivity of crop under intensive chemical fertilization.
1. Introduction
Solar greenhouse cultivation developed rapidly in the last decade for its high land-use efficiency, short production cycle, and nice incomes in northeast China. However, in order to get maximal yield of tomato, chemical fertilizers were intensively used in solar greenhouses. For example, the application of chemical N is at the rate of > 1200 kg N ha−1 (Guo et al. Citation2010). These high chemical fertilizer inputs give rise to soil acidification, macronutrient (N, P, and K) enrichment and imbalance (Han et al. Citation2015). Soil degenerated faster under this condition than open-field agricultural systems (Ju et al. Citation2006). At the same time, the yields of tomato decreased even if higher chemical fertilizers were applied (Fu et al. Citation2017).
Base cations and micronutrients play essential roles in the sustainability of agriculture (Alloway Citation2008). The decrease of tomato yield may resulted from lack of base cations (i.e., exchangeable Ca, Mg, and K) and micronutrients (i.e., Fe, Mn, Cu, and Zn) in solar greenhouse. Unlike enrichment of macronutrients (N and P), deficiencies of base cations and micronutrients have been frequently observed in agricultural soil (Kopittke et al. Citation2017). A meta-analysis including 107 independent studies indicated that chemical N additions induced 24% loss of base cations in terrestrial ecosystems over time periods less than five years (Lucas et al. Citation2016). Short-term (1–2 year) and mid-term (4–5 year) chemical N addition mobilized base cations into solution by exchanging with H+, and subsequent leaching down the soil profile (Cusack et al. Citation2016). Intensively cultivated soils always exhibit deficiencies of micronutrients, which resulted from removing of crop production (Bitondo et al. Citation2013), meanwhile lower micronutrient inputs from chemical fertilizers (Fageria et al. Citation2003).
Organic fertilizers, such as manure and crop residue, have gained increased attention in improvement of soil fertility and soil structure (Yang et al. Citation2013; Peng et al. Citation2016). Because of their balanced nutrition content and enhancing soil retention ability of nutrients (Wang et al. Citation2017a), application of organic fertilizers has been shown to increase the availability of base cations and micronutrients in soil (Wei et al. Citation2006; Zingore et al. Citation2008; Cai et al. Citation2015). However, most of the manure contains high level of micronutrients, especially Cu and Zn, and applications of animal manures or manure composts can give rise to excessive accumulation of those metals in soil, leading to adverse effects on soil quality (Hang et al. Citation2009; Yang et al. Citation2017). Vermicompost is the cast of earthworm by feeding with organic materials, such as animal manure and rice straw. Previous studies indicated that heavy metal (including Cu) concentrations were declined significantly in vermicompost compared to original manure (Wang et al. Citation2017; Zhu et al. Citation2018). As a natural organic fertilizer, vermicompost has been widely used in organic farm for the past few years (Lim et al. Citation2015). However, whether vermicompost is superior to conventional organic fertilizer in improving soil base cation and micronutrient availabilities should be studied.
Availabilities of base cations and micronutrients in soil are affected by several soil attributes, such as pH, SOM, redox potential, cation exchangeable capacity (CEC), moisture, microbial activity, and crop assimilate capacity (Perakis et al. Citation2013; Dotaniya and Meena Citation2015; Gruba and Mulder Citation2015; Wang et al. Citation2017b). Thus, the availabilities of base cations and micronutrients are codetermined by soil properties and crop growth stage. Soil microbial biomass and root assimilate ability differ sharply during growth stage of plant (Li et al. Citation2016; Wu et al. Citation2018). Even SOM, the relatively stable fraction in soil, has been showed seasonally transient (Turner et al. Citation2015). Thus, seasonal patterns in base cations and micronutrients availability are expected to occur during the plant growth period. However, most of the previous studies focused on long-term or annual change of base cations and micronutrients, seasonal change have been rarely explored.
In this study, we invested the effects of organic and inorganic fertilizers on availabilities of soil base cations and micronutrients during different growth stages of tomato in soils with different cultivation durations. We hypothesized that (1) long-term cultivation of tomato will cause decrease of base cations and micronutrients in solar greenhouse due to low input and plant uptake, (2) addition of organic fertilizers may increase availabilities of base cations and micronutrients due to improvement of soil fertility, and (3) availabilities of base cations and micronutrients will decrease because of uptake of tomato during tomato growth.
2. Materials and methods
2.1. Study sites and experimental design
Three soils that were classified as meadow brown earth (Mollic Gleysols, FAO-UNESCO system) were collected from Guanghui township of Yuhong District, Shenyang City, Liaoning Province. These soils were cultivated with tomato for different years: 0 year (collected from a field adjacent to the tomato-planting greenhouses), 5 years (in a solar greenhouse), and 20 years (in a solar greenhouse). Field managements were similar in 5-year and 20-year solar greenhouse, and both fertilized with chemical fertilizers. The basic properties of these soils are shown in . The collected soils were transferred to polyethylene plastic pots with a diameter of 30 cm and a height of 28 cm. Each pot was filled with 15 kg of air-dried soil which had yet been mixed uniformly with fertilizers. Five treatments for each soil were included: no fertilization as control (Con), chemical fertilizer (CF), rice straw (FS), chicken manure (CM), and vermicompost (VM). Concentrations of added chemical fertilizers under CF treatment were as follows: 0.40 g N, 0.11 g P, and 0.33 g K kg−1 soil as urea, superphosphate, and potassium sulfate, respectively. The added N, P, and K were equivalent to rates of 900 kg N, 250 kg P, and 747 kg K per hectare in field area. Applied rates of rice straw, chicken manure, and vermicompost were 40.8 g, 8.3 g, and 13.1 g kg−1 soil (about 30 t vermicompost per hectare), respectively. Extra chemical N, P, or K were added to FS, CM, and VM treatments to ensure that the concentrations of added N, P, and K were equal with CF treatment. All the fertilizers were applied as base fertilizer in a one-time application. Chemical properties of all the fertilizers are shown in . The tested tomato variety, ‘Gold Crown No. 9’, was grown until all the fruits were harvested. All the treatments were replicated four times, and a randomized block design was applied. The pot experiment was conducted from March to June in 2016 in the greenhouse (123°57ʹ E, 41°83ʹ N) of Shenyang Agricultural University in Shenyang, Liaoning Province. The greenhouse temperature ranged from 15 to 35°C. Natural light was supplied with no supplementary light.
Table 1. Chemical characteristics of the original soils. The parameters include pH, contents of total N (TN), total P (TP), available base cations (ACa, AMg, and AK), and available micronutrients (AFe, AMn, ACu, and AZn).
Table 2. Chemical characteristics of the fertilizers. The parameters include pH, contents of total N (TN), total P (TP), total base cations (TCa, TMg, and TK), and total micronutrients (TFe, TMn, TCu, and TZn).
2.2. Soil sampling and chemical analysis
Soil samples were collected from each pot on March 27 (flowering period, FP), April 26 (fruit set period, FSP), May 26 (early harvest period, EHP), and June 25 (late harvest period, LHP) of 2016 during tomato growth. Soils were passed through a 2-mm sieve and air-dried for the determination of chemical properties.
The soil pH (soil: water (w/v), 1:5) was measured using a Thunder Magnetic SJ-3F pH Meter (INESA, Shanghai, China). Soil available base cations were extracted with ammonium acetate according to the method described by Ochoa-Hueso et al. (Citation2014). Briefly, 5.0 g soil was mixed with 1 M ammonium acetate (pH 7.0) in a ratio of 1:10 (w/v). The mixture was shaken at 150 rpm for 30 min and then filtered. The concentrations of base cations in the filtration were analyzed by atomic absorption spectrometry (AAS, Shimadzu, Japan). Soil available Fe, Mn, Cu, and Zn were detected by the diethylenetriaminepentaacetic acid (DTPA) extraction method according to Wang et al. (Citation2017b). Briefly, 10 g soil was extracted by 20 mL 0.005 M DTPA + 0.01 M CaCl2 + 0.1 M triethanolamine (TEA; pH 7.0). The slurry was shaken at 180 rpm for 2 h and the filtered solution was used to determine the concentrations of available micronutrients by AAS. Although the DTPA method was highly recommended by the book of Soil Fertility and Fertilizers: An introduction to Nutrient Management for the measurement of available micronutrients, the secondary mineral forms of the micronutrients cannot be detected by this method. Soil organic matter (SOM) was determined by the method of dichromate oxidation and titration with ferrous ammonium sulfate (Lu Citation2000). Soil microbial biomass carbon (MBC) and microbial biomass nitrogen (MBN) were measured by the fumigation-extraction method according to Vance et al. (Citation1987) within 7 days after soil sampling. Soil, rice straw, chicken manure and vermicompost were digested by Nitric acid (HNO3)-hydrofluoric acid (HF)-perchloric acid (HClO4). Briefly, 7 mL HF and 1 mL HNO3 was added to a 30 ml teflon crucible which contains about 0.1 g soil or 0.5 g organic materials. Then the teflon crucible was heated at about 200°C until the acid liquor almost dry out. Two milliliter HClO4 was added to the same teflon crucible after the teflon crucible cool down. Once again, the teflon crucible was heated at about 200°C until the acid liquor became clear. Total N in the digested solution was measured by the Kjeldahl method, total P was measure by the molybdenum blue colorimetric method, total K was determined by flame photometer, base cations and micronutrients were measured by AAS (Lu Citation2000). Mineral fertilizers were digested by nitric acid-hydrofluoric acid-perchloric acid, concentrations of base cations and micronutrients were measured by AAS (Lu Citation2000).
2.3. Statistical analyses
Three-way ANOVAs were used to test the effects of planting time (Y), growth stage (S), and fertilizer treatment (T) on exchangeable base cations and available micronutrients. Multiple comparisons with Duncan design were conducted to determine differences in base cations and micronutrients among planting times. Pearson correlation analysis was performed to examine the relationships between base cations, micronutrients and pH, SOM, MBC, and MBN. All statistical analyses were performed with SPSS 19.0 (SPSS, Inc., Chicago, IL, USA) and statistical significance was accepted at P < 0.05.
3. Results
3.1. Soil exchangeable base cations
The concentration of exchangeable Ca decreased significantly with the increase of cultivation duration (), about 0.5 g kg−1 exchangeable Ca lost during the past 20 year planting of tomato. No significant increase or decrease trend of exchangeable Ca was found under all the treatments compared with Con. The concentration of exchangeable Ca decreased during the growth period under CM treatment in all the three soils. Three-way ANOVAs showed that the concentration of exchangeable Ca was significantly influenced by Y and S (, P < 0.001 and 0.05, respectively), but no T effect was found. Soil exchangeable Mg concentration ranged from 60 mg kg−1 to 140 mg kg−1, it increased after planting of tomato for 5 years, then decreased as the planting duration up to 20 years (). Compared with Con, CM increased concentration of exchangeable Mg in 0- and 5-year soils in early growth period of tomato (FP and FSP), whereas CF significantly decreased concentrations of exchangeable Mg at the same period. The concentration of exchangeable Mg exhibited significant Y, S, and T effects. In contrast to exchangeable Ca, soil exchangeable K exhibited a significant increasing trend with the increase of cultivation duration, irrespective of treatment (). After 20 year planting of tomato, average concentration of soil exchangeable K increased by about 63%. Compared with Con, all the treatments significantly increased exchangeable K content, with the highest content was found under the CM treatment, which was up to approximately 1200 mg kg−1. Differences of exchangeable K content between organic fertilizer treatments (CM and VM) and Con increased with the increase of cultivation duration. Concentrations of exchangeable K sharply decreased during the tomato growth season, especially between FSP and EHP. The concentrations of exchangeable K exhibited significant Y, S, and T effects.
Table 3. Results (F-values) of ANOVAs on the effects of cultivation year (Y), growth stage (S) and treatment (T) on soil exchangeable Ca, Mg, K, and available Fe, Mn, Cu, and Zn.
Figure 1. Effects of fertilization, growth period, and cultivation duration on soil exchangeable Ca (a), Mg (b), and K (c). Treatments include no fertilization as control (Con), chicken manure fertilization (CM), chemical fertilizer fertilization (CF), fresh rice straw fertilization (FS), and vermicompost fertilization (VM). Growth periods include flowering period (FP), fruit set period (FSP), early harvest period (EHP), and late harvest period (LHP). Cultivation durations include 0 year, 5 years, and 20 years. Data are represented as means ± standard error (n = 4). The capital letters indicate significant differences between different cultivation durations.
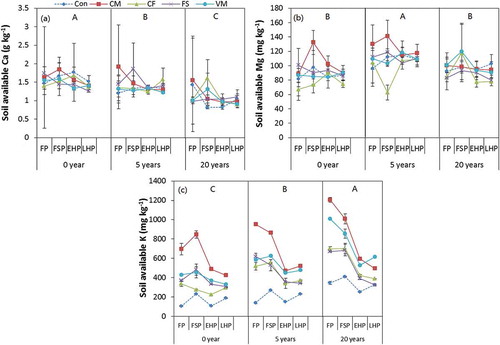
3.2. Soil available micronutrients
There was a significant inter-annual variation in the concentration of available Fe (, P < 0.05). Compared with 0-year soil, the average concentration of soil available Fe increased by 125.7% and 347.0% in 5-year and 20-year soils, respectively. However, the concentration of available Fe sharply fluctuated during different growth stages. The maximal value of available Fe under 5-year soil was larger than that of the minimum value under 20-year soil, irrespective of treatment. Similar to available Fe, average concentration of available Mn significantly increased with the extension of cultivation duration (, P < 0.05). The concentration of available Mn was occasionally higher in 0-year soil than 20-year soil. The average concentration of available Cu increased by 60.7% in 5-year soil compared with 0-year soil. However, the average concentration of available Cu in 20-year soil was about 11 and 7 fold higher than in 0- and 5-year soils, respectively (). Sharp fluctuation of the available Cu during tomato growth reason was only found on the 20-year soil. The average concentration of available Zn significantly increased with increase of cultivation duration (, P < 0.05). The concentration of soil available Zn was significantly higher under CF treatment than other treatments, irrespective of tomato cultivation duration and growth stage. Three-way ANOVAs analysis indicated that all the three factors, Y, S, and T, and their interaction had significant effects on the concentration of soil micronutrients (, P < 0.001).
Figure 2. Effected of fertilization, growth period and cultivation duration on soil available Fe (a), Mn (b), Cu (c), and Zn (d). Treatments include no fertilization as control (Con), chicken manure fertilization (CM), chemical fertilizer fertilization (CF), fresh rice straw fertilization (FS), and vermicompost fertilization (VM). Growth periods include: flowering period (FP), fruit set period (FSP), early harvest period (EHP), and late harvest period (LHP). Cultivation durations include 0 year, 5 years, and 20 years. Data are represented as means ± standard error (n = 4). The capital letters indicate significant differences between different cultivation durations.
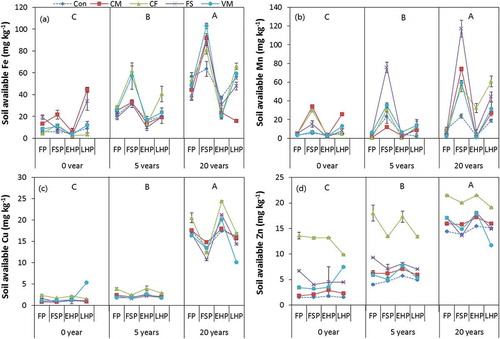
3.3. Soil pH, SOM, and MBC
Soil pH was significantly lower in 20-year soil than in 0-year and 5-year soils (). The highest soil pH during tomato growth period was found at EHP stage, regardless of tomato cultivation duration and treatment. In general, pH in CF treatment was lower than in Con and organic fertilizer treatments. Soil organic carbon increased with the increase of planting duration (), and firstly increased and then decreased during tomato growth period in all the soils and treatments. All the treatments had higher SOC than Con in most of the growth stages in all the three soils. Soil microbial biomass C had increasing trend with the increase of planting duration (). Generally, MBC was higher in organic fertilizer treatments than in CF and Con.
Figure 3. Effected of fertilization, growth period and cultivation duration on soil pH (a), soil organic matter (SOM) (b), and soil microbial biomass (SOM) (d). Treatments include: no fertilization as control (Con), chicken manure fertilization (CM), chemical fertilizer fertilization (CF), fresh rice straw fertilization (FS) and vermicompost fertilization (VM). Growth periods include flowering period (FP), fruit set period (FSP), early harvest period (EHP), and late harvest period (LHP). Cultivation durations include 0 year, 5 years and 20 years. Data are represented as means ± standard error (n = 4). The capital letters indicate significant differences between different cultivation durations.
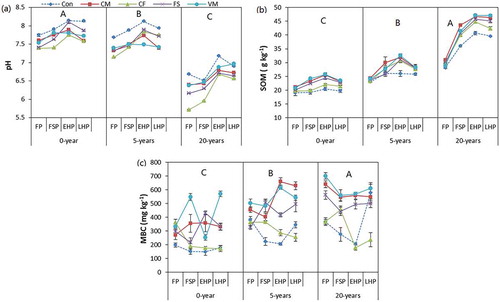
3.4. Relationships between base cations, micronutrients, and selected soil properties
The concentration of exchangeable Ca significantly and positively correlated with soil pH (, P < 0.01). No relationship was observed between exchangeable Mg and soil pH (). On the contrary, significant negative relationships were observed between exchangeable K, micronutrients and soil pH (–, P < 0.01).
Figure 4. The relationships between soil pH and exchangeable Ca (a), Mg (b), K (c), and available Fe (d), Mn (e), Cu (f), and Zn (g) across all treatments and cultivation duration during different growth periods.
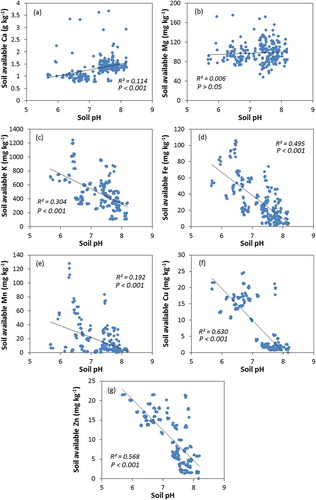
SOM was significantly and negatively correlated with exchangeable Ca (, P < 0.001), whereas significantly and positively correlated with exchangeable K and micronutrients (–G, P < 0.001).
Figure 5. The relationships between soil organic matter (SOM) and exchangeable Ca (a), Mg (b), K (c), and available Fe (d), Mn (e), Cu (f), and Zn (g) across all treatments and cultivation duration during different growth periods.
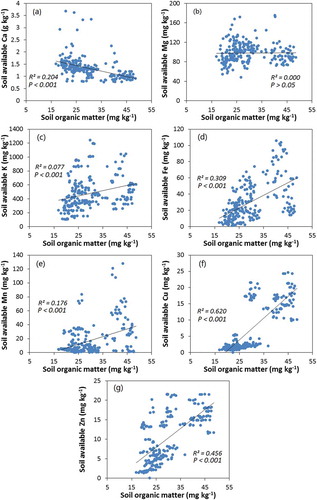
The concentration of exchangeable K significantly and positively correlated with soil MBC and MBN ( and 6B), P < 0.001). When data were analyzed individually for each cultivation duration (0 year, 5 years, and 20 years), significant positive relationships still exist between exchangeable K and MBC and MBN ( and 6B).
Figure 6. The relationships between soil exchangeable K and soil microbial biomass C (a) and biomass N (b) within each cultivation duration (0 year, 5 years, and 20 years) and across all the cultivation durations (total).
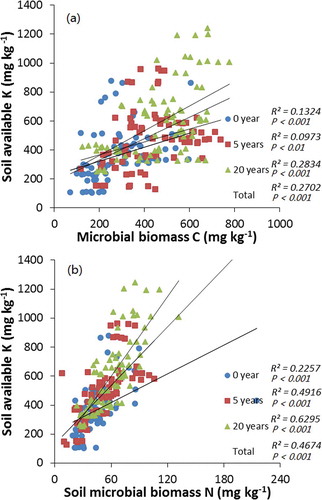
Significant relationships between the available micronutrients and pH and SOM were occasionally observed, but no constant significant relationship was found within each cultivation duration ().
Table 4. Pearson correlation analyses (R values) of micronutrients with soil pH and soil organic matter (SOM).
4. Discussion
4.1. Responses of base cations and micronutrients to cultivation duration
4.1.1. Soil exchangeable base cations
Previous studies indicated that soil exchangeable base cations exhausted under long-term input of chemical fertilizers, especially N fertilizer (Hoegberg et al. Citation2006; Guo et al. Citation2010; Lu et al. Citation2014). In this study, exchangeable Ca significantly decreased with the increase of cultivation duration. However, exchangeable Mg and K showed no decline after 20-year cultivation (), which rebutted our hypothesis that long-term cultivation of tomato will cause decrease of base cations because of intensive use of chemical fertilizers. Significant decrease of soil exchangeable Ca after long-term tomato planting ascribes to the preferential exchange of Ca with proton in soil colloid surface and subsequent leaching with water, and uptake by tomato plant (Lucas et al. Citation2016). Magnesium dynamic is more complex during the 20 year cultivation, firstly increased and subsequently decreased (). This phenomenon mainly results from (1) preferential uptake of Ca by tomato plant giving more space for the retention of exchangeable Mg in short-term cultivation soil. No obvious decreasing trend of soil available Mg during the growth of tomato in 0- and 5-year soils suggested that removing of Mg by tomato plant is not significant (); and (2) leaching with water from surface to deep layer soil after the exhaustion of Ca in long-term cultivation soil. The increase of exchangeable K () after 5 and 20 years cultivation has mainly contributed to K fertilization. In order to raise the yield and quality of tomato, K has been considered to be the key nutrient to production of quality fruits is intensively used in the greenhouse in northeast China (Usherwood Citation1985). Annual use of K reached about 750 kg ha−1 in greenhouse production in China, which has been far beyond the need of tomato production, and then accumulated in soil.
4.1.2. Soil available micronutrients
Deficiency of micronutrients due to plant uptake after long-term cultivation of crop has been recognized (Singh Citation2008). However, in this study, we observed the opposite result: soil available micronutrients significantly increased with the cultivation of tomato (). The availability of micronutrients strongly depend on the balance among input (mineral weathering release and atmospheric deposition), recycling (plant biomass turnover), retention (sorption by soil surface charge or complexing with organic matter), and output (biomass remove and hydrologic leaching) (Li et al. Citation2008). In our study, soil available micronutrients negatively correlated with soil pH, while positively correlated with SOM (–G and –G), which implied that soil acidification after long-term N addition enhanced mineral weathering and therefore release of micronutrients (input), at the same time, the increase of SOM strengthen the retention of micronutrients by adsorption (retention). Both the two aspects work together to make the increase of soil available micronutrients.
4.2. Responses of base cations and micronutrients to growth stage of tomato
4.2.1. Concentrations of exchangeable base cations
Contrasted with our second hypothesis, no constant increase of soil exchangeable Ca and Mg under organic fertilizer treatments (rice straw, chicken manure and vermicompost) was found ( and 1B). Previous long-term experiment indicates that application of organic fertilizers can maintain and restore soil exchangeable base cations, especially exchangeable Ca and Mg (Cai et al. Citation2015). Increase of exchangeable base cations under manure and crop straw additions ascribe to (1) alleviate of soil acidity, which can weaken base cations loss, (2) increase of SOM enhance the adsorption capacity of base cations by SOM (Oorts et al. Citation2003), and (3) replenishment of base cations by manure and crop straw (Xu et al. Citation2006; Cai et al. Citation2015). All of these aspects are long-term processes. In this study, the soils only been treated for one year, it is too short to have positive effect on the concentration of exchangeable Ca and Mg. However, compared with Con all treatments significantly increased soil exchangeable K (), which may ascribe to the K solubilizing microorganisms. The differences of soil exchangeable K content between organic fertilization treatments (CM and VM) and Con are obviously higher than the added amount of K (about 332 mg K kg−1 soil), which implies that part of the exchangeable K came from another source. Numerous microorganisms in soil have been verified can promote the release of K fixed in mineral lattice (Sheng and He Citation2006; Lian et al. Citation2008; Meena et al. Citation2014). Those microorganisms (termed as K solubilizing bacteria) are mainly composed of bacteria belong to Pseudomonas and Bacillus genera (Gu et al. Citation2013; Saha et al. Citation2016). Our previous study revealed that the relative abundances of Pseudomonas and Bacillus genera were relatively higher in organic fertilizer treatments than in CF and Con (Zhao et al. Citation2019). We also observed that exchangeable K is positively related to soil microbial biomass regardless of cultivation duration ( and 6B), increase of microbial biomass combined with the high relative abundance of K solubilizing bacteria may explain the extra increase of exchangeable K content under the addition of organic fertilizers compared with CF. Generally, concentrations of exchangeable Ca and K decreased with tomato growth, whereas Mg has no obvious decrease trend () indicate that Ca and K, but not Mg should be preferentially supplied in the production of tomato.
4.2.2. Concentrations of available micronutrients
Soil available Fe, Mn, and Cu exhibit no constant significant treatment effects during the tomato growth season (–C), which may due to short treatment time. The inputs of Fe, Mn, and Cu accompany with the additions of rice straw, chicken manure and vermicompost are 14.4–1.9, 5.3–0.3, and 0.54–0.33 mg kg−1, respectively. Inputs of these macronutrients from fertilizers were relatively lower than the needs of tomato growth. However, concentration of available Zn was significantly higher under chemical fertilizers application than other treatments (). The amount of available Zn bring to soil by chemical fertilizers was slight, was far less than 1 mg kg−1 (). We deem that the high Zn availability is mainly due to secondary mineral dissolution after soil acidification under chemical fertilizer application. Concentrations of available Fe and Mn fluctuated sharply during the growth season of tomato. Previous study suggested that availabilities of Fe and Mn greatly depend on soil redox condition which may contribute to the fluctuation of these micronutrients during the tomato growth period (Schwab and Lindsay Citation1983). However, it is impossible to form strict anaerobic condition in our soils because of the irrigation system used in this study was drip irrigation. We found that pH exhibited opposite development trends with soil available Fe and Mn during the growth of tomato ( and ), which indicated that greater acidity released Fe and Mn from minerals may contribute to the fluctuation of these micronutrients. Combined with the previous study that the ideal ranges of Fe, Mn, Cu, and Zn for tomato growth were 100–300, 60–240, 5–20, and 20–50 mg kg−1, respectively (Rosen and Eliason Citation2005), our results indicated that although the average concentrations of available Fe and Mn increased after long-term intensive application of chemical fertilizers, the deficiencies of these nutrients can also occurred for their lower availabilities in key growth stages. Measurement of availabilities of Fe and Mn after harvesting of crop, which has been widely used in agricultural practice is improper; their availabilities should be monitored on the key growth stages of crop.
5. Conclusion
Long-term planting of tomato with the application of chemical fertilizers will cause depletion of available Ca and Mg, whereas increased the availability of micronutrients. Soil pH and SOM are the most important two factors influencing availabilities of base cations and micronutrients. The beneficial effects of organic fertilizers on availability of base cations and micronutrients should be a long-term process, and accompanied additions of micronutrients are need. Our findings suggest that depletion of soil available Ca, but not Mg, and micronutrients are the main limit factors for the production of tomato after long-term intensive application of chemical fertilizers. The availabilities of Fe and Mn after harvesting of crop should not on behalf of the supply capacity of these nutrients for crop growth. In the production of vegetables in solar greenhouse, monitoring of micronutrients availability should be conducted on key growth stages to get high yield.
Additional information
Funding
References
- Alloway BJ 2008: Micronutrients and crop production: an introduction. In Micronutrient Deficiencies in Global Crop Production, Ed. Alloway BJ, pp. 1–39. Dordrecht: Springer Netherlands.
- Bitondo D, Tabi FO, Kengmegne SSA, Ngoucheme M, Mvondoze AD 2013: Micronutrient concentrations and environmental concerns in an intensively cultivated typic dystrandept in mount bambouto, cameroon. Open J. Soil Sci, 3(6), 283–288. doi:10.4236/ojss.2013.36033.
- Cai Z, Wang B, Xu M, Zhang H, He X, Zhang L, Gao S 2015: Intensified soil acidification from chemical N fertilization and prevention by manure in an 18-year field experiment in the red soil of southern China. J. Soil Sediment, 15, 260–270. doi:10.1007/s11368-014-0989-y
- Cusack DF, Macy J, Mcdowell WH 2016: Nitrogen additions mobilize soil base cations in two tropical forests. Biogeochemistry, 128(1–2), 67–88. doi:10.1007/s10533-016-0195-7.
- Dotaniya M, Meena V 2015: Rhizosphere effect on nutrient availability in soil and its uptake by plants: a review. Proceedings of the National Academy of Sciences, India Section B: Biol. Sci, 85, 1–12. doi:10.1007/s40011-013-0297-0
- Fageria NK, Slaton NA, Baligar VC 2003: Nutrient management for improving lowland rice productivity and sustainability. Adv. Agron, 80, 63–152.
- Fu H, Zhang G, Zhang F, Sun Z, Geng G, Li T 2017: Effects of continuous tomato monoculture on soil microbial properties and enzyme activities in a solar greenhouse. Sustainability, 9, 317. doi:10.3390/su9020317
- Gruba P, Mulder J 2015: Tree species affect cation exchange capacity (CEC) and cation binding properties of organic matter in acid forest soils. Sci. Total Environ, 511, 655–662. doi:10.1016/j.scitotenv.2015.01.013
- Gu FQ, Ji XL, Wei YL 2013: Research and progress on soil silicate bacteria. Chin. J. Microecol, 25, 609–616.
- Guo J, Liu X, Zhang Y, Shen J, Han W, Zhang W, Christie P, Goulding K, Vitousek P, Zhang F. 2010: Significant acidification in major Chinese croplands. Science, 327, 1008–1010. doi:10.1126/science.1182570
- Han J, Shi J, Zeng L, Xu J, Wu L 2015: Effects of nitrogen fertilization on the acidity and salinity of greenhouse soils. Environ. Sci. Pollut. Res, 22, 2976–2986. doi:10.1007/s11356-014-3542-z
- Hang XS, Wang HY, Zhou JM, Ma CL, Du CW, Chen XQ 2009: Risk assessment of potentially toxic element pollution in soils and rice (Oryza sativa) in a typical area of the Yangtze River delta. Environ. Pollut, 157, 2542–2549. doi:10.1016/j.envpol.2009.03.015
- Hoegberg P, Fan H, Quist M, Binkley D, Tamm CO 2006: Tree growth and soil acidification in response to 30 years of experimental nitrogen loading on boreal forest. Global Change Biol, 12, 489–499. doi:10.1111/j.1365-2486.2006.01102.x
- Ju XT, Kou CL, Christie P, Dou ZX, Zhang FS 2007: Changes in the soil environment from excessive application of fertilizers and manures to two contrasting intensive cropping systems on the North China plain. Environ. Pollut, 145, 497–506. doi:10.1016/j.envpol.2006.04.017
- Kopittke PM, Dalal RC, Menzies NW 2017: Changes in exchangeable cations and micronutrients in soils and grains of long-term, low input cropping systems of subtropical Australia. Geoderma, 285, 293–300. doi:10.1016/j.geoderma.2016.10.011
- Li H, Yang X, Weng B, Su J, Nie S, Gilbert JA, Zhu Y-G 2016: The phenological stage of rice growth determines anaerobic ammonium oxidation activity in rhizosphere soil. Soil Biol. Biochem, 100, 59–65. doi:10.1016/j.soilbio.2016.05.015
- Li J, Mendoza A, Heine P 2008: Four-decade responses of soil trace elements to an aggrading old-field forest: B, Mn, Zn, Cu and Fe. Ecology, 89, 2911–2923. doi:10.1890/07-1381.1
- Lian B, Wang B, Pan M, Liu C, Teng HH 2008: Microbial release of potassium from K-bearing minerals by thermophilic fungus Aspergillus fumigatus. Geochim. Cosmochi, 72, 87–98. doi:10.1016/j.gca.2007.10.005
- Lim SL, Wu TY, Lim PN, Shak KPY 2015: The use of vermicompost in organic farming: overview, effects on soil and economics. J. Sci. Food Agri, 95(6), 1143–1156. doi:10.1002/jsfa.6849.
- Lu R 2000: Soil Agricultural Chemical Analysis Method, pp.1–315. China Agricultural Science and Technology Press, Beijing.
- Lu X, Mao Q, Gilliam FS, Luo Y, Mo J 2014: Nitrogen deposition contributes to soil acidification in tropical ecosystems. Global Change Biol, 20, 3790–3801. doi:10.1111/gcb.12665
- Lucas R, Klaminder J, Futter M, Bishop KH, Egnell G, Laudon H, Högberg P 2016: A meta-analysis of the effects of nitrogen additions on base cations: implications for plants, soils, and streams. Forest Ecol. Manag, 262, 95–104. doi:10.1016/j.foreco.2011.03.018
- Meena VS, Maurya BR, Verma JP 2014: Does a rhizospheric microorganism enhance K+ availability in agricultural soils? Microbiol. Res, 169, 337–347. doi:10.1016/j.micres.2013.09.003
- Ochoa-Hueso R, Bell MD, Manrique E 2014: Impacts of increased nitrogen deposition and altered precipitation regimes on soil fertility and functioning in semiarid mediterranean shrublands. J. Arid Environ, 104, 106–115. doi:10.1016/j.jaridenv.2014.01.020
- Oorts K, Vanlauwe B, Merckx R 2003: Cation exchange capacities of soil organic matter fractions in a ferric lixisol with different organic matter inputs. Agr. Ecosyst. Environ, 100, 161–171. doi:10.1016/S0167-8809(03)00190-7
- Peng X, Zhu QH, Xie ZB, Darboux F, Holden NM 2016: The impact of manure, straw and biochar amendments on aggregation and erosion in a hillslope Ultisol. Catena, 138, 30–37. doi:10.1016/j.catena.2015.11.008
- Perakis SS, Sinkhorn ER, Catricala CE, Bullen TD, Fitzpatrick JA, Hynicka JD, Cromack K 2013: Forest calcium depletion and biotic retention along a soil nitrogen gradient. Ecol. Appl, 23, 1947–1961. doi:10.1890/12-2204.1
- Rosen CJ, Eliason R 2005: Nutrient Management of Commercial Fruit and Vegetable Crops in Minnesota. BU-05886. University of Minnesota Extension Service. Department of Soil, Water and Climate, Saint Paul.
- Saha M, Maurya BR, Meena VS, Bahadur I, Kumar A 2016: Identification and characterization of potassium solubilizing bacteria (KSB) from indo-gangetic plains of India. Biocatal. Agricul. Biotech, 7, 202–209.
- Schwab A, Lindsay W 1983: Effect of redox on the solubility and availability of iron. Soil Sci. Soc. Am. J, 47, 201–205. doi:10.2136/sssaj1983.03615995004700020005x
- Sheng XF, He LY 2006: Solubilization of potassium-bearing minerals by a wild-type strain of bacillus edaphicus and its mutants and increased potassium uptake by wheat. Can. J. Microbio, 52, 66–72. doi:10.1139/w05-117
- Singh MV 2008: Micronutrient deficiencies in crops and soils in India. In Micronutrient Deficiencies in Global Crop Production, Ed. Alloway WH, pp. 93–125. Springer-Science.
- Taylor MD, Locascio SJ 2004: Blossom-end rot: a calcium deficiency. J. Plant Nutr, 27, 123–139. doi:10.1081/PLN-120027551
- Tomlinson GH 2003: Acidic deposition, nutrient leaching and forest growth. Biogeochemistry, 65, 51–81. doi:10.1023/A:1026069927380
- Turner BL, Yavitt JB, Harms KE, Garcia MN, Wright SJ 2015: Seasonal changes in soil organic matter after a decade of nutrient addition in a lowland tropical forest. Biogeochemistry, 123(1–2), 221–235.
- Usherwood NR 1985: The role of potassium in crop quality. In Potassium in Agriculture, Ed.Munson R, pp. 489–513. Madison WI: ASA, CSSA and SSSA.
- Vance E, Brookes P, Jenkinson D 1987: An extraction method for measuring soil microbial biomass C. Soil Biol. Biochem, 19, 703–707. doi:10.1016/0038-0717(87)90052-6
- Wang R, Dungait JA, Buss HL, Yang S, Zhang Y, Xu Z, Jiang Y 2017b: Base cations and micronutrients in soil aggregates as affected by enhanced nitrogen and water inputs in a semi-arid steppe grassland. Sci. Total Environ, 575, 564–572. doi:10.1016/j.scitotenv.2016.09.018
- Wang R, Zhang Y, Cerdà A, Cao M, Zhang Y, Yin J, Jiang Y, Chen L 2017a: Changes in soil chemical properties as affected by pyrogenic organic matter amendment with different intensity and frequency. Geoderma, 289, 161–168. doi:10.1016/j.geoderma.2016.12.006
- Wang Y, Han W, Wang X, Chen H, Zhu F, Wang X, Lei C 2017: Speciation of heavy metals and bacteria in cow dung after vermicomposting by the earthworm, Eisenia fetida. Bioresource Technol, 245, 411–418. doi:10.1016/j.biortech.2017.08.118
- Wei X, Hao M, Shao M, Gale WJ 2006: Changes in soil properties and the availability of soil micronutrients after 18 years of cropping and fertilization. Soil Till. Res, 91, 120–130. doi:10.1016/j.still.2005.11.009
- White PJ, Broadley MR 2003: Calcium in plants. Ann. Bot, 92, 487–511. doi:10.1093/aob/mcg164
- Wu H, Xiong DH, Xiao L, Zhang S, Yuan Y, Su ZA, Zhang BJ, Yang D 2018: Effects of vegetation coverage and seasonal change on soil microbial biomass and community structure in the dry-hot valley region. J. Mount. Sci, 15(7), 1546–1558. doi:10.1007/s11629-017-4650-2.
- Xu J, Tang C, Chen ZL 2006: The role of plant residues in pH change of acid soils differing in initial pH. Soil Biol. Biochem, 38, 709–719. doi:10.1016/j.soilbio.2005.06.022
- Yang W, Li Z, Cai C, Guo Z, Chen J, Wang J 2013: Mechanical properties and soil stability affected by fertilizer treatments for an ultisol in subtropical China. Plant Soil, 363(1–2), 157–174. doi:10.1007/s11104-012-1266-8.
- Yang X, Li Q, Tang Z, Zhang W, Yu G, Shen Q, Zhao FJ 2017: Heavy metal concentrations and arsenic speciation in animal manure composts in China. Waste Manage, 64, 333–339.
- Zhang Y, Zhang S, Wang R, Cai J, Zhang Y, Li H, Huang S, Jiang Y 2016: Impacts of fertilization practices on pH and the pH buffering capacity of calcareous soil. Soil Sci. Plant Nutr, 62, 432–439. doi:10.1080/00380768.2016.1226685
- Zhao F, Zhang Y, Dijkstra FA, Li Z, Zhang Y, Zhang T, Lu Y, Shi J, Yang L 2019: Effects of amendments on phosphorous status in soils with different phosphorous levels. Catena, 172, 97–103. doi:10.1016/j.catena.2018.08.016
- Zhu W, Yao W, Shen X, Zhang W, Xu H 2018: Heavy metal and δ13C value variations and characterization of dissolved organic matter (DOM) during vermicomposting of pig manure amended with 13C-labeled rice straw. Environ. Sci. Pollut. R, 25(20), 20169–20178.
- Zingore S, Delve RJ, Nyamangara J, Giller KE 2008: Multiple benefits of manure: the key to maintenance of soil fertility and restoration of depleted sandy soils on African smallholder farms. Nutr. Cycl. Agro, 80, 267–282. doi:10.1007/s10705-007-9142-2