ABSTRACT
The aim of study was to estimate how the deadwood of different tree species in various stages of decomposition releases dissolved organic carbon (DOC) and total nitrogen concentration. The deadwood of seven species (Common alder, Common aspen, Common ash, Silver fir, Norway spruce, Common hornbeam, and Silver birch) were selected. Three logs from each species in the third, fourth, and fifth decay classes were chosen for analysis. The investigation was carried out in Czarna Rózga Reserve in Central Poland. The leachate from deadwood was collected in lysimeters. Concentrations of total carbon (TC), inorganic carbon (IC), total organic carbon (TOC), and total nitrogen (TN) were determined. In our investigations, the concentration of TOC measure in filtered water samples was equal to DOC. Additionally, pH and electrical conductivity (EC) values were determined in water samples. The results confirmed the importance of the wood species and the stage of decomposition on the concentration of DOC and TN. Significant differences in the concentration of DOC and TN in leachates released between coniferous and deciduous species were noted. Most DOC and TN were released from decomposing hornbeam and aspen wood, the least DOC, and TN were released into the soil from coniferous species, especially the spruce.
1. Introduction
Dead trees are an important element of properly functioning forest ecosystem and play a very important role in the maintenance of biodiversity, soil fertility, and participate in water retention (Persiani et al. Citation2010; Pichler et al. Citation2011; Cocciufa et al. Citation2014). The amount and type of deadwood is the result of an activity of numerous factors that occur in forest ecosystems, depending on the species composition of the tree stand and trees dimensions, habitat conditions, and especially thermal and humidity conditions (Yin Citation1999; Fravolini et al. Citation2016). Deadwood is a very important carbon reservoir (Lassauce et al. Citation2011; Błońska et al. Citation2017). The carbon fraction in wood is approximated 50% of the woody dry mass (Coomes et al. Citation2002; Weiss et al. Citation2000). During deadwood decomposition, carbon is partially transferred to the environment, thereby increasing C amounts in the soil. Europe’s soils store 26 Tg C yr−1, which accounts for 30–50% of carbon resources in forest biomass (Liski et al. Citation2002). Mechanisms responsible for carbon stabilization in the soil are of an interest to many researchers due to their importance in global carbon cycle understanding. The way of carbon storage in soil is soil organic matter (SOM), which amount is affected by the deadwood. Błońska et al. (Citation2017) results confirmed that the amount of C depends on the decay class of the deadwood. Despite the growing number of deadwood studies, we still have limited knowledge of the dynamics of transformations of carbon released from deadwood into the soil.
The main processes involved in decomposition of deadwood are heterotrophic respiration, biological transformation, physical fragmentation, and leaching (Russell et al. Citation2015; Magnússon et al. Citation2016). Of the abovementioned processes related to deadwood decomposition, the last is responsible for dissolved organic carbon (DOC) transport to the soil. DOC is the basic mean of the transport of carbon released during deadwood decomposition into the soil (Kalbitz and Kaiser Citation2008; Kahl et al. Citation2012). DOC contributes to the formation and accumulation of SOM (Kahl et al. Citation2012; Bantle et al. Citation2014), it facilitates metal and organic pollutant mobilization (Hafner et al. Citation2005) and changes soil microbial communities (Cooper et al. Citation2016). DOC is the major source of carbon and energy to sustain heterotrophic microbial communities (Baker et al. Citation2000). Spears et al. (Citation2003) noted a higher concentration of DOC derived from deadwood logs than from the layer of the litter. Kuehne et al. (Citation2008) found DOC concentration in runoff from Fagus logs increasing with decomposition stage from 28 to 118 mg L−1. DOC release from deadwood is tree species specific in terms of quantity and quality (Bantle et al. Citation2014). Also N is released during deadwood decomposition, and it is transferred to the soil (Yang et al. Citation2010). The nitrogen stimulates the growth of soil microorganisms (Strachel et al. Citation2017) and it can affect the structure and composition of soil microbial communities (Yan et al. Citation2017). The C and N dynamics in decomposing of deadwood are species specific (Palviainen et al. Citation2008).
The purpose of our study was to evaluate how the deadwood of different tree species in different decomposition stages affects the concentration of dissolved organic carbon and nitrogen in leachate released into the soil. Both deciduous and coniferous species most commonly found in temperate climate forest ecosystems were selected for analysis. Selecting three different stages of decomposition (III, IV, and V), we attempted to show the changes in the concentration in leachate of released C and N relative to the stage of deadwood decomposition. The following hypotheses were tested in this study: (1) leaching of C and N differ between tree species, especially between coniferous and deciduous species; (2) the degree of wood decomposition determines the concentration of C and N in leachate.
2. Materials and methods
2.1. Sampling sites
The investigation was performed in Czarna Rózga Reserve in Central Poland (50°59’37N; 20°1’5E). The tree stands within the area of the reserve are of natural origin, and the dominant species is the alder. The study area is characterized by the following conditions of climate: the average annual rainfall is 649 mm, the average annual temperatures amount to 7.4°C and the length of the vegetation season lasts 200–210 days. During the experiment period (from the beginning of March to the end of May 2017), the average temperature was 6°C and the monthly precipitation was in the range from 50 to 90 mm. Sample plots were located in the area with a predominance of fluvioglacial sand and loam with Gleysols, Cambisols, and Podzols (WRB (World Reference Base For Soil Resource) Citation2014).
2.2. Experimental design
The logs of seven species of trees in three stages of decomposition (III, IV, and V) were selected for the study. The deadwood Common alder (Alnus glutinosa), Common aspen (Populus tremula), Common ash (Fraxinus excelsior), Silver fir (Abies alba), Norway spruce (Picea abies), Common hornbeam (Carpinus betulus), and Silver birch (Betula pendula) were included in the research. The species included in the study were divided into two groups: coniferous (Silver fir and Norway spruce) and deciduous (Common alder, Common aspen, Common ash, Common hornbeam, and Silver birch trees). The decay classes (DC) of logs were evaluated with classification of dead trees in Maser et al. (Citation1979) (). Deadwood was located in stands well maintained, without any signs of weakening. The canopy density was 0.7. The leachate from the deadwood which means water percolating the wood, was collected in lysimeters. Under each log, one lysimeter was installed at the stem midpoint (log diameter >25 cm). The lysimeters were constructed of polyethylene tubes with a width of 300 mm and 300 mm length (). The polyethylene tube was installed under the logs and stabilized by metal handles. The gap occurred as a result of irregular shapes of logs was sealed with silicone. The silicone served as protection against the inflow of rain directly to the plastic bottle. The plastic funnel was placed between the polyethylene tubes and plastic hose and the plastic funnel was filled with quartz sand (ø 0.5–1.0 mm). The filtrate went through the lysimeter bottom and plastic hose and was collected in a plastic bottle located below the log, in the soil at the depth of 0–20 cm. The filtrate which would naturally reach the surface horizons of soil passed through to the plastic bottle placed at the depth of 0–20 cm and so had no direct contact with soil. In the case of logs in V decay class due to the texture of wood the most difficult it was placing the polyethylene tube. All loose fragments of the log on the designated length were placed in the polyethylene tube. In the case of logs in V decay classes the silicone protection was not necessary because the polyethylene tube adhered to soft wood. Water samples for analysis were collected at two week intervals in the period from the beginning of March to the end of May 2017, including snow melt period. For analysis, 100 ml water samples were collected with 42 samples of water being taken in one series (7 species × 3 decay classes × 2 repetitions). Additionally the samples of rain on the onset and at the end of the experiment were collected for the analysis. The throughfall samples were taken in three repetitions. The polyethylene tube was used to collect throughfall samples. Using the data of the Agrometeorological Bulletins on the precipitation in individual months of the previous years and based on our own observation of the area, a decision was made to obtain deadwood filtrates in the spring. The precipitation and melting water were present in the period from March to May and it would be possible to collect the required volume of filtrates. The collection of filtrates though was completed in May as there was a problem with obtaining the right volume of water for analysis. We decided to present the results as averages from March to May based on the obtained results and meteorological data. The experiment period was characterized by unchangeable thermal conditions, the temperature and the amount of rainfall was similar.
Table 1. Degrees of dead trees wood decomposition.
2.3. Laboratory analysis
Water samples collected from lysimeters were chemically analyzed in the Forest Environment and Recovery Geochemistry Laboratory at the Forest Faculty, the University of Agriculture in Krakow. Before the analysis, samples were stored at 4°C. The samples were filtered through 0.45 μm filters. Concentrations of total carbon (TC), inorganic carbon (IC), total organic carbon (TOC) and total nitrogen (TN) were determined using Shimadzu TOC analyzer (Shimadzu, Japan). In our investigations, the concentration of TOC measure in filtered water samples was equal to DOC. Additionally, pH and EC values were determined by a multifunctional computer instrument CX-741 Elmetron (Elmetron S.C., Zabrze, Poland). pH was determined by method according to the Polish norms PN-ISO 10390 (Citation1997).
2.4. Statistical analysis
The differences between the mean values were evaluated with the Kruskal-Wallis test. In order to reduce the number of variables in the statistical data set and to visualize the multivariate data set as a set of coordinates in a high-dimensional data space, the principal component analysis (PCA) method was used. PCA method was used to evaluate the relationships between properties and decay classes. The decay classes (qualitative variable) were converted into an artificial variable. The coding of variables was made according to the 0, 1 scheme. Pearson correlation coefficients between properties of leachate from deadwood different species in various decay classes were also calculated. General linear model (GLM) was used for the investigated the effect of the species and decay classes on DOC. The mean arithmetic and standard deviation (SD) for properties of leachates were calculated for different species in different decay classes. Both the mean and SD concerned the whole research period. The mean and SD for deciduous and coniferous group were calculated in the same way. The statistical significance of the results was verified at the significance level of alpha = 0.05. All the statistical analyzes were performed with Statistica 10 software (Statsoft, USA) (2010).
3. Results
The monthly precipitation during experiment period was 50–90 mm. Average throughfall was 60.8 mm · month−1. The mean amount of water collected in lysimeters was varied depending on the species and decay class (). Amount of water from deadwood in III DC it was in the range 2.4–4.2 mm · month−1, in IV DC it was 3.3–4.8 mm · month−1 and in V DC it was 4.1–7.2 mm · month−1. The pH of leachate derived from the wood of different tree species varied within different stages of decomposition and between them (). The pH of the leachates ranged from 3.85 to 6.27. The lowest pH was determined in the case of the leachate from fir wood in the V decomposition stage, and the highest in the leachate from aspen wood in the III decomposition stage. The electrical conductivity (EC) of the leachates of examined tree species was in the range of 0.100–1.237 mS · cm−1. EC for most species except birch and spruce increased with the advancement of wood decomposition (). In the case of birch and spruce, the inverse relationship was noted. The birch leachate in the III decomposition stage had an EC of 0.298 mS · cm−1, and in the V decomposition stage, the EC decreased to 0.100 mS · cm−1. In the case of spruce, EC decreased from 0.204 mS · cm−1 in the III decomposition stage to 0.173 mS · cm−1 in the V decomposition stage.
Table 2. Properties of leachate from deadwood different species in various decay classes (mean ± SD).
The differentiation in DOC concentration in the leachates from different tree species in different stages of decomposition was noted. Concentration of DOC ranged from 112.6 mg · L−1 for spruce in the III decomposition stage to 5452.9 mg · L−1 for aspen in the V decomposition stage (). In the third stage of decomposition, the highest concentrations of DOC were recorded in birch leachate (440 mg · L−1) and lowest in spruce leachate (112.6 mg · L−1). In the fourth and fifth decomposition stages, high concentrations of DOC were noted in the hornbeam and aspen leachates (). In all stages of decomposition, statistically significant differences in DOC content between the species were recorded (). The highest increase in DOC concentration in subsequent decomposition stages was noted for alder and aspen. An average concentration of DOC in ash and hornbeam leachates increased slightly over 90% between the III and V decomposition stages. The smallest increase in DOC concentration between the III and V decomposition stages was observed in the coniferous leachates (and increase exceeded 30% in the case of fir, and 20% in the case of spruce). The inverse relationship was recorded in birch leachates, where the mean DOC concentration decreased by 35% between the III and V decomposition stage. The most apparent differences (statistically significant) in the mean DOC concentration between deciduous and coniferous species were demonstrated in the IV and V decomposition stages. The lack of statistically significant differences in average DOC concentrations between deciduous and coniferous species were recorded in the leachates from the wood in the third decomposition stage (). The IC content changed with the stage of wood decomposition. There was a noticeable increase in IC concentrations along with the advancement of wood decomposition in the leachates of all species except birch and spruce (). IC concentration ranged from 5.2 mg · L−1 to 18.6 mg · L−1. Similar relationships as for DOC were noted in the case of TN. With the advancement of wood decomposition, TN concentration increased for most of examined tree species. The inverse relationship was recorded in a leachate of birch wood. An average TN concentration for this species decreased by more than 50% between the III and V decomposition stage. The highest average concentration of TN at a level of 79.4 mg · L−1 was recorded in the leachate from hornbeam wood in the fifth decomposition stage, slightly lower, i.e. 74.7 mg · L−1 was noted in the leachates from aspen (). The DOC concentrations in throughfall were always much smaller than in leachates from deadwood. The similar results as for DOC were recorded in the case of TN concentrations in throughfall (). Significant differences in TN concentration between deciduous and coniferous species were recorded in all stages of decomposition (). These differences were the smallest in the third stage of decomposition. Distinct differences were noted in the wood leachates in the IV and V decomposition stage.
Figure 2. Dissolved organic carbon (DOC) concentration (mg · L−1) for coniferous (C) and deciduous (D) species in different decay classes (III, IV, and V); different lowercase alphabets mean significant differences.
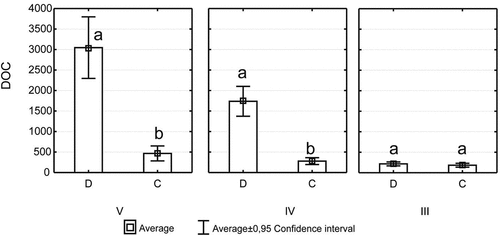
Figure 3. Total nitrogen (TN) concentration (mg · L−1) for coniferous (C) and deciduous (D) species in different decay classes (III, IV, and V); different lowercase alphabets mean significant differences.
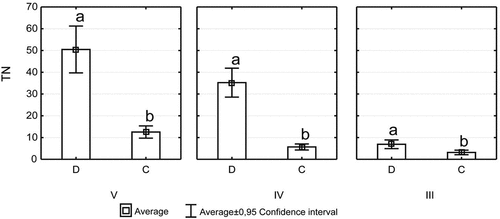
Concentration of DOC in the examined leachates strongly correlated with EC and TN concentration (). shows the relationship of DOC and EC (r = 0.91) and the relationship of TN and EC (r = 0.89). GLM analysis confirmed the simultaneous strong impact of the species and the stage of decomposition on DOC concentration (). presents a projection of variables on a plane of factors 1 and 2 distinguished in the PCA analysis. The first factor explains approx. 52% of the variables and it is related primarily to the properties of leachate from deadwood. The second factor explains approx. 17% of the variables and is linked to the rate of deadwood decomposition. PCA analysis confirms the strong correlation between DOC and EC as well as TN and EC. High concentrations of DOC and TN are associated with the highest decomposition stage (V), with the most strongly decomposed wood.
Table 3. Correlation between estimated parameters.
Table 4. Results of multivariate analysis of variance based on the general linear model (GLM) for the dissolved organic carbon (DOC), including the species and decay classes.
Figure 4. Relationships between dissolved organic carbon (DOC, mg · L−1) and electrical conductivity (EC, mS · cm−1).
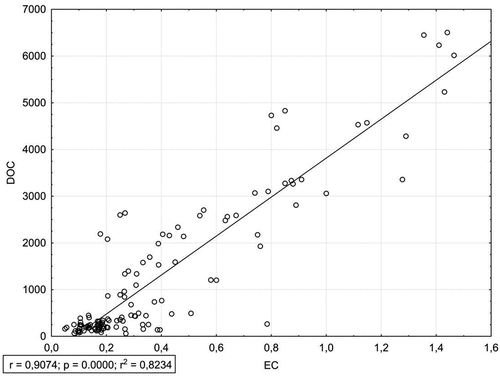
4. Discussion
The concentration of DOC and TN in leachates supplying topsoil horizons released from deadwood depends on the species of wood and its decomposition stage. In the previous works on the release of DOC and TN from deadwood to the soil, such several deciduous and coniferous species in different decomposition stages were not compared concurrently. Of the seven species of wood examined in our study, hornbeam and aspen provide the most DOC and TN to the soil at the time of decomposition, in IV and V decay rate. The obtained concentrations of DOC in leachates from deadwood in III decay class are consistent with the results from other authors (Kahl et al. Citation2012; Kuehne et al. Citation2008). The lowest concentration of DOC and TN in leachates is released from the decomposed spruce wood; 112 mg · L−1 DOC is released from spruce deadwood in the III decay classes. Yavitt and Fahey (Citation1985) recorded DOC concentration of 200 mg · L−1 for coniferous trees in IV decay class at the onset of snow melt. An average of 5452.9 mg · L−1 DOC is released into the soil from the most decomposed aspen wood (decay class V), in the case of hornbeam it is 4697.7 mg · L−1 DOC on average. DOC were released in range from 13,200 to 94,000 mg · kg−1 based on the leaching tests with sawdust obtained from maple, oak, pine, and beech (Svensson et al. Citation2014). According to these authors the particle size of wood plays an important role for DOC leaching. For oak sawdust, the DOC released was 16 times higher compared with oak wood chip. In our study, the high concentration of DOC in leachates from deadwood in V decay class can be explained by its texture and fragmentation. The wood in V decay class is characterized by soft and loose texture which results in greater water capacity and hydrophilicity (Błońska et al. Citation2018). The high DOC and TN concentration can be also associated with the term of the experiment which including snow melt period. According to Yavitt and Fahey (Citation1985) the concentrations of all dissolved constituents were highest at the beginning of the snow melt period. Our study demonstrated statistically significant differences in the concentration of DOC and TN in leachates released between deciduous and coniferous species. Soft and hard wood differs regarding anatomical aspects and unlike from hard wood, soft wood does not present transport vessels (pores) which might affect the leaching patterns (Svensson et al. Citation2014). The observed differences in the concentration of DOC in leachates released between deciduous and coniferous wood be the result of the chemical composition of the wood, lignin fraction, and nutrients content (Wong Citation2009; Kögel-Knabner Citation2002). At the same time, deciduous and coniferous species differ in the quality of hemicellulose, lignin degradation, and presence of resins. According to Weedon et al. (Citation2009) the decomposition of wood depends on many of its characteristics: the content of macronutrients and lignins, and xylem architecture. Differences in the concentration of DOC and TN may be due to differences in the amount of leachate obtained in relation to the throughfall. Lower concentrations of DOC and TN and at the same time lower amounts of leachates were obtained from less decomposed wood. The highest amounts of filtrate were obtained from the most decomposed wood. The amount of obtained leachates is associated with the physical characteristics of wood, especially density, and porosity. As the degree of decomposition increases, wood absorbs water more quickly (Błońska et al. Citation2018).
The lowest concentration of DOC and TN are released in the third decomposition stage irrespective of the species of wood. In the fifth decomposition stage compared to third decomposition stage the concentration of DOC in leachates released by the deciduous species increases more than 10-fold, and 2-fold in the case of coniferous species. Kuehne et al. (Citation2008) reported an increase in DOC and TN release with degradation stage. Hafner et al. (Citation2005) found the DON and NO3 concentrations in leachate from CWD of various decomposition stages higher than in throughfall. The advancement of deadwood decomposition is accompanied by its physical properties change. The third degree of wood decomposition is characterized by the presence of bark, which limits water penetration into the log, restricts the development of organisms. According to Palviainen et al. (Citation2008), the decomposition is slower as a result of bark cover presence. They hypothesized that coarse dead roots within soils may decomposes slower than other types of coarse woody debris due to complete bark coverage and lack of cut surfaces as in logs or stumps. Wood contains substances which are toxic or discouraging wood-destroying organisms. Resistance of toxic substances decreases over time as a result of enzymatic deactivation, self-oxidation, microbiological degradation, or leaching (Harmon et al. Citation1986). The relationships inverse to the above described were noted for the birch wood from which most DOC was released in the third decay classes, and the release was slower with the next stage of decomposition. The birch bark is characterized by high durability, confirming its presence even in the fifth decomposition stage in which the wood is completely decomposed. Birch retains its bark even after extensive decomposition (Harmon et al. Citation2000). The birch bark is different from the bark of other tree species because of the different chemical structure. The presence of suberin in amount up to 38% is characteristic for the birch bark, while the bark of most trees contains only 2% of this substance (Kociołek-Balawejder and Żebrowska Citation2009). The presence of suberin gives the bark durability, the bark is impermeable to water, and provides good thermal insulation. The birch stems decay considerably faster than stems of other tree species. Birch wood is not resistant to decay and it is quickly colonized by white-rot decomposers such as Fomes fomentarius (Mäkinen et al. Citation2006). Released of elements from decaying birch wood in the III and IV decay classes is probably the effect of the presence of undegraded bark which affecting the thermal and moisture conditions. In the V decay class the bark remains undisturbed and the debris of wood is fragmented with a large share of white-rot fungus. The average DOC concentrations runoff from the logs was higher than in throughfall. The results observed in our study correspond to the results observed in past studies (Hafner et al. Citation2005; Bantle et al. Citation2014). Kuehne et al. (Citation2008) noted significantly higher DOC and TN concentrations in the coarse woody debris leachate compared with the throughfall. These authors recorded average TN concentration of 3.6 mg · L−1 for woody debris and 2.6 mg · L−1 for throughfall. The greater DOC concentration for wood in the highest decomposition rate (114.7 mg · L−1) was noted compared to throughfall (60.7 mg · L−1) (Kuehne et al. Citation2008).
In our study, we noted a strong relationship of released DOC with electrical conductivity. The reported relationship reflects the importance of organic ions in EC formation. Monteiro et al. (Citation2013) found strong and positive relationships between EC and DOC concentration in stream water in wet and dry period. A significant positive correlation between DOC and EC was found for the organic horizon by Michalzik and Matzner (Citation1999). Clarke et al. (Citation2005) suggests that the correlation of DOC with EC might be explained by the contribution of organic acids to the total anions.
5. Conclusions
The results confirmed the importance of the wood species and the stage of decomposition on the concentration of DOC and TN in leachates. Significant differences in the concentration of DOC and TN in leachates released between coniferous and deciduous species were noted. Most DOC and TN are released from decomposing hornbeam and aspen wood, the least DOC and TN is released into the soil from coniferous species, especially the spruce. As the stage of decomposition increased, higher DOC and TN concentrations were noted in the leachates from deadwood. The species that deviated from the abovementioned relationships was birch that releases smaller concentration of DOC and TN in leachates compared to other species.
Acknowledgments
The project was financed by the National Science Centre, Poland: decision no. DEC-2016/21/D/NZ9/01333
Additional information
Funding
References
- Baker M, Valett H, Dahm C 2000: Organic carbon supply and metabolism in a shallow groundwater ecosystems. Ecology, 81, 3133–3148. doi:10.1890/0012-9658(2000)081[3133:OCSAMI]2.0.CO;2.
- Bantle A, Borken W, Ellerbrock RH, Schulze ED, Weisser WW, Metzner E 2014: Quantity and quality of dissolved organic carbon released from coarse woody debris of different tree species in the early phase of decomposition. For. Ecol. Manage, 329, 287–294. doi:10.1016/j.foreco.2014.06.035.
- Błońska E, Kacprzyk M, Spólnik A 2017: Effect of deadwood of different tree species in various stages of decomposition on biochemical soil properties and carbon storage. Ecol. Res., 32, 193–203. doi:10.1007/s11284-016-1430-3.
- Błońska E, Klamerus-Iwan A, Łagan S, Lasota J 2018: Changes of the water repellency and physical properties with decomposition rate of deadwood of different species in temperate climate. Ecohydrology, doi:10.1002/eco.2023.
- Clarke N, Røsberg I, Aamlid D 2005: Concentrations of dissolved organic carbon along an altitudinal gradient from Norway spruce forest to the mountain birch/alpine ecotone in Norway. Boreal Environ. Res., 10, 181–189.
- Cocciufa C, Gerth W, Luiselli L, Redolfi de Zan L, Cerretti P, Carpaneto GM 2014: Survey of saproxylic beetle assemblages at different forest plots in central Italy. B. Insectol., 67, 295–306.
- Coomes DA, Allen RB, Sčoty NA, Goulding C, Beets P 2002: Designing systems to monitor carbon stocks in forests and shrublands. For. Ecol. Manage., 164, 89–108. doi:10.1016/S0378-1127(01)00592-8.
- Cooper KJ, Whitaker FF, Anesio AM, Naish M, Reynolds DM, Evans EL 2016: Dissolved organic carbon transformations and microbial community response to variations in recharge waters in a shallow carbonate aqnifer. Biogeochemistry, 129, 215–234. doi:10.1007/s10533-016-0226-4.
- Fravolini G, Egli M, Derungs C, Cherubini P, Ascher-Jenull J, Gómez-Brandón M, Bardelli T, Tognetti R, Lombardi F, Marchetti M 2016: Soil attributes and microclimate are important drivers of initial deadwood decay in sub-alpine Norway spruce forests. Sci. Total Environ., 569–570, 1064–1076. doi:10.1016/j.scitotenv.2016.06.167.
- Hafner SD, Groffman PM, Mitchel MJ 2005: Leaching of dissolved organic carbon, dissolved organic nitrogen, and other solutes from coarse woody debris and litter in a mixed forest in New York State. Biogeochemistry, 74, 257–282. doi:10.1007/s10533-004-4722-6.
- Harmon ME, Franklin JF, Swanson FJ et al. 1986: Ecology of coarse woody debris in temperate ecosystems. Adv. Ecol. Res., 15, 133–302.
- Harmon ME, Krankina ON, Sexton J 2000: Decomposition vectors: a new approach to estimating wood detritus decomposition dynamics. Can. J. For. Res., 30, 76–84. doi:10.1139/x99-187.
- Kahl T, Mund M, Bauhus J, Detlef SE 2012: Dissolved organic carbon from European beech logs: patterns of input to and retention by surface soil. Ecoscience, 19, 1–10. doi:10.2980/19-4-3501.
- Kalbitz K, Kaiser K 2008: Contribution of dissolved organic matter to carbon storage in forest mineral soils. J. Plant Nutr. Soil Sci., 171, 52–60. doi:10.1002/(ISSN)1522-2624.
- Kociołek-Balawejder E, Żebrowska MK 2009: Birch tree biomass – the ways of its practical applications. Prace Naukowe Uniwersytetu Ekonomicznego we Wrocławiu, 57, 252–265.
- Kögel-Knabner I 2002: The macromolecular organic composition of plant and microbial residues as inputs to soil organic matter. Soil Biol. Biochem., 34, 139–162. doi:10.1016/S0038-0717(01)00158-4.
- Kuehne C, Donath C, Müller-Using SI, Bartsch N 2008: Nutrient fluxes via leaching from coarse woody debris in a Fagus sylvatica forest in the Solling Mountains, Germany. Can. J. For. Res., 38, 2405–2413. doi:10.1139/X08-088.
- Lassauce A, Paillet Y, Jactel H, Bouget C 2011: Deadwood as a surrogate for forest biodiversity: meta-analysis of correlations between deadwood volume and species richness of saproxylic organisms. Ecol. Indic., 11, 1027–1039. doi:10.1016/j.ecolind.2011.02.004.
- Liski J, Perruchoud D, Karjalainen T 2002: Increasing carbon stocks in the forest soils of western Europe. For. Ecol. Manage., 169, 159–175. doi:10.1016/S0378-1127(02)00306-7.
- Magnússon RJ, Tietema A, Cornalissen JHC, Hefting MM, Kalbitz K 2016: Tamm review: sequestration of carbon from coarse woody debris in forest soils. For. Ecol. Manage., 377, 1–15. doi:10.1016/j.foreco.2016.06.033.
- Mäkinen H, Hynynen J, Siitonen J, Sievänen R 2006: Predicting the decomposition of Scots pine, Norway spruce and birch stems in Finland. Ecol. Appl., 16, 1865–1879.
- Maser C, Anderson RG, Cromak K, Williams JT, Martin RE 1979: Dead and down woody material. Wildlife Habitats in Managed Forests: the Blue Mountains of Oregon and Washington, Thomas JW, Ed. USDA Forest Service Agricultural Handbook, Vol. 553, 78–95. U. S. Government Printing Office, Washington.
- Michalzik B, Matzner E 1999: Dynamics of dissolved organic nitrogen and carbon in a Central European Norway spruce ecosystem. Europ. J. Soil Sci., 50, 579–590. doi:10.1046/j.1365-2389.1999.00267.x.
- Monteiro MTF, Olivira SM, Luizão FJ, Cândido LA, Ishida FY, Tomasella J 2013: Dissolved organic carbon concentration and its relationship to electrical conductivity in the waters of a stream in a forested Amazonian Blackwater catchment. Plant Ecol. Divers., 7, 205–213. doi:10.1080/17550874.2013.820223.
- Palviainen M, Laiho R, Mäkinen H, Finér L 2008: Do decomposing Scots pine, Norway spruce, and silver birch stems retain nitrogen? Can. J. Forest Res., 38, 3047–3055. doi:10.1139/X08-147.
- Persiani AM, Audisio P, Lunghini D, Maggi O, Granito VM, Biscaccianti AB, Chiavetta U, Marchetti M 2010: Linking taxonomical and functional biodiversity of saproxylic fungi and beetles in broad-leaved forests in southern Italy with varying management histories. Plant Biosyst., 144, 250–261. doi:10.1080/11263500903561114.
- Pichler V, Homolák M, Skierucha W, Pichlerová M, Ramírez D, Gregor J, Jaloviar P 2011: Variability of moisture in coarse woody debris from several ecologically important tree species of the Temperate Zone of Europe. Ecohydrology, 5, 424–434. doi:10.1002/eco.235.
- PN-ISO 10390, 1997. Soil quality. pH determination. PKN (in Polish).
- Russell MB, Fraver S, Aakala T, Gove JH, Woodall CW, D’Amato AW, Ducey MJ 2015: Quantifying carbon stores and decomposition in dead wood: a review. For. Ecol. Manage., 350, 107–128. doi:10.1016/j.foreco.2015.04.033.
- Spears JDH, Holub SM, Harmon ME, Lajtha K 2003: The influence of decomposing logs on soil biology and nutrient cycling in an old-growth mixed coniferous forest in Oregon, USA. Can. J. Forest Res., 33, 2193–2201. doi:10.1139/x03-148.
- Strachel R, Wyszkowska J, Baćmaga M 2017: The influence of nitrogen on the biological properties of soil contamined with zonc. Bull. Environ. Contam. Toxicol., 98, 426–432. doi:10.1007/s00128-016-1977-2.
- Svensson H, Marques M, Kaczala F, Hogland W 2014: Leaching patterns from wood of different tree species and environment al implications related to wood storage areas. Water Environ. J., 28, 277–284. doi:10.1111/wej.2014.28.issue-2.
- Weedon JT, Cornwell WK, Cornelissen JHC, Zanne AE, With C, Coomes DA 2009: Global meta-analysis of wood decomposition rates: a role for trait variation among tree species? Ecol. Lett., 12, 45–56. doi:10.1111/j.1461-0248.2008.01259.x.
- Weiss P, Schieler K, Schadauer K, Radunsky K, Englisch M 2000: Carbon Budget of Austrian Forests and Considerations about Kyoto Protocol. Monographie 106. Federal Environment Agency, Wien (in German).
- Wong DWS 2009: Structure and action mechanism of ligninolytic enzymes. Appl. Biochem. Biotechnol., 157, 174–209. doi:10.1007/s12010-008-8287-z.
- WRB (World Reference Base For Soil Resource), 2014. FAO, ISRIC and ISSS.
- Yan G, Xing Y, Xu L, Wang J, Dong X, Shan W, Guo L, Wang Q 2017: Effects of different nitrogen additions on soil microbial communities in different seasons in a boreal forest. Ecosphere, 8 7, e01879. doi:10.1002/ecs2.1879.
- Yang FF, Li YL, Zhou GY, Wenigmann KO, Zhang DQ, Wenigmann M, Liu SZ, Zhang QM 2010: Dynamics of coarse woody debris and decomposition rates in an old-growth forest in lower tropical China. For. Ecol. Manage., 259, 1666–1672. doi:10.1016/j.foreco.2010.01.046.
- Yavitt JB, Fahey TJ 1985: Chemical composition of interstitial water in decaying lodgepole pine bole wood. Can. J. For. Res., 15, 1149–1153. doi:10.1139/x85-186.
- Yin X 1999: The decay of forest woody debris: numerical modeling and implications based on some 300 data cases from North America. Oecologia, 121, 81–98. doi:10.1007/s004420050909.