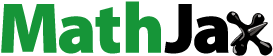
ABSTRACT
The influence of the long-term combination of rice straw removal and rice straw compost application on methane (CH4) and nitrous oxide (N2O) emissions and soil carbon accumulation in rice paddy fields was clarified. In each of the initial and continuous application fields (3 and 39−51 years, respectively), three plots with different applications of organic matter were established, namely, rice straw application (RS), rice straw compost application (SC) and no application (NA) plots, and soil carbon storage (0−15 cm), rice grain yield and CH4 and N2O fluxes were measured for three years. The soil carbon sequestration rate by the organic matter application was higher in the SC plot than in the RS plot for both the initial and continuous application fields, and it was lower in the continuous application field than in the initial application field. The rice grain yield in the SC plot was significantly higher than those in the other plots in both the initial and continuous application fields. Cumulative CH4 emissions followed the order of the NA plot < the SC plot < the RS plot for both the initial and continuous application fields. The effect of the organic matter application on the N2O emissions was not clear. In both the initial and continuous application fields, the increase in CH4 emission by the rice straw application exceeded the soil carbon sequestration rate, and the change in the net greenhouse gas (GHG) balance calculated by the difference between them was a positive, indicating a net increase in the GHG emissions. However, the change in the GHG balance by the rice straw compost application showed negative (mitigating GHG emissions) for the initial application field, whereas it showed positive for the continuous application field. Although the mitigation effect on the GHG emissions by the combination of the rice straw removal and rice straw compost application was reduced by 21% after 39 years long-term application, it is suggested that the combination treatment is a sustainable management that can mitigate GHG emissions and improve crop productivity.
1. Introduction
Recently, the importance of carbon sequestration in agricultural soils has been recognized from the viewpoint of climate change and maintenance of crop productivity. For example, to mitigate the climate change caused by an increase in atmospheric carbon dioxide (CO2), “4 ‰ initiative”, targeting a 0.4% year−1 increase in soil carbon storage has been launched (Minasny et al. Citation2017). Generally, carbon sequestration (i.e., organic matter accumulation) in agricultural soils leads to the maintenance of soil fertility and crop productivity. Maintaining the soil organic matter is important especially in rice paddy fields, because the nitrogen uptake of the rice plants derived from the soil is high (over 50% of the total plant nitrogen uptake, Shoji and Mae Citation1984).
To accumulate carbon in agricultural soil, there are ways to reduce its loss or increase its input (Lal Citation2004). As a method to reduce carbon loss, soil organic matter decomposition can be suppressed by reduced tillage (e.g., Higashi et al. Citation2014 [upland]; Kim et al. Citation2016 [paddy]). To increase the carbon input, organic matter, such as crop residues and compost (Koga and Tsuji Citation2009 [upland]; Sumida et al. Citation2005 [paddy]) and green manure (Higashi et al. Citation2014 [upland]), can be applied. In particular, since paddy soils are under anaerobic conditions due to flooding during the cultivation period, soil carbon tends to accumulate in greater amounts than in upland soils (Mitsuchi Citation1974).
The application of organic matter to paddy fields promotes the emission of methane (CH4), which is a potent greenhouse gas (GHG); for example, crop residues, such as rice straw and wheat straw (Li et al. Citation2014; Yagi and Minami Citation1990), compost (Kumagai et al. Citation2010), and green manure (Lee et al. Citation2010). Therefore, it is necessary to pay attention to the trade-off between soil carbon accumulation (i.e., CO2 sequestration) and CH4 due to the application of organic matter. The amount of CH4 emissions from paddy fields varies greatly depending on the type, source and amount of the organic matter applied, and the managements in the fields (Cheng et al. Citation2018; Sander et al. Citation2018; Tirol-Padre et al. Citation2018; Toma et al. Citation2016). The incorporation of rice straw in the paddy soil causes large CH4 emissions during the rice cultivation period, but the CH4 emissions are significantly reduced by composting the straw and applying it to the soil (Yagi and Minami Citation1990). It has also been reported that CH4 emissions differ depending on whether livestock manure species are used as the compost material (Kumagai et al. Citation2010). It has also been reported that there is a significant positive correlation between acid detergent-soluble organic matter (indicator of readily decomposable organic matter) and the CH4 emission potential in various organic materials, including rice straw (Nagumo et al. Citation2016). It has been reported that the CH4 emissions from rice paddy fields increases in parallel with the application rate of organic matter in rice straw (Naser et al. Citation2007; Watanabe et al. Citation1995) and green manure (Lee et al. Citation2010). The influence of organic matter application on N2O emissions varies, and the suppression of the N2O emissions due to the application of rice straw has also been reported (e.g., Li et al. Citation2014). Most of these results were obtained in short-term experiments (several years), and almost no consideration was given to the effects of long-term application. In addition, although there have been examples evaluating the effect of the application of organic matter (livestock manure compost) to upland fields on long-term soil carbon accumulation and N2O emissions (Koga Citation2017), no similar reports in paddy fields have been found. In addition, if the application of organic matter is continued for a long time, the soil carbon accumulation rate is thought to become lower than at the beginning of application (e.g., Shiga et al. Citation1985; Yokozawa et al. Citation2010), and as a result, it is considered that the balance between the soil carbon accumulation and the increase in the CH4 emissions due to organic matter could be changed.
Therefore, in this study, the influence of the long-term combination of the rice straw removal and rice straw compost application on CH4 and N2O emissions, soil carbon accumulation and GHG balance integrating them were clarified in rice paddy fields with the long-term application of organic matter.
2. Materials and methods
2.1. Study site
The experiment was conducted at the rice paddy fields of the Hokuriku Research Station, Central Region Agricultural Research Center, National Agriculture and Food Research Organization (NARO) (37°07′Ν, 138°16′Ε), located at Niigata Prefecture, Japan for three years (2010 to 2012). The soils of the study fields were classified as gley lowland soil (Typic Hydraquents in the USDA Soil Taxonomy (Soil Survey Staff Citation2010) and Gleyic Fluvisol in the World Reference Base (FAO Citation2006)). The main soil physico-chemical properties of the studied fields are shown in .
Table 1. Main soil physicochemical properties of the studied fields (0–15 cm, April 2010).
The mean annual temperature and precipitation in this region (obtained from the automated meteorological data acquisition system [AMeDAS] at Takada) were 13.6°C and 2,755 mm, respectively. The average air temperatures during the cultivation period (May–September) in 2010–2012 were higher than the 30-year average during the study period, and the difference was particularly obvious during July to September (). The air temperatures in August 2010 and September 2012 were 2.4 and 2.5°C higher than the 30-year average, respectively. Compared to the 30-year average, precipitation during the growing season in 2010 and 2012 was lower, while those in 2011 were higher. In 2010, the monthly precipitations of July and August were lower than the average. However, in 2012, the monthly precipitations were lower than the average during May to August.
Table 2. Monthly air temperature and precipitation during the rice growing seasons.
2.2. History of the organic matter application treatment and plant cultivation
Two sets of paddy fields with different organic matter application histories (i.e., initial application or continuous application) were used in this study. Each set consisted of three treatments: rice straw application, rice straw compost application and no application.
In the continuous (long-term) application fields, treatments of the rice straw application (C-RS), rice straw compost application (C-SC) and no application (C-NA) had been continued for 32, 39 and 51 years at the start of this study in 2010. The area of each treatment plot was 150 m2 without field replication (). All the rice grains and straw were removed from all of the plots at the time of the harvest. In the C-RS, 600 g m−2 (= 6.0 Mg ha−1, fresh weight) of the rice straw cut into pieces 5-cm in length was scattered on the soil surface of the plot after the autumn harvest and incorporated into the soil by plowing during the following spring (). In the C-SC, 1.5 kg m−2 (= 15 Mg ha−1, fresh weight) of rice straw compost, which was produced in the research station without any addition of nitrogen, was applied to the plot and incorporated into the soil by plowing in the spring. The chemical properties of the rice straw and rice straw compost applied are shown in . Assuming that the amount of SiO2 in the rice straw was not lost throughout the compost production process, the yield of the compost production from the rice straw (i.e., conversion factor) was estimated as 60 and 31% for fresh and dry weight basis, respectively. The yield was relatively lower than the value (40% for dry weight basis) reported by Cheng et al. (Citation2016). In total, six rice (Oryza sativa L.) cultivars were planted in the fields for 1959−1990 (2–10 years for each cultivar), and the cultivar ‘Dontokoi’ was planted for 1991-present (until 2012).
Table 3. Date of cultivation management.
Table 4. Physicochemical properties of rice straw and rice straw compost and estimated yield of rice straw compost production (3-year average).
Figure 1. Layout of the study fields. NA, no application; RS, rice straw, and SC, rice straw compost.
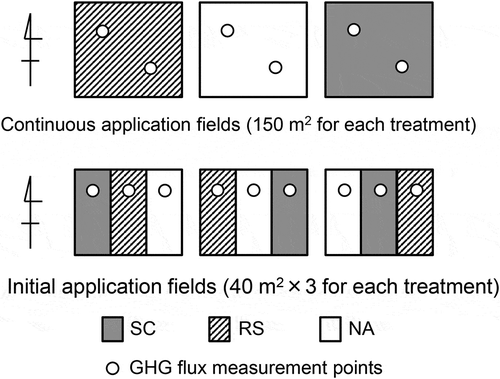
Three fields with continuous paddy rice cultivation without organic matter application were used as the initial application fields, and each field was divided into three plots (i.e., I-RS, I-SC and I-NA) for replication (40 m2 × 3 for each treatment) (). The organic matter application treatments were conducted from the spring of 2010 in the same manner as the continuous fields (i.e., rice straw application [I-RS], rice straw compost application [I-SC] and no application [I-NA]), with the exception of the application of rice straw in 2010, which had been kept in the warehouse during the winter and applied to the plot just before the spring plowing (middle of April).
Detailed information about the rice cultivation management is shown in . The same amounts of fertilizer (N-P2O5-K2O) as the basal application (4-7-7 g m−2) and top-dressing (1.5-0-0 g m−2) were applied to all the fields. The average plant density of the rice was 19.2 hills m−2. Chemical control of diseases and pests was conducted according to local conventions. Mid-season drainage was mainly conducted during late June to the middle of July. Intermittent drainage (saturated irrigation) was carried out after the mid-season drainage. Subsequently, the final drainage was carried out in the end of August or the beginning of September. The harvest was carried out in the middle of September.
2.3. Sampling and analysis of the soil and the calculation of the soil carbon sequestration rate
Bulk and intact soil samples were taken at a depth of 0–15 cm from all the plots before the initiation of the study (April 2010) to determine the carbon storage in the soil. In the initial application fields, the bulk soil samples were also taken twice a year (in April and October) throughout the study period. After the bulk soil samples were air-dried, sieved (2-mm mesh), and finely ground, the total carbon and nitrogen contents were measured using an N/C analyzer (JM3000CN, J-SCIENCE LAB. CO. Ltd., Kyoto, Japan). Intact soil samples were taken from the center of the layer (0–15 cm) using a 100-cm3 core sampler with three replicates for the bulk density measurement. In the initial application fields, the rates of the soil carbon sequestration derived from the organic matter application (0–15 cm) were calculated by the temporal changes in the soil carbon content over the study period. For the calculation, the averaged bulk density across the treatments (0.882 Mg m−3) was used ().
To eliminate the effect of the continuous application of the organic matter on the soil bulk density, soil carbon storage (0–15 cm) in the continuous application fields was calculated on a soil mass basis (Koga et al. Citation2006; Paustian et al. Citation1997). Soil depths of C-RS and C-SC were adjusted to make that their dry soil mass was equal to that of the C-NA (0–15 cm). The common soil mass was used to calculate the soil carbon storage in all the treatments. Assuming that the initial value of the soil carbon storage at the start of the organic matter application was similar between the application and no application plots, the rates of soil carbon sequestration derived from the organic matter application in the C-RS and C-SC were calculated by the difference in the soil carbon storage with the C-NA (EquationEquation 1(1)
(1) ; for more details, see Fig. S1).
2.4. Rice yield
At the harvest stage (late September), 64 rice hills were harvested from each plot to measure the yield. After air-drying, threshing, and wind selection, the unhulled rice was hulled and sieved (1.9-mm mesh). The weight of the hulled rice (i.e., brown rice yield) was converted to that with 15% moisture content.
2.5. Gas flux measurement
CH4 and N2O fluxes were measured during the rice-growing period using a closed-chamber method described by Takakai et al. (Citation2017a). Rectangular transparent acrylic chambers (30 × 65 × 60 or 120 cm in length × width × height) were used for the measurements. During the measurement, the chamber included four hills of rice plants.
In the continuous application fields, the flux measurement was conducted with two replicates per field (), while in the initial application fields, the flux measurement was conducted with three replicates (i.e., one measurement point per plot). Gas samples were taken at 1, 11 and 21 min after the chamber was closed. Measurements were conducted almost once a week during the growing period except for the N2O fluxes after the mid-season drainage in 2012 (thinning of the N2O concentration analysis). In 2011 and 2012, the soil redox potential (Eh) was measured at a depth of 5 cm using platinum-tipped electrodes (three replicates per measurement point) and a portable Eh meter (PRN-41, Fujiwara Scientific Company Co. Ltd., Tokyo, Japan) simultaneously with each measurement of the gas fluxes. To avoid any disturbance, the flux measurements were conducted from boardwalks constructed in the fields.
The CH4 and N2O concentrations were analyzed using a gas chromatograph equipped with a flame ionization detector and an electron capture detector, respectively (GC-14B, Shimadzu, Kyoto, Japan). CH4 and N2O fluxes were calculated using a linear regression method. The cumulative emissions of CH4 and N2O during the measurement period were calculated by integrating the fluxes using linear interpolation.
2.6. Evaluation of the effect of organic matter application on net greenhouse gas balance
To evaluate the net GHG balance including the soil carbon sequestration rate due to the application of organic matter, CH4 and N2O emissions were converted into the CO2 equivalent using the global warming potentials (34 and 298 for CH4 and N2O, respectively, IPCC Citation2013).
In the initial and continuous application fields, the changes in the net GHG balances by the organic matter application in RS and SC plots were calculated based on the difference with the corresponding NA plot.
The yield-scaled net GHG balance was calculated by dividing the net GHG balance by the grain yield (brown rice).
2.7. Statistical analysis
Differences in the cumulative CH4 and N2O emissions among the plots in the initial application fields were compared using a Tukey’s HSD test for each year. Differences in the cumulative CH4 and N2O emissions and rice yields among the plots were compared by two-way analysis of variance (ANOVA, Year × Plot) followed by a Tukey’s HSD test for three years. In this study, differences with p < 0.10 were considered significant. For all the statistical analyses, Excel statistics 2012 for Windows (SSRI, Tokyo, Japan) was used.
3. Results
3.1. Soil carbon sequestration rate
Throughout the three-year experimental period, the soil carbon content in the initial field tended to increase in both the organic matter application plots (). In particular, the increase in the soil carbon content of I-SC was high, and its relation with the date was significant (p < 0.01). Although the soil carbon content in the I-NA increased slightly every year, in the calculation of the soil carbon sequestration rate, the soil carbon content was considered to have not changed due to the high p value (0.58). Therefore, the increase rates of carbon content in both the organic matter application plots were taken as the soil carbon sequestration rates derived from the organic matter application, and the rate in the I-SC was approximately 2.5 times higher than in the I-RS (). In the continuous application field, the bulk density decreased, and the soil carbon contents increased compared to the no application plot (C-NA) due to the long-term continuous application of rice straw (32 years) and rice straw compost (39 years) (). The soil carbon sequestration rate by the organic matter application in the C-SC plot was approximately two times higher than that in the C-RS plot. The soil carbon sequestration rate by the organic matter application in the continuous application field was lower than that in the initial application field () (31% and 29% for RS and SC, respectively).
Table 5. Soil carbon (C) sequestration rate in the initial application field.
Table 6. Soil carbon (C) sequestration rate in the continuous application field.
3.2. Rice yields
The grain yield of the rice (3-year average) in the SC plot was significantly higher than that in the other plots in both the initial and continuous application fields (). This was mainly due to increased number of panicles and number of spikelets per panicle (Table S1).
Table 7. Averaged grain yield over three years.
3.3. CH4 and N2O emissions
In 2010, the range of CH4 flux before and during the mid-season drainage was similar with that during the re-flooded period after the mid-season drainage in all the plots, and a large increase in the CH4 emissions by the rice straw application was found in the earlier growing period before the mid-season drainage (). It is thought that the large CH4 emissions in the rice straw plot in the initial application field was caused by the application of undecomposed rice straw due to storage in the warehouse in the spring (Watanabe et al. Citation1993). Simultaneously, in 2011 and 2012, the Eh decreased during the re-flooded period after the mid-season drainage, and the CH4 flux rose greatly compared with before the mid-season drainage. This tendency was remarkable particularly in the continuous application field. The N2O flux remained mostly near zero throughout the measurement period for three years, but episodic emissions were observed before and after the final drainage in the continuous field ().
Figure 3. Seasonal variations in the soil Eh at the 5 cm depth (a), CH4 flux (b) and N2O (c). Bars for the CH4 and N2O fluxes indicate standard errors. C, continuous application; I, initial application; NA, no application; RS, rice straw; SC, rice straw compost. FD, final drainage; H, harvesting; MD, mid-season drainage; T, transplanting.
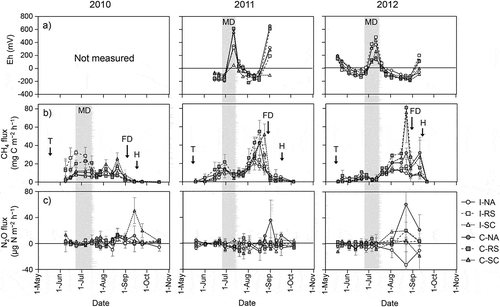
The cumulative CH4 emissions followed the order of the NA plot < the SC plot < the RS plot with the exception of the continuous application field in 2011 (). Similarly, in the 3-year average (excluding the I-RS plots in 2010 influenced by the application of rice straw in spring), the cumulative CH4 emissions followed the order of the NA plot < the SC plot < the RS plot in both the initial and continuous application fields, and those in the RS plot were significantly higher than those in the other plots. In this experiment, organic matter was applied to the initial application field continuously for three years, and an analysis of variance is performed with the year as a factor. Therefore, there is a possibility that a ‘carry-over effect’ on CH4 emissions due to organic matter accumulation in the soil may occur. However, the carry-over effect was not clear, as the CH4 emissions in both the organic matter application plots did not increase yearly. Throughout the three-year experimental period, there was a tendency for the CH4 emissions to be higher in the continuous application field than in the initial application field. In the initial application field, the cumulative N2O emissions followed in the order of the NA plot < the RS plot < the SC plot on average for three years, and a significant difference was observed between the NA and SC plots. In the continuous application field, the cumulative N2O emissions in the NA plot tended to be higher than those in the other plots, but there was no significant difference among the plots.
Table 8. Cumulative methane (CH4) and nitrous oxide (N2O) emissions for three years.
3.4. Net greenhouse gas balance
In terms of CO2-equivalent, the CH4 emissions were more than two orders of magnitude higher than the N2O emissions (). In both the initial and continuous application fields, the net GHG balance in the RS plot was higher compared to the NA plot because the increment in CH4 emission by the rice straw application exceeded the soil carbon sequestration rate, indicating a net increase in GHG emissions. The net increase in the GHG emissions due to the application of the rice straw in the continuous field was larger than that in the initial application field, mainly due to both the greater increase in the CH4 emissions and the lower soil carbon sequestration rate. The change in the net GHG balance by the application of the rice straw compost showed a negative value (mitigation of net GHG emissions) for the initial application fields, while the change in the continuous application field showed a positive value, resulting from both the decline in the carbon sequestration rate and the increase in CH4 emission due to the long-term application. Although the mitigation effect on the net GHG emissions by the combination of the rice straw removal and rice straw compost application in the continuous application field (−3.72 Mg CO2-eq ha−1 y−1) was lower than that in the initial application field (−4.71 Mg CO2-eq ha−1 y−1), it remained approximately 79% of that in the initial application field.
Table 9. Changes in net greenhouse gas (GHG) balance due to the application of organic matter.
The yield-scaled net GHG balances followed the order of the SC plot < the NA plot < the RS plot in both the initial and continuous application fields.
4. Discussion
4.1. Soil carbon sequestration rate
In both the initial and continuous application fields, the soil carbon sequestration rate by the rice straw compost application was higher than that by the rice straw application (, , ). It is considered that the rate of carbon sequestration by the application of organic matter is regulated by the amount and the quality of the organic matter applied. In this study, the carbon application rate by the application of the rice straw (1.43 Mg C ha−1 y−1) exceeded that of the rice straw compost (1.29 Mg C ha−1 y−1) by calculations derived from their application rates and carbon contents (). Therefore, the difference in the carbon sequestration rate was thought to be mainly due to the difference in the quality of the organic matter applied. Shiga et al. (Citation1985) evaluated the decomposition characteristics of the organic matter applied to the paddy soils by a glass fiber-filter paper bag method and reported that the decomposition rate of the rice straw compost is lower than that of the rice straw, and the amount of the soil carbon accumulated due to the continuous application of the rice straw compost was large. In addition, they also reported that the carbon decomposition rate of the organic matter applied was regulated by the lignin content. Similarly, Miura (Citation2003) reported that the easily decomposable carbon (including cellulose and hemicellulose, which is a substrate of CH4) decreased in the process of composting the rice straw, while the recalcitrant fraction, including lignin, increased. From the calculations described above, the rice straw compost high in recalcitrant organic matter was thought to have a higher soil carbon sequestration rate compared to the rice straw.
Soil carbon sequestration by organic matter application declined due to long-term continuous application (, ). Continuing the application of the organic matter, the soil carbon sequestration rate could decrease yearly, and the soil carbon storage is thought to approach the equilibrium state. Yokozawa et al. (Citation2010) applied the Roth C model to agricultural soil throughout Japan and estimated that the soil carbon sequestration rate by manure application to paddy fields (1.0 Mg C ha−1 y−1) decreased in the order of 1 year (0.62 Mg C ha−1 y−1) > 10 years average (0.43 Mg C ha−1 y−1) > 25 years average (0.31 Mg C ha−1 y−1). The rate in this study was relatively higher than this. Because the study site was a paddy field with poor drainage located in a cool-temperate region, the decomposition of applied organic matter may be slow. Yokozawa et al. (Citation2010) evaluated the soil carbon storage at a depth of 0−30 cm. Although this study evaluated only the plowed layer at a depth of 0−15 cm, it is known that carbon and nitrogen could also accumulate in the subsoil due to the application of organic matter, such as compost (e.g., Sumida et al. Citation2002; Takakai et al. Citation2017b, Citation2017c). Therefore, in this study, there was a possibility of underestimating the soil carbon sequestration rate by the organic matter application.
4.2. Rice yield and soil nitrogen
Cheng et al. (Citation2016) reported that the C/N ratio in the soil is nearly constant (on average 13.49) regardless of the carbon content in a long-term application experiment with different organic matter management. This indicates that the application of organic matter to the paddy soils can simultaneously accumulate not only carbon but nitrogen. The rice yield in the rice straw compost plot tended to be higher than that in the no application plot, and the increase was larger in the continuous application field than in the initial field (). It is considered to be due not only to the nitrogen supply from the compost applied in the current year but also due to an increase in the nitrogen supply from the organic matter accumulated in the soil (). Ohyama (Citation1982) indicated that the nitrogen supply from the compost (nitrogen uptake of the rice plant) was low at the initial application, and it increased yearly from an analysis of a long-term continuous application experiment of rice straw compost. In addition, Yasuda and Okada (Citation1998) reported that the nitrogen supply by the continuous application of the rice straw compost (1.0 kg m−2 y−1) reached its maximum at 13 years for continuous application by analyzing the same data shown in Ohyama (Citation1982). Similarly, in this study, the application of the rice straw compost to the continuous application field had been continuing for approximately 40 years, and the increase in the rice yield by the compost application was considered to be larger than that in the initial application field.
The effect of the application of rice straw on the rice yield was not clear for both the initial and continuous application fields (). The accumulation of the soil nitrogen by the continuous application of the rice straw was confirmed, but the increase was small compared to the rice straw compost (). In addition, the rice straw application to the paddy fields with poor drainage in a cool-temperate region could promote the abnormal reduction of the soil and the immobilization of nitrogen, which could have a negative influence on the growth of rice (Kubota Citation1992). Although abnormal reduction (i.e., large decrease in soil Eh) was not confirmed in this study (), it was hypothesized that the increase in the rice yield by the rice straw application was lower than that by the rice straw compost due to the combination of the factors described above.
4.3. CH4 and N2O emissions
The CH4 flux tended to be high in the late growing period after the mid-season drainage () with the exception of the rice straw plot in the initial application field in the first year (application in the spring). Typically, the CH4 emissions derived from rice straw are higher in the early growing period (Watanabe et al. Citation1999), and it has been thoroughly reported that the CH4 emissions from the rice straw application plots tend to be remarkably higher than those in the no application plots in the early growing period (Shiono et al. Citation2014; Takakai et al. Citation2017a; Yagi and Minami Citation1990). Although the reason why the CH4 emissions in the early growing period from all the plots, including the rice straw application plot, was low in this study is not clear, there is a possibility that high soil-free ferric iron content may have an effect. CH4 production in paddy soil is controlled by the balance between the reduction capacity (readily decomposable organic matter) and the oxidation capacity (iron and manganese oxides) (Inubushi et al. Citation1984, Citation2018). The soil ferric iron contents in this study (15.7 g Fe kg−1) were high compared to the case of the CH4 flux measurement in the same area (Niigata: 8−9 g Fe kg−1 (Shiratori et al. Citation2007) and Yamagata: 10.1 g Fe kg−1 (Shiono et al. Citation2014)) and different types of paddy soils collected around Japan for evaluating CH4 production by an incubation experiment (2.7−10.6 g Fe kg−1, Cheng et al. Citation2007), suggesting the possibility that the soil reduction and CH4 production after flooding were suppressed. Alternatively, for the high CH4 flux in the late growing period, the soil clay content in the study site was high, resulting in low field drainage; thus, the soil remained reductive in the late growing period after the mid-season drainage, and the CH4 production activity was considered to be active in the summer with high soil temperature. In the first year (2010), rainfall was small in July and August (). It is not certain because the soil Eh was not measured, but there was a possibility that the reduction of the soil did not proceed compared to the other two years, and the CH4 emissions were suppressed.
In both the initial and continuous application fields, the CH4 emissions from the rice straw plot were higher than those from the rice straw compost plot (; ). This finding was consistent with previous studies (e.g., Miura Citation2003; Yagi and Minami Citation1990). The higher CH4 emissions could be due to the decrease in cellulose and hemicellulose, which are substrates of CH4, during the rice straw composting process (Miura Citation2003). The reduction rate of the CH4 emissions when changing the applied organic matter from rice straw to the rice straw compost (initial: 19%, continuous: 16%) was lower than those in the previous study (40 to 61%, Yagi and Minami Citation1990). This could be mainly due to a small increase in the CH4 emissions due to the application of rice straw (compared to the no application plot, initial: 40%, continuous: 35%). An increase in the CH4 emissions due to the application of rice straw to paddy fields has been reported at various locations in Japan; 172−229% in Ibaraki (Yagi and Minami Citation1990), 289% in Fukushima (Miura Citation2003), 641−1191% in Yamagata (Shiono et al. Citation2014), and 113% in Akita (Takakai et al. Citation2017a). Katayanagi et al. (Citation2016) evaluated the relationship between the amount of organic matter applied (Manure + Residue) and the CH4 emissions using a meta-analysis of CH4 emission data from the paddy fields in various locations in Japan. They subsequently reported that the slope (emitted CH4/applied manure and residue [on carbon basis]) was 0.12−0.23 in the Tohoku region, including the study site, and 0.14−0.27 in the Hokuriku region. The slope in this study (calculated from [CH4 emissions from the organic matter application plot − CH4 emissions from the no application plot]/amount of applied organic matter) was 0.02−0.08, and the response of CH4 emissions to the application of organic matter was lower than the study. Though the reason is not clear, this difference is thought to be related to the low CH4 flux in the early growing period as described above.
In the continuous application field, the CH4 flux in the rice straw and rice straw compost plots tended to be higher than that from the no application plot in the late growing period after mid-season drainage (). Increased CH4 emissions in the continuous application field are considered to be attributed to two factors: the supply of substrate from the organic matter applied in the current year and the supply of substrate from the soil carbon accumulated by previous continuous application (i.e., continuous application effect). Cheng et al. (Citation2016) reported that the carbon content and the carbon mineralization potential of the soil increased due to the long-term continuous application of organic matter (rice straw and rice straw compost) to the paddy soils. However, Watanabe et al. (Citation1999) indicated that the proportion of the CH4 emissions derived from the soil carbon to the total CH4 emissions from the paddy fields was almost constant throughout the growing period. In addition, although the soil carbon storage was higher in the rice compost plot than that in the rice straw plot (), the tendency of the CH4 emissions was the opposite: higher in the rice straw plot than in the rice straw compost plot. Based on these results, the increase in CH4 emissions due to the continuous application of organic matter cannot be explained only by the accumulation of soil carbon, and the possibilities of the influence of organic matter applied in the current year and the difference in the decomposability of the carbon accumulated in the soil (Cheng et al. Citation2016) were considered. The promotion of rice growth was also considered to be an indirect cause of the increase in CH4 emissions due to the long-term application of organic matter. Root exudates and rice debris are important substrates for CH4 emissions in the late growth period (Watanabe et al. Citation1999). Because the rice yield in the rice straw compost plot of the continuous application field was high (), it is expected that its biomass was also high throughout the growing period. Therefore, the increase in the supply of the substrate and the subsequent CH4 emissions in the late growing period could be considered. To distinguish and analyze the influence of these two factors (effect of the organic matter applied in the current year or continuous application effect), it is considered necessary to establish a new no application plot in the continuous organic matter application field (i.e., a plot with interruption of organic matter application) to compare with the continuous organic matter application plot. However, it is considered to be difficult to conduct such an analysis due to restrictions, such as the field area and cultivation management, in most of the long-term organic matter application experiment fields, including the site in this study.
The CH4 emissions from the continuous application fields, including the no application plot, were higher than those from the initial application fields (). Although the reason is not clear, the soil carbon content in the no application plot of the continuous application field was higher than the initial value of the initial application field, and the possibility that this could be due to the influence of the field management before this study was considered.
The enhancement of N2O emissions by the application of organic matter was not observed in both the initial and continuous application fields (). The soil nitrogen accumulated due to the long-term continuous application of organic matter, in particular the rice straw compost (), but the effect on N2O emissions was not clear. The cumulative N2O emissions (−176 to 346 g N ha−1) were comparable to those of Shiono et al. (Citation2014) (−318 to 255 g N ha−1) and were lower than the average value (667 g N ha−1) of the N2O emissions from the Japanese paddy fields summarized by Akiyama et al. (Citation2005). When evaluated as the CO2-equivalent, the proportion of N2O emissions to CH4 emissions was as small as 1% or less (), which was comparable to that reported by Shiono et al. (Citation2014).
4.4. Net greenhouse gas balance
In both the initial and continuous application fields, the increase in CH4 by the application of rice straw exceeded the soil carbon sequestration rate, indicating a net increase in the GHG emissions (). Alternatively, in the initial application field, the application of the rice straw compost has a high soil carbon sequestration rate, indicating a net mitigation of the GHG emissions. This agreed with the estimation of a trade-off relationship between the soil carbon accumulation and the increase in the CH4 and N2O emissions due to the application of the livestock manure compost (Yokozawa et al. Citation2010). In addition, the soil carbon sequestration rate decreased, and the mitigation effect of the GHG emissions turned into a promotion effect due to the long-term continuous application of the rice straw compost. Although the mitigation effect on the GHG emissions by the combination of the rice straw removal and rice straw compost application declined by continuing the treatment for the long-term, it was hypothesized that the treatment is effective even if it continued for approximately 40 years. Besides, the rice yield in the rice straw compost application plot was significantly higher than those in other two plots, and the yield-scaled net GHG balance was lower (, ). Consequently, it is considered that the rice straw removal and rice straw compost application is a sustainable management that can balance crop production and mitigating GHG emissions.
In this study, the GHG balance in the rice straw and the rice straw compost plots were compared simply in the same area (, ). However, to produce rice straw compost with a general application rate to the field, it is necessary to collect rice straw from a field with a larger area than the applied field. In this study, considering the yield of compost production (), the rice straw collected from the field of 4.14 ha is needed to produce the rice straw compost to be applied to a field of 1 ha (EquationEquation 2(2)
(2) ).
where AreaRS and AreaSC are the areas of rice straw collection (4.14 ha) and rice straw compost application (1.00 ha) respectively. AppSC and AppRS are the application rates of rice straw compost (15 Mg ha−1) and rice straw (6.0 Mg ha−1), respectively. Yield is the yield of the compost production from the rice straw (60% for fresh weight basis, ).
It is necessary to evaluate the influence on the GHG balance, including the influence of the rice straw collection (i.e., removal) from the entire field. In addition, CH4 and N2O are also emitted during the process of composting rice straw (Miura Citation2003). Taking these factors into consideration, the GHG balance was evaluated when the rice straw was removed from the field and composted and applied to the field (EquationEquation 3(3)
(3) ; ).
Figure 4. Effects of rice straw removal and compost application on net greenhouse gas balance (CO2 equivalent) evaluated by taking compost production into consideration. Positive values indicate net emissions to the atmosphere, and negative values indicate net uptake from the atmosphere. To produce 15 Mg of rice straw compost applied to 1 ha of paddy field, 24.83 Mg of rice straw was estimated to be collected from 4.14 ha (calculated from the by assuming negligible SiO2 loss during the composting process with the rice straw application rate of 6.0 Mg ha−1). Changes in CH4 and the soil carbon due to the rice straw removal (4.14 ha) and rice straw compost application (1.00 ha) were estimated from . CH4 emissions during composting were calculated using a factor estimated by Miura (Citation2003). N2O emissions during compositing were excluded from the evaluation because it was not observed with no nitrogen addition (Miura Citation2003). Changes in N2O emissions by the organic matter application were excluded from the evaluation because the values were very small.
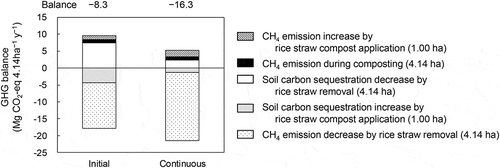
where SoilCRS and SoilCSC are the soil carbon sequestration rate by the application of rice straw and rice straw compost, respectively and CH4NA, CH4RS and CH4SC are the CH4 emissions from the NA, RS and SC plots, respectively (Mg CO2-eq ha−1 y−1, from ). FCOMP is the conversion factor for CH4 emission during composting (43 g CO2-eq kg rice straw−1) estimated by Miura (Citation2003) with the GWP of CO2:CH4 = 1:34 (IPCC Citation2013). N2O emissions during compositing were excluded from the evaluation because it was not observed with no nitrogen addition, while nitrogen addition can increase N2O emissions during composting (Miura Citation2003). Changes in N2O emissions by the organic matter application were excluded from the evaluation because the values were very small.
In both the initial and continuous application fields, the combination of the rice straw removal and the application of the rice straw compost mitigated GHG emissions, and the mitigation effect in the continuous application field was higher compared with the initial ones. Converting the estimate value per 1 ha, the mitigation effect in the initial and continuous applications was −2.0 and −3.9 Mg CO2-eq ha−1 y−1, respectively. Compared to the result of the simple comparison of the impact on the net GHG balance in the same area (), the estimated value considering area for rice straw removal was greatly lower in the initial application field and was similar in the continuous application field. These results suggested that consideration should be given to the area of the rice straw removal when evaluating the influence of the combination of the rice straw removal and rice straw compost application on the GHG balance. In addition, this estimate assumes a combination of a field that continues to remove the rice straw in the long-term and a field that continues to apply the rice straw compost in the long-term. However, in reality, it is considered practical to combine the rice straw removal every year from all the fields and apply the rice straw compost to some fields once every few years by rotation. The effect of the organic matter application once every several years on soil carbon storage, crop productivity and the GHG balance is not clearly understood, and future research is merited.
As described above, the result of this time period may underestimate the soil carbon sequestration due to an evaluation of the plowed layer at a depth of only 0−15 cm. Considering the carbon sequestration of the subsoil, it is possible that the balance between the soil carbon sequestration and the increase in CH4 emissions due to the application of organic matter may change. In addition, in this study, assuming that the increase in soil carbon storage is linear, the net GHG balance of the field with continuous application of organic matter is evaluated (Fig. S1). However, as the period of continuous application becomes longer, the soil carbon sequestration rate decreases (Shiga et al. Citation1985; Yokozawa et al. Citation2010), so there is a possibility that overestimation of the soil carbon sequestration rate due to continuous application of organic matter occurs in this study. Furthermore, unlike the soil carbon sequestration, the increase in CH4 emission and net GHG balance by the organic matter application throughout the application period cannot be evaluated in this study. As the soil carbon sequestration due to the continuous application of organic matter is not linear, the increase in the CH4 emissions may not be linear as well. To accurately estimate the balance between the two, it is necessary to quantitatively evaluate the relationship between the soil carbon storage and CH4 emissions and to evaluate them with the DNDC model (Katayanagi et al. Citation2016; Minamikawa et al. Citation2014) and the Roth C model (Shirato et al. Citation2011). The rice straw compost used in this study is popular in the long-term organic matter application experiments (Kanamori Citation2000), but since the combine harvest has become widespread, and scattering the rice straw to the field has become popular now, it is not realistic. It is thought that evaluation with other organic matter, such as livestock manure compost or evaluation by using a model, will be necessary in the future.
5. Conclusions
The combination of the rice straw removal and rice straw compost application mitigated GHG emissions from the paddy fields due to its accumulation in the soil carbon and the reduction in CH4 emissions. Although the mitigation effect by the combination of rice straw removal and rice straw compost application decreases by continuing the application of organic matter, even when continuing for 40 years, the mitigation effect was maintained at approximately 79% of initial application by comparing in the same area basis. The combination is considered to be a sustainable management that can mitigate GHG emissions while improving crop productivity.
Supplemental Material
Download Zip (445.7 KB)Acknowledgments
The authors would like to thank the staff members of the Hokuriku Research Station, Central Region Agricultural Research Center, NARO for support with the management of the experimental field.
Supplementary material
Supplemental data for this article can be accessed here.
Additional information
Funding
References
- Akiyama H, Yagi K, Yan XY 2005: Direct N2O emissions from rice paddy fields: summary of available data. Glob. Biogeochem. Cycles, 19, GB1005.
- Cheng W, Kimani SM, Kanno T, Tang S, Oo AZ, Tawaraya K, Sudo S, Sasaki Y, Yoshida N 2018: Forage rice varieties Fukuhibiki and Tachisuzuka emit larger CH4 than edible rice Haenuki. Soil Sci. Plant Nutr., 64, 77–83. doi:10.1080/00380768.2017.1378569
- Cheng W, Padre AT, Sato C, Shiono H, Hattori S, Kajihara A, Aoyama M, Tawaraya K, Kumagai K 2016: Changes in the soil C and N contents, C decomposition and N mineralization potentials in a rice paddy after long-term application of inorganic fertilizers and organic matter. Soil Sci. Plant Nutr., 62, 212–219.
- Cheng W, Yagi K, Akiyama H, Nishimura S, Sudo S, Fumoto T, Hasegawa T, Hartley AE, Megonigalet JP 2007: An empirical model of soil chemical properties that regulate methane production in Japanese rice paddy soils. J. Environ. Qual., 36, 1920–1925.
- FAO (Food and Agriculture Organization of the United Nations) 2006: World Reference Base for Soil Resources 2006. FAO, Rome.
- Higashi T, Yunghui M, Komatsuzaki M, Miura S, Hirata T, Araki H, Kaneko N, Ohta H 2014: Tillage and cover crop species affect soil organic carbon in Andosol, Kanto, Japan. Soil Tillage Res., 138, 64–72.
- Inubushi K, Saito H, Arai H, Ito K, Endoh K, Yashima MM 2018: Effect of oxidizing and reducing agents in soil on methane production in Southeast Asian paddies. Soil Sci. Plant Nutr., 64, 84–89. doi:10.1080/00380768.2017.1401907
- Inubushi K, Wada H, Takai Y 1984: Easily decomposable organic matter in paddy soil, IV. Relationship between reduction process and organic matter decomposition. Soil Sci. Plant Nutr., 30, 189–198.
- IPCC (Intergovernmental Panel on Climate Change 2013: In Climate change 2013: the physical science basis, chapter 8 anthropogenic and natural radiative forcing. contribution of working group i to the fifth assessment report of intergovernmental panel on climate change. Ed. T Stocker, et al. http://www.ipcc.ch/report/ar5/wg1/ (December 2018)
- Kanamori T 2000: Long-term field experiments on successive application of chemical fertilizers and composts as organic matters in national and prefectural agricultural research stations of Japan. Misc. Publ. Nat. Agric. Res. Cent., 42, 1–303. in Japanese.
- Katayanagi N, Fumoto T, Hayano M et al. 2016: Development of a method for estimating total CH4 emission from rice paddies in Japan using the DNDC-Rice model. Sci. Total Environ., 547, 429–440.
- Kim SY, Gutierrez J, Kim PJ 2016: Unexpected stimulation of CH4 emissions under continuous no-tillage system in mono-rice paddy soils during cultivation. Geoderma, 267, 34–40.
- Koga N 2017: Tillage, fertilizer type, and plant residue input impacts on soil carbon sequestration rates on a Japanese Andosol. Soil Sci. Plant Nutr., 63, 396–404. doi:10.1080/00380768.2017.1355725
- Koga N, Sawamoto T, Tsuruta H 2006: Life cycle inventory-based analysis of greenhouse gas emissions from arable land farming systems in Hokkaido, northern Japan. Soil Sci. Plant Nutr., 52, 564–574. doi:10.1111/j.1747-0765.2006.00072.x
- Koga N, Tsuji H 2009: Effects of reduced tillage, crop residue management and manure application practices on crop yields and soil carbon sequestration on an Andisol in northern Japan. Soil Sci. Plant Nutr., 55, 546–557.
- Kubota M 1992: Reasonable application of rice straw to wet and semiwet paddy fields with heavy clay soil in Niigata. J. Niigata Agric. Exp. Stn., 39, 1–87. in Japanese with English summary.
- Kumagai K, Shiono H, Morioka M, Nakagawa K 2010: Effect of application of livestock dung compost instead of spring incorporation of rice straw on methane emission from paddy fields in Yamagata. Bull. Yamagata Pref. Agric. Res., 2, 1–18. in Japanese with English summary.
- Lal R 2004: Soil carbon sequestration to mitigate climate change. Geoderma, 123, 1–22.
- Lee CH, Park KD, Jung KY, Ali MA, Lee D, Gutierrez J, Kim PJ 2010: Effect of Chinese milk vetch (Astragalus sinicus L.) as a green manure on rice productivity and methane emission in paddy soil. Agric. Ecosyst. Environ., 138, 343–347.
- Li X, Ma J, Yao U, Liang S, Zhang G, Xu H, Yagi K 2014: Methane and nitrous oxide emissions from irrigated lowland rice paddies after wheat straw application and midseason aeration. Nutr. Cycl. Agroecosyst., 100, 65–76.
- Minamikawa K, Fumoto T, Itoh M, Hayano M, Sudo S, Yagi K 2014: Potential of prolonged midseason drainage for reducing methane emission from rice paddies in Japan: a long-term simulation using the DNDC-Rice model. Biol. Fertil. Soils, 50, 879–889.
- Minasny B, Malone BP, McBratney AB et al. 2017: Soil carbon 4 per mille. Geoderma, 292, 59–86
- Mitsuchi M 1974: Characters of humus formed under rice cultivation. Soil Sci. Plant Nutr., 20, 249–259.
- Miura Y 2003: Rice straw management for mitigation of methane emission from paddy field. Spe. Bull. Fukushima Pref. Agric. Exp. Stn., 7, 1–38. in Japanese.
- Nagumo Y, Oyanagi W, Tanahashi T 2016: Estimate of methane emission potential of organic matter from amount of acid detergent soluble organism. Jpn. J. Soil Sci. Plant Nutr., 87, 455–457. in Japanese.
- Naser HM, Nagata O, Tamura S, Hatano R 2007: Methane emissions from five paddy fields with different amounts of rice straw application in central Hokkaido, Japan. Soil Sci. Plant Nutr., 53, 95–101. doi:10.1111/j.1747-0765.2007.00105.x
- Ohyama N 1982: Decomposition and accumulation of some kinds of organic matters applied to the paddy field and their effects on growth of rice plant. Tohoku Agric. Res., 30, 57–72. in Japanese.
- Paustian K, Andrén O, Janzen H et al. 1997: Agricultural soil as a C sink to offset CO2 emissions, Soil Use Manage., 13, 230–244
- Sander BO, Samson M, Sanchez PB, Valencia KP, Demafelix EAM, Buresh RJ 2018: Contribution of fallow periods between rice crops to seasonal GHG emissions: effect of water and tillage management. Soil Sci. Plant Nutr., 64, 200–209. doi:10.1080/00380768.2018.1440937
- Shiga H, Ohyama N, Maeda K, Suzuki M 1985: An evaluation of different organic materials based on their decomposition patterns in paddy soils. Bull. Natl. Agric. Res. Cent., 5, 1–19. in Japanese with English summary.
- Shiono H, Saito H, Nakagawa F, Nishimura S, Kumagai K 2014: Effects of crop rotation and rice straw incorporation in spring on methane and nitrous oxide emissions from an upland paddy field in a cold region of Japan. Jpn. J. Soil Sci. Plant Nutr., 85, 420–430. in Japanese with English summary.
- Shirato Y, Yagasaki Y, Nishida M 2011: Using different versions of the Rothamsted Carbon model to simulate soil carbon in long-term experimental plots subjected to paddy–upland rotation in Japan. Soil Sci. Plant Nutr., 57, 597–606.
- Shiratori Y, Watanabe H, Furukawa Y, Tsuruta H, Inubushi K 2007: Effectiveness of a subsurface drainage system in poorly drained paddy fields on reduction of methane emissions. Soil Sci. Plant Nutr., 53, 387–400.
- Shoji S, Mae T 1984: Dynamics of inorganic nutrients and water. In Ecophysiology of Agricultural Plants, Ed. Sato K, pp. 97–120. Buneido, Tokyo in Japanese
- Soil Survey Staff 2010: Keys to Soil Taxonomy 11th ed. USDA. Natural Resources Conservation Service, Washington, DC.
- Sumida H, Kato N, Nishida M 2002: Fate of fertilizer nitrogen and compost nitrogen in long-term experiments on gray lowland soil in a cool region. Bull. Natl. Agric. Res. Cent. Tohoku Reg., 100, 49–59. in Japanese with English summary.
- Sumida H, Kato N, Nishida M 2005: Depletion of soil fertility and crop productivity in succession of paddy rice-soybean rotation. Bull. Natl. Agric. Res. Cent. Tohoku Reg., 103, 39–52. in Japanese with English summary.
- Takakai F, Ichikawa J, Ogawa M, Ogaya S, Yasuda K, Kobayashi Y, Sato T, Kaneta Y, Nagahama K 2017a: Suppression of CH4 emission by rice straw removal and application of bio-ethanol production residue in a paddy field in Akita, Japan. Agriculture, 7, 21.
- Takakai F, Kikuchi T, Sato T, Takeda M, Sato K, Nakagawa S, Kon K, Sato T, Kaneta Y 2017b: Changes in the nitrogen budget and soil nitrogen in a field with paddy–upland rotation with different histories of manure application. Agriculture, 7, 39.
- Takakai F, Nakagawa S, Sato K, Kon K, Sato T, Kaneta Y 2017c: Net greenhouse gas budget and soil carbon storage in a field with paddy–upland rotation with different history of manure application. Agriculture, 7, 49.
- Tirol-Padre A, Minamikawa K, Tokida T, Wassmann R, Yagi K 2018: Site-specific feasibility of alternate wetting and drying as a greenhouse gas mitigation option in irrigated rice fields in Southeast Asia: a synthesis. Soil Sci. Plant Nutr., 64, 2–13. doi:10.1080/00380768.2017.1409602
- Toma Y, Oomori S, Maruyama A, Ueno H, Nagata O 2016: Effect of the number of tillages in fallow season and fertilizer type on greenhouse gas emission from a rice (Oryza sativa L.) paddy field in Ehime, southwestern Japan. Soil Sci. Plant Nutr., 62, 69–79. doi:10.1080/00380768.2015.1109999
- Watanabe A, Katoh K, Kimura M 1993: Effect of rice straw application on CH4 emission from paddy fields. I. Effect of weathering of rice straw in the field during off-crop season. Soil Sci. Plant Nutr., 39, 701–706.
- Watanabe A, Satoh Y, Kimura M 1995: Estimation of the increase in CH4 emission from paddy soils by rice straw application. Plant Soil, 173, 225–231.
- Watanabe A, Takeda T, Kimura M 1999: Evaluation of carbon origins of CH4 emitted from rice paddies. J. Geophys. Res., 104(D19), 23623–23630.
- Yagi K, Minami K 1990: Effect of organic matter application on methane emission from some Japanese paddy fields. Soil Sci. Plant Nutr., 36, 599–610.
- Yasuda M, Okada T 1998: Effects of long-term application of organic matters on nutrient uptake of rice. Tohoku Agric. Res., 51, 59–60. in Japanese.
- Yokozawa M, Shirato Y, Sakamoto T, Yonemura S, Nakai M, Ohkura T 2010: Use of the RothC model to estimate the carbon sequestration potential of organic matter application in Japanese arable soils. Soil Sci. Plant Nutr., 56, 168–176.