ABSTRACT
Sulfur (S) and Nitrogen (N) metabolisms in plants are interacted and it is known that S deficiency decrease N absorption and metabolism. In leguminous plants S deficiency also decreases N2 fixation by rhizobia in the nodules. Deep placement of a controlled-release N fertilizer is a good method to provide nitrogen to soybean without inhibiting N2 fixation; thus, it was hypothesized that this method is able to provide nitrogen effectively to sulfur-deficient soybean plants. In this study effects of deep placement of coated urea on S-N physicological interaction, growth and productivity in soybean plants were examined using pot experiments. Soybean plants were grown with sulfate concentrations of 30, 100, or 1000 μM, with or without deep placement of coated urea. Shoot weights at the developing stage were not affected by S deficiency. SPAD values of leaves during the flowering stage decreased with S deficiency and increased with the deep placement of coated urea. S deficiency decreased seed weight per plant at the harvesting stage, but this decrease was attenuated by the deep placement of coated urea. N and S content in shoots at the developing stage increased with the deep placement of coated urea, whereas in seeds, only the N content increased. N2 fixation activity based on the relative ureide-N content in xylem sap indicated that the deep placement of coated urea did not inhibit N2 fixation activity at the early flowering stage. Without deep placement of coated urea, the relative ureide-N content decreased under S deficiency at the seed filling stage. These results suggest that the deep placement of coated urea is an efficient method to supply N to support soybean yield under S deficiency.
Abbreviations: Deep+: with deep placement of coated urea; Deep–: without deep placement of coated urea
1. Introduction
The assimilation processes of sulfur (S) and nitrogen (N) are closely interlinked in plants as both elements are components of proteins. Adequate S assimilation is required for functional N assimilation and vice versa (Kopriva et al. Citation2002, Citation2004; Kopriva and Rennenberg Citation2004). S is taken up by plants as sulfate, and is then reduced to sulfide, which reacts with O-acetyl-L-serine (OAS) to produce cysteine. The precursor of OAS is serine, which is produced by N assimilation. A high N:S ratio leads to increased OAS concentrations, which is a signal eliciting the up-regulation of S assimilation (Kim et al. Citation1999; Ohkama-Ohtsu et al. Citation2004). These results demonstrate that a minimum amount of N is needed to facilitate efficient S assimilation in plants.
Not only S is important for adequate N assimilation plants, S is needed for N2 fixation by rhizobia in the nodules of leguminous plants. Nitrogenase, the enzyme responsible for N2 fixation, contains several S atoms, for instance in the [Fe-S] clusters in the protein (Long et al. Citation2015). In the model legume Lotus japonicus, the nodule-specific sulfate transporter (SST1) was shown to be required for nodule formation and N2 fixation (Krusell et al. Citation2005). In peas (Pisum sativum), the effects of S deficiency on growth were shown to be caused by a shortage of N due to decreased N2 fixation (Zhao et al. Citation1999). Scherer and Lange (Citation1996) reported decreased N2 fixation due to S deficiency in several legume plants, such as Medicago sativa, Trifolium Pratense, Vicia faba, and P. sativum.
Among leguminous plants, soybean is an important plant protein source for animals and humans as its seeds contain high amounts of protein (approximately 40%). Numerous studies have shown that deep placement of controlled-release N fertilizer, such as coated urea or lime N, promotes soybean growth and increases seed yield due to a supplementation of N during the seed filling stage without inhibition of N2 fixation (Kaushal et al. Citation2002, Citation2004, Citation2005a, Citation2005b). As described above, N absorption, assimilation, and N2 fixation are inactivated by S deficiency. We hypothesized that deep placement of coated urea effectively provides N to N deficient soybean plants caused by S deficiency, which should, in turn, lead to increase S utilization as S and N metabolism are interacted. To analyze this hypothesis, pot experiments were performed to examine the effects of deep placement of coated urea on the growth, yield, S and N contents of soybeans under S deficiency.
2. Materials and methods
2.1. Seed sterilization and rhizobium inoculation
Soybean seeds of Glycine max (L.) Merr cv ‘Enrei’ were used in this study. Seeds were sterilized using 0.7 L L −1 ethanol for 1 min and a 3 g L −1 sodium hypochlorite solution for 4 min. After this, the sterilized seeds were washed thoroughly using tap water and were inoculated with Bradyrhizobium diazoefficiens USDA 110. For this, a single colony of B. diazoefficiens USDA 110 was cultured in 100 mL of YM broth medium (YMB, Jordan Citation1984) on a shaker at 100 rpm and at 28°C for one week. Cells were collected by centrifugation and were re-suspended in 100 mL of S-deprived N-free nutrient solution modified from Tejima et al. (Citation2003). The nutrient solution contained 1 mol m−3 CaCl2, 0.5 mol m−3 KH2PO4, 10 mmol m−3 Fe-EDTA, 250 mmol m−3 MgCl2, 500 mmol m−3 KCl, 1 mmol m−3 MnCl2, 2 mmol m−3 H3BO3, 0.5 mmol m−3 ZnCl2, 0.2 mmol m−3 CuCl2, 0.1 mmol m−3 CoCl2, and 0.1 mmol m−3 Na2MoO2; the pH was adjusted to 6.8 by the addition of NaOH. The sterilized soybean seeds were soaked in the bacterial solution for 15 minutes with gentle shaking.
2.2. Soybean culture conditions
The inoculated soybean seeds were placed in 1/5000 long-type Wagner pots (200 cm2 × 30 cm) on 15 May 2017. The pots were filled with vermiculite (Yoshino Gypsum CO., LTD, Tokyo, Japan) to a height of 25 cm. Coated urea (100-d sigmoid type; LP coat S100, JCAM Agri. CO., LTD., Tokyo, Japan) was applied at a depth of 20 cm at an amount of 2.836 g per pot, which corresponded to 100 kg N ha−1 (Kaushal et al. Citation2002). The controls received no additional N fertilizer. The treatment with deep placement of coated urea is termed ‘Deep+’ and the treatment without fertilizer is termed ‘Deep–,’ hereafter. Sulfate concentrations in the soybean nutrient solutions were adjusted at 30 μM (severe S deficiency), 100 μM (moderate S deficiency), and 1000 μM (S sufficiency) by adding Na2SO4 to the S-deprived N-free nutrient solution, supplemented with 0.5 mM urea as N source. The culture solution was applied to each pot twice every week to 60% of the maximum water-holding capacity of the vermiculate (3.35 g/g). Water purified by reverse osmosis was added once or twice every week to keep the vermiculite at 60% of the maximum water-holding capacity. The soybean plants were grown in a glass house under natural light, and the temperature was kept at 25°C from 08.00 to 17.00 and at 18°C from 17.00 to 08.00. Six replicates of each treatment condition were used. Each pot contained three plants, and one plant of each pot was cut 40 and 67 days after germination, respectively, for xylem sap collection. The remaining plants were grown until seed harvest.
2.3. Determination of phenological growth stages
Phenological growth stages were determined according to the BBCH scale (Munger et al. Citation1997).
2.4. Leave SPAD measurements
The soil-plant analysis development (SPAD) values were measured using a SPAD-502 Plus device (Konica Minolta, Inc., Tokyo, Japan). SPAD values were determined at the center part of trifoliate leaves at the second node from the top and were averaged per trifoliate.
2.5. N content in plant shoots
The upper parts of whole shoots were cut above the cotyledons, 40 and 67 days after sowing, for xylem sap collection, and mature seeds collected at the harvest stage were dried in a ventilator oven at 80°C for two days. Dry weights were recorded and after this, the dried shoots were powdered using a blender. The N and C content of the powder was determined using an N.C-ANALYZER device (SUMIGRAPH NC-TR22, Sumika Chemical Analysis Service Ltd., Tokyo, Japan).
2.6. S content in plant shoots
The dried shoots sampled 40 and 67 days after sowing and mature seeds collected at the harvest stage were powdered as described above and digested with HNO3. Total S content was determined using inductively coupled plasma–mass spectrometry (Agilent 7800 ICP-MS, Agilent) with 2 ppb indium as an internal standard.
2.7. Relative ureide-N content in xylem sap
Xylem saps were collected 40 and 67 days after sowing and the relative ureide-N contents in them determined as described by Takahashi et al. (Citation1991, Citation1992) and Sakazume et al. (Citation2014). The root bleeding sap was collected and the concentrations of ureide-N, NO3–N, and amino-N in the sap were determined. The shoots of 1 plant in each plot were decapitated at about 3 cm above ground in the morning from 9:30 till 12:00 AM, and a silicon tube was attached to the decapitated stem. Approximately 0.5 ml of root-bleeding sap was collected for the initial 60 min, and the sap was stored at −20°C until analysis. The ureide-N concentration was measured by the Young-Conway’s method (Young and Conway Citation1942), the NO3 – N concentration was analyzed by the method of Cataldo (Cataldo et al. Citation1974), and the amino-N concentration was measured by a ninhydrin method (Herridge Citation1984). Relative ureide-N content in the sap samples was determined as (4 x ureide-N concentration/4 x ureide-N concentration + 2 x amino-N concentration + NO3-N concentration) x 100 (%). Analysis was performed in three biological replicates.
3. Results
3.1. Plant growth
Soybean seeds inoculated with B. diazoefficiens USDA 110 were sown on 15 May 2017, and their growth phenology was examined at intervals of approximately 10 days until 15 September 2017, according to the BBCH scale (Munger et al. Citation1997). The growth stages were similar between the different S treatments, regardless of the coated urea treatment (Supplementary Table 1). Flowering started at the end of June or in the beginning of July, wet seeds were observed at the end of July, and dry seeds were harvested in mid-September.
The soil-plant analysis development (SPAD) meters is widely used to monitor chlorophyll contents to know N status in leaves (Matsunaka et al., Citation1997; Chen et al. Citation2011; Xiong et al. Citation2015). The SPAD values of trifoliates at the second node from the top and at the flowering stage are shown in . Deep + significantly increased the SPAD values at S100 and S30 on 16th June, and at S30 on 29th June. On 13th July, the highest SPAD values were recorded in Deep+ plants with 1000 μM sulfate, which was significantly higher than that in Deep + with 100 μM sulfate.
Table 1. Effects of S supply and deep placement of coated urea on SPAD values in leaves at the flowering stage
Dry weights of shoots 40 days after sowing are shown in . No significant effect of sulfate concentrations on shoot dry weight in Deep+ and Deep – plants was observed when plants started to flower 40 days after sowing (24 June). The shoot weights of Deep+ plants were higher than those of Deep – plants; the increase being most significant with 100 μM sulfate.
Figure 1. Effects of S supply and deep placement of coated urea on shoot biomass at the developing stage. Shoot dry weights were determined 40 days after sowing. Means ± standard deviations of four biological replicates are shown. The asterisk indicates a significant difference using a Student’s t-test (P < 0.05)
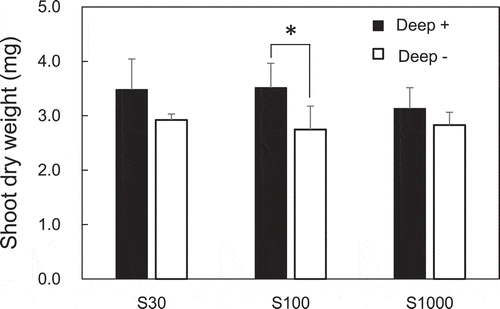
3.2. Soybean yield
Mature dried seeds were harvested, weighed, and counted. Seed weight per plant in Deep – plants was significantly lower when treated with 30 μM sulfate than when treated with 1000 μM (). No difference in seed weights was observed between sulfate concentrations in Deep+ plants. At 30 μM sulfate concentration, the seed weight in Deep+ plants was significantly higher than that in Deep – plants.
Figure 2. Effects of S supply and deep placement of coated urea on soybean seed yield and yield components at the harvest stage. Mature dry seeds were harvested, and seed dry weight per plant (a), hundred-seed weight (b), seed number per pod (c), and pod number per plant (d) were determined. Means ± standard deviations of four biological replicates are shown. Asterisks indicate significant differences using a Student’s t-test (P < 0.05)
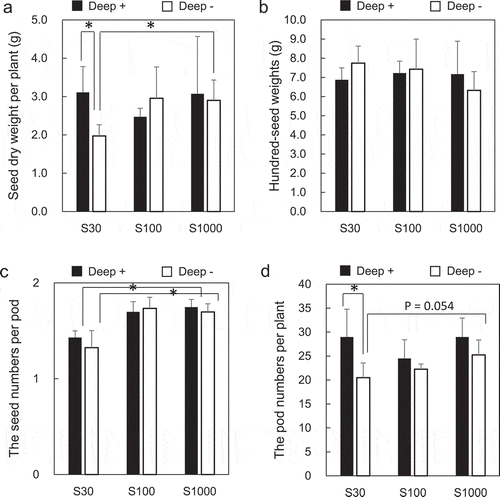
Hundred-seed weights showed no significant difference between sulfate concentrations in Deep+ and Deep – plants (). The number of seeds per pod was significantly lower at 30 μM sulfate than at 1000 μM in Deep+ and Deep – plants (), whereas in Deep – plants the number of pods per plant was lower at 30 μM sulfate than at 1000 μM, which was marginally significant (P = 0.054; ). At 30 μM sulfate, the number of seeds per pod was significantly higher in Deep+ plants than that in Deep – plants.
Figure 3. Effects of S supply and deep placement of coated urea on N concentrations and content in shoots of soybean at developing stage and in seeds at harvest stage. N concentration in shoots (a) and N content in shoots per plant (b) were determined 40 days after sowing, and N concentration in mature dry seeds (c) and N content in mature dry seeds per plant (d) were determined at the harvest stage. Means ± standard deviations of four biological replicates are shown. Asterisks indicate significant differences using a Student’s t-test (P < 0.05)
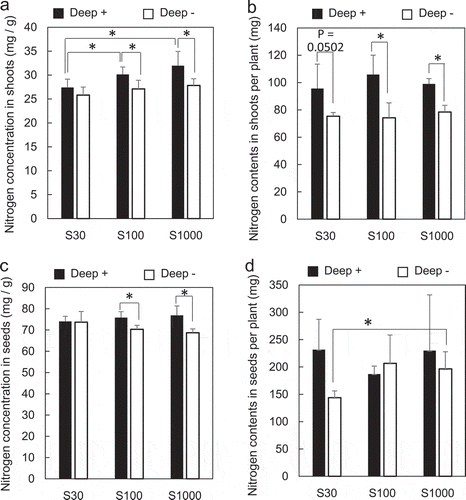
3.3. N content in shoots and seeds
Total N content in shoots at 40 days after sowing and in dried mature seeds were determined. No significant difference in N concentrations between 30, 100, and 1000 μM sulfate was observed in Deep – shoots (). N concentrations in Deep+ plant shoots were significantly higher than those in Deep – plants at 100 and 1000 μM (P < 0.05), and N concentrations at 30 μM of sulfate were lower than those at 100 and 1000 μM sulfate in Deep+ plants.
N content per shoot was calculated by multiplying N content by plant dry weight (). As shoot dry weights increased with the Deep+ treatment (), the total N content per shoot also increased, which was significant at 100 and 1000 μM (P < 0.05) and marginally significant at 30 μM sulfate (P = 0.0502).
N content in seeds is shown in . No significant differences in seed N concentration were observed between 30, 100, and 1000 μM sulfate in Deep+ and Deep- plants. In the seeds of Deep+ plants, N concentrations were higher than those in Deep – plants significantly at 100 and 1000 μM sulfate.
Figure 4. Effects of S supply and deep placement of coated urea on S concentrations and content in soybean shoots at the developing stage and in seeds at the harvest stage. S concentration in shoots (a) and the S content in shoots per plant (b) were determined at 40 days after sowing, and S concentration in mature dry seeds (c) and the S content in mature dry seeds per plant (d) were determined at the harvest stage. Means ± standard deviations of four biological replicates are shown. Asterisks indicate significant differences using a Student’s t-test (P < 0.05)
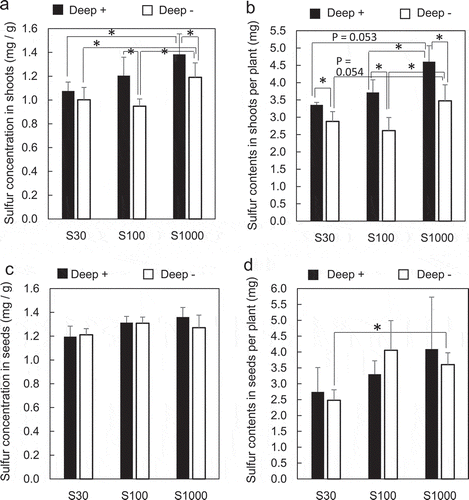
N concentration in seeds per plant is shown in . As the seed weights increased in Deep+ plants at 30 μM sulfate (), the seed N content per plant also increased at this sulfate concentration. In Deep–, the seed N content per plant was lower at 30 μM sulfate than at 1000 μM sulfate. In contrast, the seed N concentration per plant was similar in 30 μM and 1000 μM sulfate in Deep+ plants.
3.4. S content in shoots and seeds
Total S content was determined in shoots 40 days after sowing and in dried mature seeds. Shoot S concentrations in Deep – plants were lower at 30 and 100 μM sulfate than at 1000 μM (). In Deep+ plants, shoot S concentrations were lower at 30 μM sulfate than at 1000 μM. The S concentration in Deep + plants increased significantly compared to that in Deep – plants at 100 μM and 1000 μM sulfate.
The shoot S content per plant was calculated by multiplying S concentration by the plant’s dry weight (). As shoot dry weights increased in Deep+ plants (), the total S content of shoots per plant also increased significantly in Deep+ plant at all sulfate concentrations. Shoot S content per plant was lower at 30 μM sulfate than at 1000 μM sulfate, which was marginally significant in Deep+ (P = 0.053) and Deep – (P = 0.054) plants.
S concentrations in seeds are shown in . No significant differences in seed S concentrations were observed between 30, 100, and 1000 μM sulfate in Deep – and Deep+ plants.
The seed S content per plant is shown in . The seed S content per plant was lower in Deep – plants treated with at 30 μM sulfate than in plants treated with 1000 μM sulfate. In contrast, the seed S content per plant showed no significant difference between Deep+ plants treated with 30 μM and 1000 μM sulfate.
3.5. Relative ureide-N content in xylem sap
The relative ureide-N content in the ureide-N + NO3–N + amino-N pool in the xylem sap of nodulated soybean is known to be an indicator of N2 fixation activity in nodules (Herridge Citation1984). The relative ureide-N content in xylem sap was determined 40 (June 24) and 67 days (July 21) after sowing (). The sampling procedure 40 days after sowing produced an insufficient amount of xylem sap from Deep – plants grown at 1000 μM sulfate; thus, the respective analysis was not performed. No significant difference in the relative ureide-N content was observed between sulfate concentrations and between Deep+ and Deep – plants 40 days after sowing when plants just started to flower. The relative ureide-N content of Deep+ plants at 1000 and 100 μM sulfate concentration were only slightly but significantly lower than Deep – plants 67 days after sowing when pods were developing. In Deep – plants, the relative ureide-N content at 30 µM sulfate was significantly lower than at 1000 µM sulfate, 67 days after sowing.
Table 2. Ureide, amide, and nitrate concentration and relative ureide-N content in xylem sap
4. Discussion
In the present study, soybean plants were grown in pots using hydroponic solutions with three different sulfate concentrations in Deep+ and Deep – conditions. To compare the effects of sulfate concentration in the hydroponic solution or deep placement, pot culture was used for all of plant growth conditions being the same other than sulfate and deep placement. In our pot culture, plant shoot dry weights and seed weights were lower than those in field experiments (Kaushal et al. Citation2002, Citation2004), which is considered to be due to limited space for root elongation in the pots compared to the field.
Forty days after sowing when plants started to flower and leave size was still increasing, shoot dry weight did not differ among sulfate concentrations (). In Deep+ plants, shoot dry weights were significantly higher than in Deep – at 100 μM sulfate and at other sulfate concentrations they were also tended to be higher in Deep+ although the difference was not significant. This suggests the effect of N supply on soybean growth during the developing stage is stronger than that of S supply.
The SPAD values which is an indicator of chlorophyll contents in leaves were higher with Deep+ than with Deep – treatment in early flowering stages such as 16th and 29th June (). In the early in flowering stage 40 days after sowing, S concentrations in shoots were lower in S deficient conditions (, ) and the Deep+ treatment increased S and N concentrations in shoots (, ). It is reported that chlorophyll contents decrease by S deficiency (Marschner Citation1995) thus chlorophyll judged by SPAD values in flowering stages may thus be correlated with not only N but also S concentrations in shoots.
At the harvest stage, seed dry weights per plant were significantly lower at 30 μM sulfate than at 1000 μM sulfate in Deep – plants (), which indicates that S deficiency affected the seed yield. It is known that leguminous plants require a higher amount of S than Gramineae, which is evident from the S concentrations in their seeds (Marschner Citation1995). We therefore suggest that S deficiency has a stronger effect on soybean seed yields. Unexpectedly, the Deep+ treatment increased the seed dry weight per plant at 30 μM sulfate to the same level as that at 1000 μM sulfate (), which indicated that the Deep+ treatment may help to reduce the adverse effect of S deficiency on seed yield. The number of pods per plant produced trends similar to those of seed dry weight per plant (, ), which was a decreased under S deficiency but a compensatory effect by the Deep+ treatment. In contrast, the hundred-seed weights and the seed numbers per pod did not differ between the Deep+ and the Deep–treatment at 30 μM sulfate. These results indicate that the decrease in seed weight per plant is likely associated with the decrease in the number of pods per plant.
N content in shoots was not affected by S deficiency at the developing stage but it increased in the Deep+ plants (, ). At the harvest stage, N content in seeds was lower at 30 μM than at 1000 μM sulfate (). N and S are integral components of proteins and it is known that S is required for functional N utilization and vice versa (Kopriva et al. Citation2002, Citation2004; Kopriva and Rennenberg Citation2004). As soybean seeds contain high amounts of protein (up to 40%), S deficiency may adversely affect N utilization in soybean seeds. The Deep+ treatment, however, may help to compensate for S deficiency regarding seed N content in seeds ().
S concentrations in shoots at the developing stage and the S content in seeds at the harvest stage were lower at 30 μM sulfate than at 1000 μM sulfate (–). The Deep+ treatment increased S concentrations in shoots at the developing stage (, ), which was associated with an increase in N content in shoots (, ). Thus, increased N availability may help to enhance S utilization, as shown in previous studies (Kopriva et al. Citation2002, Citation2004; Kopriva and Rennenberg Citation2004). However, S content of seeds was not increased at 30 μM sulfate by the Deep+ treatment () despite increased N content in seeds (). This may be because S content of the hydroponic solution was below the concentration necessary to increase the S content in seeds. Even though S content in seeds did not increase in Deep+ plants at 30 μM sulfate (), the seed dry weights per plant increased (). Seed N content also increased at 30 μM sulfate in Deep+ plants (); thus, it is possible that the soybean plants adjusted the components of proteins under S deficiency. In soybeans, seed storage proteins with a high S content decrease whereas those with a low S content increase under S deficiency in order to keep total protein content stable (Blagrove et al. Citation1976; Gayler and Sykes Citation1985; Spencer et al. Citation1990; Fujiwara et al. Citation1992; Hirai et al. Citation1995; Naito et al. Citation1995).
Relative ureide-N 40 days after sowing was not affected by the Deep+ treatment, which indicated that N2 fixation was not inhibited by the treatment when the plants were in an early flowering stage (). This is in line with previous studies (Kaushal et al. Citation2002, Citation2004, Citation2005a, Citation2005b). The N2 fixation activity in soybean nodules peaks at approximately 35 days after sowing, and it decreases to less than one-third of the peak activity at approximately 60 days after sowing (Dalton et al. Citation1986). In our study, N2 fixation activity remained high in the Deep+ plants 40 days after sowing when N2 fixation is almost at its peak.
In the Deep plants, S deficiency did not affect the shoot dry weight in the developing stage () but SPAD values () and N content in seeds decreased () in correlation with decreased S content in seeds (). This result indicated that S is a limiting factor for N2 fixation and N absorption under S deficiency. In Deep – plants, the relative ureide-N content was lower at 30 μM sulfate than at 1000 μM sulfate, 67 days after sowing, indicating that S deficiency decreased N2 fixation activity. Decreased N2 fixation under S deficiency was also observed previously in several legume species (Zhao et al. Citation1999; Scherer and Lang Citation1996). In contrast, S concentrations and content in shoots and seeds of Deep+ plants decreased under S deficiency (), whereas N content in the shoots () and seeds () were not repressed by S deficiency. The additional N supply from lower portions of roots during the reproductive stage may have supported N absorption and N2 fixation during later stages, and thus, resulted in seed yields similar to those under S sufficiency.
In conclusion, the Deep+ treatment effectively provided nitrogen to soybean plants even under sulfur deficiency, and it enhanced S utilization in shoots. An increase in seed N content resulted in increased seed yields. Our study suggested that the Deep+ fertilization treatment is a good method to increase soybean yield under S deficiency.
Supplemental_Table_S1.pdf
Download PDF (138.2 KB)Acknowledgments
We thank Dr. Salem Djedidi in Tokyo University of Agriculture and Technology for his critical reading of the manuscript.
Supplemental material
Supplemental data for this article can be accessed here.
Additional information
Funding
References
- Blagrove RJ, Gillespie JM, Randall PJ 1976: Effect of sulphur supply on the seed globulin composition of Lupinus angustifolius. Funct. Plant. Biol., 3, 173–184. doi:10.1071/PP9760173
- Cataldo KA, Schrader LE, Youngs VL 1974: Analysis by digestion and colorimetric assay of total nitrogen in plant tissues high in nitrate. Crop. Sci., 14, 854–856. doi:10.2135/cropsci1974.0011183X001400060024x
- Chen X, Wu P, Xhao W, Li S 2011: Effects of atmospheric ammonia enrichment and nitrogen status on the growth of maize. Soil Sci. Plant Nutr., 58, 32–40. doi:10.1080/00380768.2011.654349
- Dalton DA, Russell SA, Hanus FJ, Pascoe GA, Evans HJ 1986: Enzymatic reactions of ascorbate and glutathione that prevent peroxide damage in soybean root nodules. Proc. Natl. Acad. Sci. USA., 83, 3811–3815. doi:10.1073/pnas.83.11.3811
- Fujiwara T, Hirai MY, Chino M, Komeda Y, Naito S 1992: Effects of S nutrition on expression of the soybean seed storage protein genes in transgenic petunia. Plant Physiol., 99, 263–268.
- Gayler KR, Sykes GE 1985: Effects of nutritional stress on the storage proteins of soybeans. Plant Physiol., 78, 582–585.
- Herridge DF 1984: Effect of nitrate and plant development on the abundance of nitrogenous solutes in root-bleeding and vacuum-extracted exudates of soybean. Crop Sci., 24, 173–179. doi:10.2135/cropsci1984.0011183X002400010041x
- Hirai MY, Fujiwara T, Chino M, Naito S 1995: Effects of sulfate concentrations on the expression of a soybean seed storage protein gene and its reversibility in transgenic Arabidopsis thaliana. Plant Cell Physiol., 36, 1331–1339.
- Jordan DC 1984: Bergey’s manual of systematic bacteriology. In Family III Rhizobiaceae, Krieg NR, Holt JG Ed., Vol. 1, pp. 234–244. Williams & Wilkins, Baltimore.
- Kaushal T, Onda M, Ito S, Yamazaki A, Fujikake H, Ohtake N, Sueyoshi K, Takahashi Y, Ohyama T 2004: Effect of placement of urea and coated urea on yield and quality of soybean (Glycine max (L.).) seed. Soil Sci. Plant Nutr., 50, 1245–1254. doi:10.1080/00380768.2004.10408600
- Kaushal T, Onda M, Ito S, Yamazaki A, Fujikake H, Ohtake N, Sueyoshi K, Takahashi Y, Ohyama T 2005a: N-15 analysis of the promotive effect of deep placement of slow-release N fertilizers on growth and seed yield of soybean. Soil Sci. Plant Nutr., 51, 885–892. doi:10.1111/j.1747-0765.2005.tb00123.x
- Kaushal T, Onda M, Ito S, Yamazaki A, Fujikake H, Ohtake N, Sueyoshi K, Takahashi Y, Ohyama T 2005b: Analysis of promotive effect of deep placement of slow release N fertilizers on growth and seed yield of soybean. Soil Sci. Plant Nutr., 51, 501–512. doi:10.1111/j.1747-0765.2005.tb00123.x
- Kaushal T, Suganuma T, Fujikake H, Ohtake N, Sueyoshi K, Takahashi Y, Ohyama T 2002: Effect of deep placement of calcium cyanamide, coated urea, and urea on soybean (Glycine max (L.) Merr.) seed yield in relation to different inoculation methods. Soil Sci. Plant Nutr., 48, 855–863. doi:10.1080/00380768.2002.10408712
- Kim H, Hirai MY, Hayashi H, Chino M, Naito S, Fujiwara T 1999: Role of O-acetyl-L-serine in the coordinated regulation of the expression of a soybean seed storage-protein gene by sulfur and nitrogen nutrition. Planta., 209, 282–289. doi:10.1007/s004250050634
- Kopriva S, Hartmann T, Massaro G, Honicke P, Rennenberg H 2004: Regulation of sulfate assimilation by N and S nutrition in poplar trees. Trees-Struct. Funct., 18, 320–326. doi:10.1007/s00468-003-0309-4
- Kopriva S, Rennenberg H 2004: Control of sulphate assimilation and glutathione synthesis: interaction with N and C metabolism. J. Exp. Bot., 55, 1831–1842. doi:10.1093/jxb/erh013
- Kopriva S, Suter M, von Ballmoos P, Hesse H, Krähenbühl U, Rennenberg H, Brunold C 2002: Interaction of sulfate assimilation with carbon and nitrogen metabolism. Lemna Minor. Plant Physiol., 130, 1406–1413. doi:10.1104/pp.007773
- Krusell L, Krause K, Ott T et al. 2005: The sulfate transporter SST1 is crucial for symbiotic nitrogen fixation in Lotus japonicus root nodules. Plant Cell., 17, 1625–1636. doi:10.1105/tpc.104.030106
- Long SR, Kahn M, Seefeldt L, Tsay YF, Kopriva S 2015: Chapter 16 Nitrogen and Sulfur. In Biochemistry & Molecular Biology of Plants, Ed. Buchanan BB, Gruissem W, Jones RL, pp. 746–768. Wiley Blackwell, Oxford, UK.
- Marschner H 1995: Mineral Nutrition of Higher Plants. Academic Press, London.
- Matsunaka T, Watanabe Y, Miyawaki T, Ichikawa N 1997: Prediction of grain protein content in winter wheat through leaf color measurements using a chlorophyll meter. Soil Sci. Plant Nutr., 43, 127–134. doi:10.1080/00380768.1997.10414721
- Munger P, Bleiholder H, Hack H, Hess M, Stauss R, Boom T, Weber E 1997: Phenological growth stages of the soybean plant (Glycine max L. Merr.): codification and description according to the BBCH scale. J. Agron. Crop Sci., 179, 209–217. doi:10.1111/j.1439-037X.1997.tb00519.x
- Naito S, Hirai MY, Inaba-Higano K, Nambara E, Fujiwara T, Hayashi H, Chino M 1995: Expression of soybean seed storage protein genes in transgenic plants and their response to sulfur nutritional conditions. J. Plant Physiol., 145, 614–619. doi:10.1016/S0176-1617(11)81272-1
- Ohkama-Ohtsu N, Kasajima I, Fujiwara T, Naito S 2004: Isolation and characterization of an Arabidopsis mutant that overaccumulates O-acetyl-L-Ser. Plant Physiol., 136, 3209–3222. doi:10.1104/pp.104.047068
- Sakazume T, Tanaka K, Aida H, Ishikawa S, Nagumo Y, Takahashi Y, Ohtake N, Sueyoshi K, Ohyama T 2014: Estimation of nitrogen fixation rate of soybean (Glycine max (L.) Merr.) by micro-scale relative ureide analysis using root bleeding xylem sap and apoplast fluid in stem. Bull. Facul. Agric. Niigata Univ., 67, 27–41.
- Scherer HW, Lange A 1996: N2 fixation and growth of legumes as affected by sulphur fertilization. Biol. Fertil. Soils, 23, 449–453. doi:10.1007/BF00335921
- Spencer D, Rerie WG, Randall PJ, Higgins TJV 1990: The regulation of pea seed storage protein genes by S stress. Funct. Plant Biol., 17, 355–363. doi:10.1071/PP9900355
- Takahashi Y, Chinushi T, Nagumo Y, Nakano T, Ohyama T 1991: Effect of deep placement of controlled release nitrogen fertilizer (coated urea) on growth, yield and nitrogen fixation of soybean plants. Soil Sci. Plant Nutr., 37, 223–231. doi:10.1080/00380768.1991.10415032
- Takahashi Y, Chinushi T, Nakano T, Ohyama T 1992: Evaluation of N2 fixation and N absorption activity by relative ureide method in field grown soybean plants with deep placement of coated urea. Soil Sci. Plant Nutr., 38, 699–708. doi:10.1080/00380768.1992.10416700
- Tejima K, Arima Y, Yokoyama T, Sekimoto H 2003: Composition of amino acids, organic acids, and sugars in the peribacteroid space of soybean root nodules. Soil Sci. Plant Nutr., 49, 239–247. doi:10.1080/00380768.2003.10410003
- Xiong D, Chen J, Yu T, Wanlin G, Xiaoxia L, Li Y, Peng S, Huang J 2015: SPAD-based leaf nitrogen estimation is impacted by environmental factors and crop leaf characteristics. Sci. Rep., 5, 13389. doi:10.1038/srep13389
- Young E, Conway CF 1942: On the estimation of allantoin by the Remini-Shryver reaction. J. Biol. Chem., 142, 839–853.
- Zhao FJ, Wood AP, McGrath SP 1999: Effects of sulphur nutrition on growth and N fixation of pea (Pisum sativum L.). Plant Soil., 212, 209–219. doi:10.1023/A:1004618303445