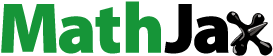
ABSTRACT
Hairy vetch (Vicia villosa Roth ssp. dasycarpa) mulch is widely used as green manure. This mulch decomposes in the soil and produces nitrous oxide (N2O) emissions. Although rice husk charcoal can be used as a soil conditioner to reduce N2O emissions, the effects of charcoal on the decomposition of vetch mulch and consequent N2O emissions have not been well studied. We conducted an incubation experiment to examine how the decomposition of vetch mulch, charcoal amendments and two soil moisture regimes (field capacity and at a slightly drier moisture regime) affect the mineralisation of N during decomposition. We applied high-performance size-exclusion chromatography and chemiluminescent N detection to quantify soluble organic-N compounds of various molecular sizes. Changes over time in inorganic-N, soluble organic-N fractions and N2O fluxes were measured in soils . We found that vetch mulch induced the accumulation of small-molecular-size soluble organic-N compounds in the early phase during decomposition; however, in wet soils with charcoal, the small-molecular-weight N fraction was depleted earlier than in wet soils without charcoal, suggesting more rapid mineralisation in wet soils. Charcoal in wet soils resulted in earlier and sharper N2O peak emissions and did not reduce cumulative N2O emissions in vetch mulch treatments, suggesting that previously reported N2O emission reductions due to charcoal do not occur in the soils . The rates of N2O emission did not correlate with the concentrations of soluble organic N and inorganic N, indicating that other factors control the N2O emission rates during the decomposition of vetch mulch. Our results indicated that the incorporation of charcoal does not reduce N2O emissions in soils where vetch mulch is applied. The depletion of nitrate-N in wet soils with charcoal demonstrates that adding charcoal can reduce nitrate-N leaching from soils.
KEY WORDS:
1. Introduction
Vicia villosa Roth ssp. dasycarpa (legume hairy vetch, HV) is used as green manure to supply nitrogen (N) to a wide variety of crop plants and to increase soil N. For example, a soil surface application of HV mulch provides 90–100 kg N ha−1 in a no-tillage corn (Zea mays L.) approach (Ebelhar et al. Citation1984). Therefore, HV can be used as a substitute for synthetic fertilisers (Power et al. Citation1991; Sainju et al. Citation2001). However, it has been reported in previous studies that the N use efficiency of cash crops is lower for N derived from HV mulch compared to the use efficiency with synthetic fertilisers. For example, in a 15N-labelling experiment in maize (Zea mays L.), Seo et al. Citation2006) determined that the N recovery rate with HV mulch was approximately half that with a synthetic fertiliser (ammonium sulphate). In addition, Alluvione et al. (Citation2009) and ShimotsuShimotsuma et al. (Citation2017) reported that a substantial amount of greenhouse gas (as nitrous oxide, N2O-N) is emitted as HV decomposes.
The main difference between synthetic fertiliser N and organic-fertiliser N (e.g. HV N) is that organic N must be mineralised before it becomes available to plants. In our previous study, we revealed that the magnitude of N2O emissions cannot be determined simply by fluctuations in inorganic N (ShimotsuShimotsuma et al. Citation2017). Therefore, in order to improve the N use efficiency of HV fertilisers by cash crops, more information on the fluctuation of organic N and inorganic N during the mineralisation (decomposition) process is needed.
The decomposition rates of plant residues vary in relation to soil conditions, such as temperature regime (Six et al. Citation2002), moisture regime (Harmon et al. Citation1986; Sierra Citation1997), the recalcitrance of the specific plant residues involved and the carbon/nitrogen (C/N) ratios of the residues (Cobo et al. Citation2002). Therefore, interactions among these factors should be investigated in order to understand the effects that these factors have on nitrogen mineralisation to fully understand the effect of the plant residue decomposition on nitrogen availability in soils and on the environmental risks. For example, when the soil temperature is within some optimum range, the soil moisture conditions are particularly important in controlling the decomposition rates and subsequent denitrification rates (a major source of N2O emissions) (Ruffo and Bollero Citation2003; Shelton et al. Citation2000).
Incorporating charcoal in soils is a well-known technique for controlling soil conditions. Charcoal as a soil amendment has received much interest as a technique for increasing the N retention capacity of soils (Lehmann and Joseph Citation2009). In addition, there have been many reports on the effects of charcoal amendments on the decomposition of organic matter in soils and on N cycling (Clough et al. Citation2013). In fact, charcoal amendment is often reported as a method for mitigating soil N2O emissions (Cayuela et al. Citation2013). However, such mitigative effects depend on specific soil conditions, such as the soil moisture content. Therefore, more work is needed to determine how the application of charcoal affects the soil conditions and the decomposition of plant residues (organic materials). Due to the fact that any organic source of N must undergo several decomposition steps to provide plant-available inorganic N to soils, studies should focus on how solid organic N (e.g. HV mulch) is converted into soluble organic N in soils and then into inorganic N. For example, data on the distribution of molecular sizes of soluble organic-N compounds during the decomposition process of HV in soils might provide some new insights into understanding the role that charcoal plays in decomposing organic N.
High-performance size-exclusion chromatography and chemiluminescent N detection (HPSEC-CLND) are techniques that are often used to detect the molecular sizes of soluble organic-N compounds in soils (Fujinari and Damon Citation1997; Moriizumi and Matsunaga Citation2011). Hot-water-soluble N in soils has been analysed to investigate the fate of soluble organic N in soils. The amount and distribution of molecular sizes of this fraction of N (hot-water-soluble) have been shown to be influenced by the application of compost to soils (Moriizumi and Matsunaga Citation2011). Thus, HPSEC-CLND analyses enable us to determine the stages of soluble organic- and inorganic-N conversion (mineralisation) following the application of organic N to soils.
Currently, little is known about how added charcoal and moisture conditions influence the mineralisation of soluble organic N to inorganic N in soils. It has been suggested in previous studies that the addition of charcoal to soils can either positively or negatively impact the mineralisation rates, depending on the condition of the charcoal and soil (Clough et al. Citation2013; El-Naggar et al. Citation2018; Zimmerman et al. Citation2011). In addition, the impact of mineralisation processes on soil N2O emissions is not well known. Studies of the mineralisation process are important to understand how plant available N (mineral N) is produced in soils after the application of organic N sources. Therefore, we aim to answer the following key questions in our study: (1) Does the presence of charcoal in soils influence the changes (over time) in the concentrations of various soluble organic-N fractions (relative to large, medium and small molecular compounds) during the decomposition of HV mulch? (2) Can charcoal influence N2O emissions during the early stages of HV decomposition in a soil? (3) How do variously sized soluble organic-N compounds correlate with the accumulation of inorganic N and N2O emissions from soils? We hypothesise that as the soluble organic-N fraction of HV mulch decomposes, the concentration of larger-molecular-size compounds declines over time, small-molecular-size organic-N compounds mineralise and N accumulates in soils as inorganic N. We also hypothesise that the fluctuation of N2O during the decomposition of HV mulch can be partly explained by the fluctuation in the amount of organic N present in soils.
2. Materials and methods
2.1. Materials used for the experiment
The soil that we used in our experiment was an Andosol obtained from an experimental field at the Institute for Agro-Environmental Sciences, NARO, Japan (36°01′N, 140°07′E). The soil characteristics were as follows: total C, 3.13%; total N, 0.26%; pH 5.9; bulk density, 0.70 g cm−3 (Uchida et al. Citation2013). An incubation experiment was performed to investigate the influence of the following three parameters on the soluble organic- and inorganic-N content in the soil: (1) HV residue, (2) charcoal made of rice husk and (3) soil moisture. At soil sampling (Marchin the year 2014), the field was fallow. The Andosol was air-dried at room temperature before beginning the experiment.
We grew HV (Vicia villosa Roth ssp. dasycarpa) from seed (Kaneko Seeds Co., Ltd., Gunma, Japan). HV plants were grown in a water culture for one month, harvested and air-dried prior to the experiment. The HV residue of the harvested leaves was 41% C and 8% N (C/N ratio = 5.06). After air drying, the leaves were finely cut (< 1 cm long pieces) before the application, with the mean weight of each piece equalling 3.70 ± 0.61 mg (one standard deviation, SD) ( = 3).
We prepared commercially available rice husk charcoal (Green Tech Inc., Tochigi, Japan) for the charcoal application treatment. This rice husk biochar was created at 200–300°C via slow pyrolysis (for 24 h). The resulting C/N ratio was 93 (total C = 41.7 ± 0.3%, total N = 0.45 ± 0.03%; = 3). The specific gravity of the rice husk biochar was 0.11 when loosely packed. When mixed with Milli-Q water (6 g of charcoal + 60 mL of water), the mean pH of the water was 8.78 ± 0.16 (
= 3).
2.2. Application of treatments and experimental setup
The Andosol was sieved through a 4 mm mesh and loosely packed into PVC pipe cores (inner diameter: 5.5 cm, height: 10 cm). Each core contained 19 g of air-dried soil. The bottom of each core was covered with a nylon mesh (0.6 mm mesh size). Then, the cores received one of eight treatments (with or without HV leaves applied to the soil surface × with or without charcoal × high or low soil moisture). Each treatment was comprised of three replicates. Then, we destructively sampled the soil cores at four different times. Therefore, 96 cores were examined in total for this part of the experiment (with or without HV leaf mulch × with or without amended charcoal × two moisture regimes × three replicates × four sampling intervals).
Our study simulated no-tillage or reduced-tillage systems wherein HV is used as green mulch (surface-applied) to provide organic N to soils. We applied HV to the soil surface at an application rate of 0.49 g core−1, equivalent to 28 g kg−1 of HV. Charcoal was incorporated into the soil cores at an application rate of 5.15% (by weight) (i.e. 0.95 g core−1). The soil bulk density was 0.53 g cm−3 without charcoal treatment and 0.49 g cm−3 with charcoal treatment. The cores were incubated at a constant ambient temperature of 23 ± 2°C.
The soil cores then received one of two moisture treatments (high or low moisture). For cores without charcoal, the soil moisture content was controlled at 34% water-filled pore space (WFPS) (low moisture) and 48% WFPS (high moisture), equivalent to 52% and 74% moisture (by weight), respectively. For core treatments with charcoal, the soil moisture content was held at 39% WFPS (low moisture) and 52% WFPS (high moisture), equivalent to 66% and 87% moisture (by weight), respectively. Because the soils were loosely packed, the high-moisture-treated soils were near field capacity, despite having relatively low (52%) WFPS, whereas the low-moisture soil cores held about 3.8 g core−1 less water compared to the high-moisture cores.
All soil cores were maintained at 25°C throughout the experiment. Throughout the experiment, we sprayed deionised water onto the soil surfaces once every two to three days; thus, the soil moisture fluctuated by ± 5% WFPS. The experimental period was 28 days. The measurements performed are described in the following sections.
2.3. Measurement of organic N
Organic-N measurements were performed on all treatment soils at 3, 11, 18 and 28 days after applying HV to the soil surfaces. Soluble organic N was extracted in water at 80°C (over a 16 h period) (3 g of soil to 50 mL of water) and stored at −30°C until evaluated.
We performed HPSEC-CLND to investigate the distribution of organic-N compounds for various molecular sizes using a serially connected YMC-Pack Diol 120 column (a silica-based column, 8 mm × 300 mm) and a high-performance liquid chromatography (HPLC) system (LC-4000; Jasco, Tokyo, Japan) equipped with a chemiluminescent nitrogen detector (ANTEK 8060; PAC, Houston, TX, USA). We used a 50 mM sodium acetate/acetic acid buffer (pH 7) as an eluent according to the study of Moriizumi and Matsunaga (Citation2011). The flow rate was 1 mL min−1, with 200 mL min−1 of the eluent flow split to the CLND detector. The CLND detector conditions were as follows: 50 mL min−1 argon, 250 mL min−1 oxygen, 25 mL min−1 ozone and 1050°C oven temperature. The injected volume was 100 μL for all samples.
We performed HPSEC-CLND measurements for each time increment ( = 4) and treatment (with one replicate each) to investigate the change over time for the variously sized hot-water-soluble organic-N compounds (i.e. with or without charcoal × with or without HV × two moisture treatments × four time increments;
= 32). In general, we observed three major organic-N peaks in the chromatograph data. We classified these peaks to represent three fraction sizes relative to their molecular sizes: L (large), M (medium) and S (small) [)]. The retention time for each fraction ranged from 5 to 7 min for L, from 8 to 10.5 min for M and from 12 to 13.5 min for S. This classification approach was applied in our study following methods used by Moriizumi and Matsunaga (Citation2011). The HPLC/CLND also showed a peak for NO3−-N occurring at around 11 min and a peak for NH4+-N peak occurring at about 15 min. Therefore, these time intervals were not included when assessing organic-N fractions. An NO3−-N solution with a known concentration was used as a standard, making relative comparisons among samples possible.
Figure 1. HPSEC-CLND chromatograms of hot-water-extractable soil organic matter. Samples were taken at four points in time (Days 3, 11, 18 and 28). The treatment combinations (with or without charcoal, with or without (±) HV and moisture [high or low]) are identified for each panel. Large (L), medium (M) and small (S) fractions of soluble organic-N peaks are identified in Panel (a)
![Figure 1. HPSEC-CLND chromatograms of hot-water-extractable soil organic matter. Samples were taken at four points in time (Days 3, 11, 18 and 28). The treatment combinations (with or without charcoal, with or without (±) HV and moisture [high or low]) are identified for each panel. Large (L), medium (M) and small (S) fractions of soluble organic-N peaks are identified in Panel (a)](/cms/asset/ced1c56c-5e94-41a5-8a02-4458bc7d705e/tssp_a_1624139_f0001_b.gif)
2.4. Measurement of inorganic N
We extracted soil inorganic N (NH4+-N and NO3−-N) from the sampled soils with 10% potassium chloride (KCl) (0.5 g of soil to 1.5 mL of KCl solution). The concentrations of the inorganic N were determined colourimetrically using a flow injection auto-analyser system (Aqua Lab, Nagoya, Japan). The soil water content was also measured by removing separate soil samples (approximately 5 g of fresh soil) and drying them at 105°C for more than 24 h. We used the obtained soil water content values to express concentrations as soil inorganic N per dry weight of soil.
2.5. Measurement of soil N2O emissions
A separate set of cores (two moisture conditions × with or without HV × with or without charcoal × three replicates) ( = 24 cores) were used to measure the N2O emissions, but the application rates of HV mulch and charcoal relative to the soil moisture regime were the same as in the previously described experiment. Because N2O emissions could be measured repeatedly in each set of cores, all cores (
= 24) for this part of the experiment could be used to measure changes over time (via different incubation periods). N2O emissions were measured two or three times per week over a period of one month. A headspace was created in each core by placing each core into a 1.5 L glass jar that was longer than the core and fitting the jar with a sealable, screw-on lid. After 30 min, we sampled both ambient air outside the jar and the headspace gas emissions inside the jar. We then withdrew gas samples of approximately 30 mL volume using a gas-tight syringe inserted through a septum embedded in the jar lids and transferred the evacuated gas into 15 mL vials. The gas samples in the vials were then analysed for N2O using a gas chromatograph equipped with an electron capture detector (ECD) (GC-2014; Shimadzu, Kyoto, Japan). The emission rates were expressed as N2O-N m−2 h−1.
Cumulative N2O-N emissions over the experimental period were calculated using a trapezoidal approach, wherein emission factors were calculated from the differences in cumulative N2O-N emission between the conditions with and without HV treatment divided by the total amount of N applied to soil cores as HV mulch (expressed as %).
2.6. Statistical and correlation analyses
We performed a three-way analysis of variance (ANOVA) to investigate the significant effects of HV, charcoal and moisture treatment on the inorganic-N concentration and size of organic-N peaks for each time increment and to evaluate the cumulative N2O emission data. When we observed significant effects in treatments and interactions, we compared the means using Tukey’s multiple comparison test to determine whether the means were significantly different. All data analyses were performed using R software (ver. 3.4.4).
3. Results
3.1. Molecular size distribution of soluble organic N
Soils with HV mulch showed three distinct peaks of hot-water-soluble organic N based on the HPSEC-CLND analyses (for fractions L, M and S) [–]. In the HPSEC-CLND analyses, the larger-molecular-size organic-N compounds appeared earliest in the chromatograph. Thus, the fractions were identified as L (large), M (medium) and S (small), according to the longer retention times for smaller molecular sizes for each peak. Organic-N peaks were ambiguous in soils without HV mulch [)–1(H)]. Thus, only results from the soils covered with HV leaves are described from here on.
For fraction S, we observed relatively large peaks from Day 3 to Day 11 for all soils covered with HV leaves [–]. The largest peak appeared on Day 3 without charcoal amendment, whereas peaks on Days 3 and 11 were similar in size to peaks from soils amended with charcoal. In soils with a low moisture content, the difference in peaks between treatments with and without charcoal was clearer than in those from soils subjected to high moisture. Within high-moisture soils, depletion of the S fraction occurred on Day 18 in charcoal-amended soils, but such depletion was not observed for soils without added charcoal.
The peak sizes of the L and M fractions were smaller than the peak size of fraction S. Peaks of fractions L and M were larger in soils amended with charcoal, particularly by Day 11, than in soils not amended with charcoal. As was the case for fraction S, the depletion of fractions L and M occurred by Day 18 in soils with both high moisture and charcoal.
3.2. Inorganic-N accumulation in soils during HV decomposition
The addition of HV leaves significantly ( < 0.001) increased the total soil inorganic-N concentrations throughout the experiment relative to the control (−HV) treatment (Table S1, ). With HV mulch, the total inorganic-N content in the soil peaked at 1,590 mg N kg−1 on Day 28. Averaged across the other treatments, this inorganic-N content was equivalent to 71% of the N contained in the applied HV mulch [ and ]. The soil inorganic-N concentrations in soils without HV mulch were < 100 mg N kg−1 throughout the experimental period [ and ]. The significant effects of the moisture and charcoal treatments on the total inorganic-N contents appeared only on Day 28. On Day 28, there were also significant interaction effects between HV mulch and moisture treatments and between HV mulch and charcoal treatments (Table S1). On Day 28, the total inorganic N in low-moisture soils covered with HV leaves was higher than the inorganic-N content in wetter soils covered with vetch mulch [ and ], whereas the relationship between total inorganic N and soil moisture was not clear in soils not covered with HV mulch [ and ]. In addition, in soils covered with HV mulch, charcoal-treated soils on Day 28 [] showed a significantly lower total N content compared to soils not amended with charcoal [)].
In soils covered with HV mulch, inorganic N was dominated by NH4+-N (i.e. NO3−-N concentrations were much lower) throughout the entire experimental period. For soils covered with HV mulch, soils not amended with charcoal [)] showed higher NH4+-N concentrations compared to soils amended with charcoal [)] on Days 18 and 28. In addition, on Day 28, soils covered with HV mulch had lower NH4+-N concentrations under high-soil-moisture treatment compared to under the low-moisture treatment.
Soil cores covered with HV mulch positively influenced the soil NO3−-N concentrations throughout the experimental period (28 days). We observed significant effects of factors other than HV mulch on Days 3 and 28 (Table S1). On Day 3, the NO3−-N concentrations were lower in soils amended with charcoal than in soils not amended with charcoal, particularly in soils covered with HV mulch. On Day 28, the NO3−-N concentrations were lower in soils with HV mulch and amended with charcoal than in soils with HV and without the charcoal [)].
3.3. N2O emissions
The amount of N2O-N relative to N from HV (emission factors, ) varied from 0.05% to 0.16% over the incubation period. There was a significant positive effect of HV mulch on cumulative N2O emissions, but the effect of charcoal amendment and soil moisture was not clear (). In soils with low moisture, emissions from soils covered with HV mulch appeared to be stronger in soils without charcoal than in soils with charcoal. In contrast, when the soil moisture was high, cumulative N2O emissions from soils covered with HV mulch appeared to be stronger in soils amended with charcoal than in soils not amended with charcoal.
Table 1. Cumulative nitrous oxide emissions and emission factors for soils covered with HV leaves during a four-week incubation period. The -values are based on a three-way ANOVA: *
< 0.05; ***
< 0.001. Errors = s.d. (
= 3)
When examining N2O emissions through time, they were found to be highest on Day 6 from high-moisture (saturated) soils amended with charcoal and covered with HV mulch [)]. In soils not amended with charcoal (and covered with vetch mulch), the N2O emission peak size was lower and occurred five days later (Day 11) compared to mulched soils amended with charcoal [)]. The N2O emission peaks over time for treatments with vetch mulch clearly differed between soils with high- and low-moisture conditions when amended with charcoal [)], but changes over time did not differ much between soils with different moisture conditions when the soils were not amended with charcoal [)].
4. Discussion
4.1. Decomposition of HV mulch and its relationships among different organic-N fractions
The accumulation of the S fraction of soluble organic N occurred early during the decomposition of HV (Days 3 and 11, ‒). This small molecular fraction consists of small peptides and amino acids, occurring within a retention time range exhibited by vitamin B12 (Moriizumi and Matsunaga Citation2011). Matsumoto (Citation2003) reported that amino acids and small-sized proteins, extracted from soils using a phosphate buffer, accumulate in soils during the decomposition of rice bran. Similarly, we found that small, soluble organic-N compounds, presumably derived from the decomposition of HV, accumulate in soils within days.
The largest peak of the smallest molecular fraction appeared at the earliest sampling period (within three days) in soil not amended with charcoal, whereas a relatively large peak was prolonged for an additional week (Days 3 and 11) in soils amended with charcoal, suggesting that the charcoal’s ability to adsorb organic N may be responsible. The adsorption of small-molecular-size organic N (amino acids) by aged charcoal made from chicken manure and paper sludge has been reported by Lin et al. (Citation2012). The rice husk charcoal that we used in our study is known to provide a large surface area (21–152 m2 g−1), and so the charcoal is also likely to be capable of adsorbing soluble substances in soils (Miura and Ikeoka Citation2008). Theeba et al. (Citation2012) stated that carboxylic and phenolic functional groups on charcoal are important in the adsorption process and that rice husk charcoal incorporates these functional groups.
It is well known that the addition of charcoal to soils often increases the soil’s water holding capacity (Novak et al. Citation2012). However, in drier soils, the availability of water to microbes may be lower in soils amended with charcoal because water is adsorbed within the charcoal intra-pores, thus making water inaccessible to microbes living outside the charcoal on soil particles (Liu et al. Citation2017). Thus, in our experimental soils, amending with charcoal under low-moisture conditions may have reduced the availability of water to microbes, thus reducing their capacity to decompose HV mulch.
We observed that the depletion of the S fraction occurred late in the decomposition process (Days 18 and 28) in high-moisture-content soils amended with charcoal, but not in soils not amended with charcoal, suggesting that the mineralisation of the S fraction likely occurs more rapidly in soils amended with charcoal than in soils not amended with charcoal. This more rapid mineralisation of N in response to charcoal amendments in soils has been previously reported by Nelissen et al. (Citation2012).
Throughout the course of our experiments (28 days), S fraction peaks were higher than the L and M () fraction peaks, which differed from a study by Moriizumi and Matsunaga (Citation2011) that showed soils with a history of compost applications having their highest peaks of the M fraction. The L fraction likely consists mostly of peptidoglycan (the main component of cell walls), whereas the M fraction is likely comprised of fulvic acid. However, the composition of organic N in soils varies widely, and there may be other types of organic N included in these fractions (Moriizumi and Matsunaga Citation2011). In our study, the soluble organic-N fractions derived from decomposing HV mulch were smaller than the molecular fractions derived from typical compost. This result agrees with the study of Agehara and Warncke (Citation2005), showing that the N released from organic N was more rapid from decomposing legumes than from compost. The accumulation and change in N release over time in the S fraction have not been previously reported. This small-sized organic-N fraction may be related to important components produced in the N cycling process during the early stages of green manure decomposition, such as molecules produced during N immobilisation/mineralisation and gaseous losses of N, including ammonia volatilisation. In our study, the accumulation of this small-sized organic-N fraction only appeared in soils for a short period of time as HV decomposed and did not remain in soils for very long.
The peak sizes of fractions L and M were larger when charcoaled rice husk was applied to soils, particularly by Day 11 ( and ), suggesting that some of the organic N derived from the decomposition of HV mulch is adsorbed by charcoal (a carbon energy source), thus forming large-molecule-size soluble organic matter.
The depletion of fractions L and M occurred late in the decomposition process (Days 18 and 28) under high-moisture conditions when soils were amended with charcoal, a situation similar to the depletion of the S fraction, which suggests that mineralisation might be accelerated in wet or moist soils amended with charcoal.
4.2. Inorganic-N accumulation during the decomposition of HV mulch
The rapid accumulation of inorganic N, particularly NH4+-N, occurring from Day 3 to Day 11 ( and ) in soils covered with vetch mulch, suggests that N mineralisation occurs during this time frame. This observation agrees with other studies showing that the peak of N mineralisation occurs within one week after applying HV mulch (Kuo and Sainju Citation1998; Rosecrance et al. Citation2000).
Figure 2. Changes over time in the soil NH4+-N (white bars) and NO3‒-N (grey bars) levels with and without charcoal for each sampling day (Days 3, 11, 18 and 28) and each soil treatment. The error bars represent 1 SD ( = 3). L_HV−: low moisture without hairy vetch; H_HV−: high moisture without hairy vetch; L_HV+: low moisture with hairy vetch; H_HV+: high moisture with hairy vetch. The concentration data (
-axis) for soils covered with hairy vetch mulch [Panels (a) and (b)] and without hairy vetch mulch [Panels (c) and (d)] differ in magnitude
![Figure 2. Changes over time in the soil NH4+-N (white bars) and NO3‒-N (grey bars) levels with and without charcoal for each sampling day (Days 3, 11, 18 and 28) and each soil treatment. The error bars represent 1 SD (n = 3). L_HV−: low moisture without hairy vetch; H_HV−: high moisture without hairy vetch; L_HV+: low moisture with hairy vetch; H_HV+: high moisture with hairy vetch. The concentration data (y-axis) for soils covered with hairy vetch mulch [Panels (a) and (b)] and without hairy vetch mulch [Panels (c) and (d)] differ in magnitude](/cms/asset/17aa8ce2-01f8-4928-86eb-f6b92948cde7/tssp_a_1624139_f0002_c.jpg)
The amount of inorganic N (mostly NH4+-N) in soils was relatively constant from Day 11 to Day 28 in soils covered with ()) HV mulch and amended with charcoal (regardless of the soil moisture content), whereas the inorganic-N levels gradually increased over time throughout the incubation period in soils not amended with charcoal ()). This difference in the rate of change over time suggests that NH4+-N will be more rapidly supplied to crops when charcoal and HV mulch are added to soils. Awad et al. (Citation2012) reported that charcoal application increases organic matter decomposition by increasing soil enzyme activity. They also observed higher soil respiration rates in response to the addition of charcoal. A positive influence of charcoal on the nitrification potential in soils has previously been reported (Prommer et al. Citation2014); however, in our study, the rates of NO3−-N accumulation did not differ between soils amended with charcoal and those not amended with charcoal. However, nitrification was likely not actively occurring in our experiment, potentially because of the high-soil-moisture content (on a volumetric basis) of our experimental soils. Further studies are needed to confirm the effects of soil moisture on the nitrification rates in treated soils.
NO3−-N was depleted by Day 28 in soils with charcoal and high-moisture content. This depletion might have been due to increased denitrification rates (requiring anaerobic conditions) because no depletion was observed for soils with low-moisture content and with charcoal (potentially used as an energy source for denitrification). This depletion of NO3−-N was unexpected because all our experimental soils were well aerated, even the wetter soils used for the high-moisture treatments. Because no depletion of NO3−-N was observed in soils without charcoal, it is possible that charcoal provides micro-hot spots for denitrification to occur. In their study, Cayuela et al. (Citation2013) suggested that the addition of charcoal to soils promotes denitrification because charcoal can act as an electron donor.
Increasing the soil moisture induced a decline in NH4+-N on Day 28 in soils mulched with HV. Because leaching of nutrients was not possible in our experiment, some of the NH4+-N might have been immobilised by microbes under the high-soil-moisture condition. In addition, nitrifier denitrification coupled with nitrification–denitrification might occur in soils with a high-moisture content; as a consequence, inorganic N might be emitted (as gases) without showing any accumulation of NO3−-N.
4.3. N2O emissions and cumulative N2O
N2O emission rates were higher in soils with HV mulch, especially in the early stage of our 28-day incubation period, than in soils without HV mulch, but these emission rates () were lower than the rates found by ShimotsuShimotsuma et al. (Citation2017), who applied similar charcoal and HV treatments under very wet (nearly saturated) conditions. ShimotsuShimotsuma et al. (Citation2017) observed approximately 1,500 mg N2O-N m−2 soil emitted during 45 days of HV decomposition, which is approximately 50 times higher than the values observed in our study. (The soils used in our study and in the study of ShimotsuShimotsuma et al. (Citation2017) were both Andosols collected from the same field.) Therefore, microbes involved in N2O emissions from decomposing HV are evidently moisture-sensitive. Our emission factors (0.05–0.16%) were much lower than the values reported by Alluvione et al. (Citation2009), wherein the mean annual emission factor for HV was reported as 2.3% per annum obtained from a field over a two-year period.
Figure 3. Changes in N2O fluxes in soils with HV mulch. Panels (a) and (b) track emissions with vetch mulch (with and without charcoal amendment), whereas Panels (c) and (d) track emissions without vetch mulch (with and without charcoal amendment). For each figure, low- and high-moisture treatments are shown as separate lines. The error bars represent 1 SD ( = 3)
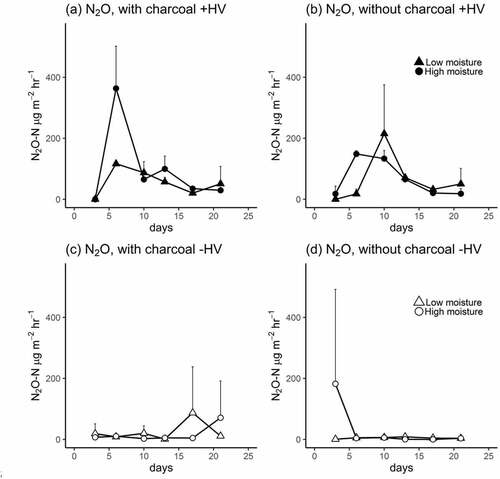
It has been suggested in some studies that the application of charcoal to soils results in reduced N2O emissions, a response to increased N2O reductase activity and/or improved soil aeration (Case et al. Citation2012; Singh et al., Citation2010; Taghizadeh-Toosi et al. Citation2011). In contrast, we did not observe any decline in the cumulative N2O emissions from soils after adding charcoal, perhaps because the mechanisms affecting N2O emissions (due to the surface application of plant residues) are more complex than those affecting emissions from synthetic fertilisers (which are relatively well studied). Plant residues provide both N and C to soils, which stimulate microbial activity. In addition, plant residues have to be mineralised before they can become substrates for microbes responsible for N2O emission pathways N2O, whereas synthetic fertilisers are themselves substrates. In addition, the diffusion of nutrients from surface-applied plant residues with soil depth must be considered when quantifying N2O emissions.
Peak N2O emissions in soils mulched with HV occurred five to six days sooner in soils amended with charcoal than in soils not amended with charcoal ( and ), suggesting that the substrates (C and N) derived from the decomposition of surface-applied plant residues become more quickly available for N2O-producing microbes in soils with charcoal. This speculation may explain the earlier appearance of the NH4+-N peak and the earlier depletion of NO3N in the charcoal-amended soils in our study.
Although the soil moisture did not influence the cumulative N2O emissions in our study, wetter soils possessed a higher N2O emission peak in soils amended with charcoal ()) and mulched with HV, suggesting that the microbes responsible for N2O emission peaks are more sensitive to moisture in the presence of charcoal.
4.4. Interaction among fluctuations of organic N, inorganic N and N2O during the decomposition of HV
We hypothesised that as soluble organic-N compounds in HV decompose, the molecular sizes of the compounds become reduced over time and N accumulates in soils as inorganic N. We found that the larger fractions (fractions L and M) of soluble organic N correlated with one another and that they correlated with NH4+-N concentrations (Fig. S1), suggesting that as HV mulch decomposes, the amount of NH4+-N that accumulates depends on the fluctuation of large- to medium-sized organic-N compounds, which is independent of the fluctuations of smaller molecular organic-N compounds. Ma et al. (Citation2017) found that amino acids (a major component of the small organic-N compounds in soils) mineralise within hours. Our sampling frequency was too low to catch this rapid rate of mineralisation, which may explain why it was difficult for us to link the fluctuations of the S fraction to other parts of the N cycle.
In a soil incubation study by McLain and Martens (Citation2005), the authors showed that amino acid mineralisation rates correlate with soil N2O emissions. In our study, the S fraction of organic N included pure amino acids, peptides and vitamins; however, because we used hot water to extract soluble organic N, the S fraction included substances from both inside and outside microbial cells (Ghani et al. Citation2003), which may explain why the S fraction that we measured did not correlate well with N2O emissions.
Hou et al. Citation2010) identified a large variation in N2O emissions during the decomposition of N-rich plant residues. For example, although the levels of N2O emitted during the decomposition of Chinese cabbage leaves in the field during harvesting were similar (in range) to our experimental results, the variability of N2O emissions between replicates was larger than the variability of emissions from control plots, owing to the heterogeneous decay patterns of cabbage leaves. The large variability that we measured in N2O emissions from Day 3 to Day 11 () and (B)) may have also been caused by the heterogeneous decomposition of HV mulch. Future experiments should focus on micro-scale N2O production in potential hot spots during decomposition, such as at the interface where mulch rests on the soil surface.
5. Conclusions
In this study, we examined the effects of various combinations of surface applications of HV (as mulch), amendments of charcoal to soils and soil moisture on the accumulation of organic N, inorganic N and N2O emissions. Our results suggest that small-molecular-size compounds of organic N rapidly accumulate in soils during the early decomposition of HV mulch but that charcoal amended to soils can moderate the accumulation rates of small organic-N compounds. Amending with charcoal did not reduce the cumulative N2O emissions during the decomposition process, but charcoal-treated soils was related to an earlier N2O emissions peak, suggesting that the charcoal accelerates the diffusion of decomposed materials to N2O-producing soil microbes. The fluctuations of medium-to-large organic-N compounds correlated with the fluctuations of ammonium-N concentrations in soils, but not for small nitrogenous organic compounds, suggesting that the small organic-N compounds rapidly turn over in the soils. In high-moisture soils amended with charcoal, nitrate-N was depleted near the end of the 28-day incubation period, suggesting the occurrence of denitrification in those soils. This means that that charcoal amendments to soils can reduce nitrate-N leaching from the soils, although relying on charcoal amendments is not the ideal approach for growing crops that require nitrate-N to thrive.
Supplemental_figures_and_tables_20190328.docx
Download MS Word (123.5 KB)Supplementary Material
Supplementary data for this article can be accessed here.
Additional information
Funding
References
- Agehara S, Warncke DD 2005: Soil moisture and temperature effects on nitrogen release from organic nitrogen sources. Soil Sci. Soc. Am.J, 69, 1844–1855. doi:10.2136/sssaj2004.0361
- Alluvione F, Bertoza C, Zavattaro L, Grignani C 2009: Nitrous oxide and carbon dioxide emissions following green manure and compost fertilization in corn. Soil Sci. Soc. Am.J, 74, 384–395. doi:10.2136/sssaj2009.0092
- Awad YM, Blagodatskaya E, Ok YS, Kuzyakov Y 2012: Effects of polyacrylamide, biopolymer, and biochar on decomposition of soil organic matter and plant residues as determined by 14C and enzyme activities. Euro. J. Of Soil Biol, 48, 1–10. doi:10.1016/j.ejsobi.2011.09.005
- Case SDC, McNamara NP, Reay DS, Whitaker J 2012: The effect of biochar addition on N2O and CO2 emissions from a sandy loam soil – the role of soil aeration. Soil Biol. Biochem., 51, 125–134. doi:10.1016/j.soilbio.2012.03.017
- Cayuela ML, Sanchez-Monedero MA, Roig A, Hanley K, Enders A, Lehmann J 2013: Biochar and denitrification in soils: when, how much and why does biochar reduce N2O emissions?. Sci Rep, 3, Article number. 1732. doi:10.1038/srep01732
- Clough TJ, Condron LM, Kammann C, Müller C 2013: A review of biochar and soil nitrogen dynamics. Agronomy, 3(2), 275–293. doi:10.3390/agronomy3020275
- Cobo JG, Barrios E, Kass DCL, Thomas RJ 2002: Decomposition and nutrient release by green manures in a tropical hillside agroecosystem. Plant Soil, 240, 331–342. doi:10.1023/A:1015720324392
- Ebelhar SA, Frye WW, Blevins RL 1984: Nitrogen from legume cover crops for no-tillage corn 1. Agronomy J, 76, 51–55. doi:10.2134/agronj1984.00021962007600010014x
- El-Naggar A, Lee SS, Awad YM, Yang X, Ryu C, Rizwan M, Rinklebe J, Tsang DCW, Ok YS 2018: Influence of soil properties and feedstocks on biochar potential for carbon mineralization and improvement of infertile soils. Geoderma, 332, 100–108. doi:10.1016/j.geoderma.2018.06.017
- Fujinari E, Damon MJ 1997: Determination of molecular mass distribution of food-grade protein hydrolyzates by size-exclusion chromatography and chemiluminescent nitrogen detection. J. Chromatogr. A, 763, 323–329. doi:10.1016/S0021-9673(96)00871-0
- Ghani A, Dexter M, Perrott KW 2003: Hot-water extractable carbon in soils: a sensitive measurement for determining impacts of fertilisation, grazing and cultivation. Soil Biol. Biochem., 35, 1231–1243. doi:10.1016/S0038-0717(03)00186-X
- Harmon ME, Franklin JF, Swanson FJ et al. 1986: Ecology of coarse woody debris in temperate ecosystems. Advances in Ecol. Res, 15, 133–302.
- Hou A, Tsuruta H, McCreary MA, Hosen Y 2010: Effect of urea placement on the time-depth profiles of NO, N2O and mineral nitrogen concentrations in an Andisol during a Chinese cabbage growing season. Soil. Sci. Plant. Nutr, 56, 861–869. doi:10.1111/j.1747-0765.2010.00519.x
- Kuo S, Sainju UM 1998: Nitrogen mineralization and availability of mixed leguminous and non-leguminous cover crop residues in soil. Biol Fert Soils, 26, 346–353. doi:10.1007/s003740050387
- Lehmann J, Joseph S 2009: Biochar for environmental management: an introduction. In Biochar for Environmental Management Science, Technology and ImplementationLehmann edited by Lehmann J, Joseph S, 1–12. Routledge: London. doi:10.4324/9780203762264.
- Lin Y, Munroe P, Joseph S, Kimber S, Van Zwieten L 2012: Nanoscale organo-mineral reactions of biochars in ferrosol: an investigation using microscopy. Plant Soil, 357, 369–380. doi:10.1007/s11104-012-1169-8
- Liu Z, Dugan B, Masiello CA, Gonnermann HM 2017: Biochar particle size, shape, and porosity act together to influence soil water properties. PLoS ONE, 12(6), e0179079. doi:10.1371/journal.pone.0179079
- Ma H, Pei G, Gao R, Yin Y 2017: Mineralization of amino acids and its signs in nitrogen cycling of forest soil. Acta Ecol. Sin, 37, 60–63. doi:10.1016/j.chnaes.2016.09.001
- Matsumoto S 2003: Identification of organic forms of nitrogen in soils and possible direct uptake by crops. Bull. Shimane Agric. Exp. Station, 34, 1–46.
- McLain JET, Martens DA 2005: Nitrous oxide flux from soil amino acid mineralization. Soil Biol. Biochem, 37, 289–299. doi:10.1016/j.soilbio.2004.03.013
- Miura Y, Ikeoka S 2008: Method of the water processing using carbonized chaff. Bull. Tottori Swine & Poult. Station, 58, 17–21.
- Moriizumi M, Matsunaga T 2011: Molecular weight separation of hot-water extractable soil organic matter using high-performance size exclusion chromatography with chemiluminescent nitrogen detection. Soil. Sci. Plant. Nutr, 57, 185–189. doi:10.1080/00380768.2011.565478
- Nelissen V, Rütting T, Huygens D, Staelens J, Ruysschaert G, Boeckx P 2012: Maize biochars accelerate short-term soil nitrogen dynamics in a loamy sand soil. Soil Biol. Biochem., 55, 20–27. doi:10.1016/j.soilbio.2012.05.019
- Novak JM, Busscher WJ, Watts DW et al. 2012: Biochars impact on soil-moisture storage in an Ultisol and two Aridisols. Soil Sci, 177, 310–320. doi:10.1097/SS.0b013e31824e5593
- Power JF, Doran JW, Koerner PT 1991: Hairy vetch as a winter cover crop for dryland corn production. J. Prod. Agric, 4(1), 62–66. doi:10.2134/jpa1991.0062
- Prommer J, Wanek W, Hofhansl F et al. 2014: Biochar decelerates soil organic nitrogen cycling but stimulates soil nitrification in a temperate arable field trial. PLoS ONE, 9(1), e86388. doi:10.1371/journal.pone.0086388
- Rosecrance RC, McCarty GW, Shelton DR, Teasdale JR 2000: Denitrification and N mineralization from hairy vetch (Vicia villosa Roth) and rye (Secale cereale L.) cover crop monocultures and bicultures. Plant Soil, 227, 283–290. doi:10.1023/A:1026582012290
- Ruffo ML, Bollero GA 2003: Modeling rye and hairy vetch residue decomposition as a function of degree-days and decomposition-days. Agron J, 95, 900–907. doi:10.2134/agronj2003.9000
- Sainju UM, Rahman S, Singh BP 2001: Evaluating hairy vetch residue as nitrogen fertilizer for tomato in soilless medium. Hort. Sci, 36(1), 90–93.
- Seo J-H, Meisinger JJ, Lee H-J 2006: Recovery of nitrogen-15–labeled hairy vetch and fertilizer applied to corn. Agronomy J., 98, 245–254. doi:10.2134/agronj2005.0013
- Shelton D, Sadeghi A, McCarty GW 2000: Effect of soil water content on denitrification during cover crop decomposition. Soil Sci, 164, 365–371. doi:10.1097/00010694-200004000-00007
- Shimotsuma M, Uchida Y, Nakajima Y, Akiyama H 2017: The effects of rice (Oryza sativa L. ssp. japonica) husk biochar on nitrogen dynamics during the decomposition of hairy vetch in two soils under high-soil moisture condition. Soil. Sci. Plant. Nutr, 63, 178–184. doi:10.1080/00380768.2017.1290498
- Sierra J 1997: Temperature and soil moisture dependence of N mineralization in intact soil cores. Soil Biol. And Biochem, 29, 1557–1563. doi:10.1016/S0038-0717(96)00288-X
- Singh BP, Hatton BJ, Singh B, Cowie AL, Kathuria A. 2010: Influence of biochars on nitrous oxide emission and nitrogen leaching from two contrasting soils. Journal Of Environment Quality, 39, 1224. doi: 10.2134/jeq2009.0138
- Six J, Conant RT, Paul EA, Paustian K 2002: Stabilization mechanisms of soil organic matter: implications for C-saturation of soils. Plant Soil, 241, 155–176. doi:10.1023/A:1016125726789
- Taghizadeh-Toosi A, Clough TJ, Condron LM, Sherlock RR, Anderson CR, Craigie RA 2011: Biochar incorporation into pasture soil suppresses in situ nitrous oxide emissions from ruminant urine patches. J. Environ. Qual, 40, 468–476. doi:10.2134/jeq2010.0419
- Theeba M, Bachmann RT, Illani ZI, Zulkefli M, Husni MHA, Samsuri AW 2012: Characterization of local mill rice husk charcoal and its effect on compost properties. Malaysian J. Soil Sci, 16, 89–102.
- Uchida Y, Rein IV, Akiyama H, Yagi K 2013: Contribution of nitrification and denitrification to nitrous oxide emissions in Andosol and from Fluvisol after coated urea application. Soil Sc. And Plant Nutr, 59, 46–55. doi:10.1080/00380768.2012.708646
- Zimmerman AR, Gao B, Ahn M-Y 2011: Positive and negative carbon mineralization priming effects among a variety of biochar-amended soils. Soil Biol. Biochem., 43, 1169–1179. doi:10.1016/j.soilbio.2011.02.005