ABSTRACT
Mangrove ecosystems play an important role in carbon (C) accumulation in tropical and subtropical regions. Below-ground deep anoxic soil is especially important for C accumulation. However, quantitative data on below-ground soil C stocks in mangrove ecosystems are lacking compared with data on above-ground biomass. In addition, soil C accumulation processes in mangrove ecosystems have not been sufficiently clarified. In this study, we quantified soil C stocks and focused on the mass of fallen litter and below-ground roots, which are produced by tree and that may directly influence soil C stocks in a mature subtropical mangrove in the estuary of Fukido River, Ishigaki Island, southwestern Japan. The principal species in this study site were Bruguiera gymnorhiza and Rhizophora stylosa, and total above-ground biomass at the site was 80.7 ± 1.3 (mean ± SD) Mg C ha−1 over the period from 2014 to 2016. Litter was collected in six litter traps from May 2013 to November 2016, it ranged from 7.8 to 11.5 Mg C ha−1, with the major proportion of litter being from foliage (leaves and stipules). The root C density at 90-cm depth was 27.1 ± 11.3 Mg C ha−1. The soil C stock in the mangrove forest at a depth of 90 cm at the study site was 251.0 ± 34.8 Mg C ha−1, and it seems to be lower value in the tropical region but it to be higher in subtropical East Asian mangrove sites. Dead roots, especially dead fine roots, but not fallen litter, were significantly positively correlated with soil C stocks. The δ13C values obtained from soils ranged from −29.3‰ to −27.0‰; these values are consistent with those for below-ground fine roots. These results strongly suggest that dead fine roots could be a main factor controlling soil C stocks at this study site.
1. Introduction
Mangrove ecosystems have a high potential to sequester large amounts of carbon (C) from the atmosphere (Siikamäki, Sanchirico, and Jardine Citation2012; Saintilan et al. Citation2013). The net ecosystem production (NEP) values of mangrove ecosystems (e.g., Barr et al. Citation2010; Poungparn et al. Citation2012) are more than fourfold greater than those of general terrestrial forest ecosystems, where NEP values from the tropics to the boreal zone are approximately 2 to 3 Mg C ha−1 (Kato and Tang Citation2008). In support of NEP data, mangrove ecosystems have been identified as some of the most C-rich forest ecosystems on Earth (Bouillon, Connolly, and Lee Citation2008). For example, in the Indian Ocean–Pacific region, which has the greatest distribution of mangrove forests in the world, mangrove ecosystems reportedly stored 1023 Mg C ha−1 (415 to 2203 Mg C ha−1) on average, taking into account both above- and below-ground storage. This amount of C stocks per area is enormous, even when compared with that of other terrestrial forest ecosystems (Nellemann et al. Citation2009; Donato et al. Citation2011). Although mangroves account for less than 1% of total tropical forest area, they sequester approximately 3% of all C sequestered by tropical forests (Alongi Citation2014).
The huge amount of C stocks in mangrove ecosystems is due to the high C burial rate especially in deep anoxic soils (Alongi Citation2014; Donato et al. Citation2011). A study of 25 stands of mangrove forests distributed in the Indian Ocean–Pacific region reported that 49% to 98% of total ecosystem C was accumulated below ground in soil at depths of 50 to 300 cm (Donato et al. Citation2011). Soil C stocks to a depth of 100 cm account for 62% to 88% of total ecosystem C in Yingluo Bay, China (Wang et al. Citation2013). Thus, soil plays a significant role in C sequestration in mangrove ecosystems. However, many data on soil C stocks relied heavily on the use of a pedotransfer function for estimating bulk density and were missing soil C content at depths greater than 50 cm (Atwood et al. Citation2017). Furthermore, data regarding soil C stocks and accumulation processes in mangrove ecosystems have been reported mainly from the tropics, and only limited data exist for regions in subtropical East Asia, including Japan, which is at a relatively high latitude (>20°N), although mangroves are widely distributed in the area due to a monsoon climate.
Soil C accumulation in mangrove ecosystems may be strongly influenced by litter produced in the mangroves, which usually consists of fallen leaves, dead fine roots, and fallen woody debris (Alongi Citation1998; Ono et al. Citation2015). In particular, dead fine roots in mangroves may strongly contribute to soil C accumulation, even at depths greater than 50 cm (Ono et al. Citation2015). However, the effects of those litters, particularly dead fine roots, on below-ground soil C stocks have not been sufficiently elucidated. To clarify these effects, we need not only to investigate the details of the relationship between root mass (live and dead) and soil C stocks but also to determine the primary source of soil C stocks. Unlike terrestrial forest ecosystems, mangroves are constantly affected by tidal fluctuations (Yong et al. Citation2011). Therefore, we need to consider sources of soil C stocks other than litter, such as particulate and dissolved organic matter flowing from the ocean, as well as benthic algae (Bouillon, Connolly, and Lee Citation2008). In this situation, analysis of the C stable isotope ratio (δ13C) is one of the most powerful tools for determining the origin of soil C in mangrove ecosystems (e.g., Kennedy et al. Citation2004; Prasad and Ramanathan Citation2009).
Thus, we need to understand the levels of soil C stocks and soil C accumulation processes in mangrove ecosystems. We hypothesized that dead roots (especially fine roots) are a main source of soil C stocks in mangrove ecosystems. To test our hypothesis, we estimated the amount of fallen litter, below-ground roots, and soil C stocks to a depth of 90 cm, and we also measured δ13C to clarify the primary origin of soil C stocks in a subtropical mangrove ecosystem in the estuary of the Fukido River, Ishigaki Island, southwestern Japan. In addition, we compared the soil C stock levels at this study site with those of other mangrove sites.
2. Materials and methods
2.1. Study site
The study site was located in the estuary of the Fukido River on Ishigaki Island, southwestern Japan (24°29´N, 124°13´E). The average annual precipitation (1980–2000) is 2169 mm and is distributed throughout the year under the Asian monsoon climate. The annual mean temperature was 23.8°C, and the mean temperatures in January (the coldest month) and July (the hottest month) are 18.3°C and 28.8°C, respectively. Climatic data were collected at a weather station (Ibaruma, 24°30.5´N, 124°16.8´E; 15 m asl) located approximately 5 km from the study area. At high tide, the ground surface of the mangroves is immersed in sea water, whereas at low tide, the ground surface is exposed to air. In general, these tides are repeated twice a day at 12-h intervals. The mangroves extend from the estuary to the land for approximately 13 ha (Nakasuga, Ôyama, and Haruki Citation1974; Nakaza et al. Citation2011). A 0.64-ha study plot (80 m × 80 m) was established in the estuary in May 2013. The study plot was divided into 64 subplots of 100 m2 each by compass surveying ().
Figure 1. Schematic of the study plot (80 m × 80 m) in January 2014, subtropical mangrove in the estuary of Fukido River, Ishigaki Island, Southwestern Japan. Gray and white circle indicate the distribution of all living tree stems in each diameter class of the two mangrove species (B. gymnorhiza and R. stylosa). White triangle and gray triangle (P1 to P6) indicate the places of litter traps and selected six litter traps, respectively. Dashed line shows the course of the river
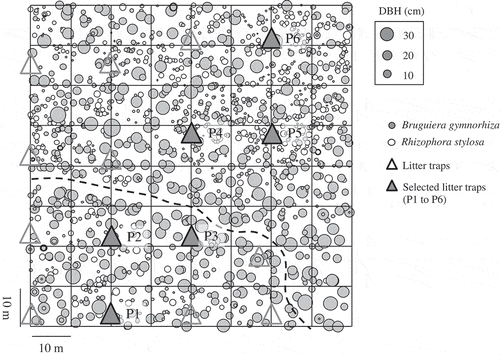
2.2. Outline of above-ground trees in this study site
The distribution of all living tree stems in each diameter class of mangroves in January 2014 is shown in . A detailed description of the above-ground trees and litterfall dynamics was previously reported (Ohtsuka et al. Citation2019). Briefly, the plot contained 2323 and 594 stems (ha−1) greater than 1.3 m in height of the two mangrove species Bruguiera gymnorhiza and Rhizophora stylosa, respectively, in January 2014. The basal area of the mangrove stand was 35.9 m2 ha−1, which included 29.8 m2 ha−1 (83%) and 6.1 m2 ha−1 (17%) for B. gymnorhiza and R. stylosa, respectively. The average diameters of B. gymnorhiza and R. stylosa were 10.9 cm and 11.2 cm, respectively. The total above-ground biomass in the plot was 161.3 ± 2.7 (mean ± SD) Mg ha−1 (80.7 ± 1.3 Mg C ha−1) over the 3 years (from 2014 to 2016). Litterfall in this mangrove study site over the 3 years (from 2014 to 2016) peaked around September for all successive years. Foliage is the main component, except in September 2015 when woody materials predominated litter production. The net primary production of the annual foliage over the 3 years (from 2014 to 2016) was 7.56 ± 0.99 (mean ± SD) Mg ha−1 y−1.
In this study site, R. stylosa logs were scattered across the plot, of which, a few juveniles were recorded with unimodal diameter distribution of present stems. Therefore, R. stylosa may have dominated the forest in the study plot in its early stages, before it succeeded to a B. gymnorhiza-dominated stage (Ohtsuka et al. Citation2019).
2.3. Litterfall
Sixteen litter traps (each 0.5 m2 in surface area) were set at more than 1 m above-ground in the 0.64-ha study plot in May 2013. To determine the relationship between litterfall and below-ground soil C stocks, we randomly selected six litter traps (P1 to P6) from 16 litter traps (). Litterfall was collected approximately once every 2 months from May 2013 to November 2016. It was classified as foliage (leaves and stipules), reproductive organs (flowers and seeds), woody materials (bark and twigs), and other (e.g., insect excrement) and was oven-dried at 60°C for more than 48 h to a constant mass and weighed. The litterfall was calculated converting mass to C by multiplying by 0.45 (Kauffman and Donato Citation2012).
2.4. Below-ground root and soil sampling
To estimate the density of below-ground roots, we collected 18 core samples from six points (three replicants) near the six litter traps (P1 to P6), using PVC tubes (diameter 5 cm × depth 30 cm) till 90-cm depth (). In this study, we defined roots with a diameter of <2 mm as fine roots, and those with a diameter of ≧2 mm as coarse roots (Poungparn et al. Citation2015). The roots were washed on a stainless steel mesh (0.5 mm in size) using tap water and manually sorted between living and dead roots according to their color and firmness (Poungparn et al. Citation2015). Living roots are typically white or yellow in color and float in water (Poungparn et al. Citation2015). Each root sample was divided into four fractions: live coarse roots (≧2 mm), live fine roots (<2 mm), dead coarse roots (≧2 mm), and dead fine roots (<2 mm). Each root sample was oven-dried at 60°C for more than 48 h and then weighed. The root C density was calculated converting mass to C by multiplying by 0.39 (Kauffman and Donato Citation2012).
Soil samples were collected using a handy geoslicer (upper-lower width 8.5–9.5 cm × length 100 cm × thickness 3 cm) at approximately the same locations as the litter traps where the root cores were sampled. Each geoslicer sample was cut into 10-cm sections, then air-dried with light crushing, and passed through a 2-mm sieve; as much as possible of the free organic residues (e.g., live and dead roots) were removed with tweezers before sieving. Subsequently, the soil was dried at 80°C for more than 48 h. For each oven-dried soil sample, soil bulk density was calculated as the ratio of soil dry weight to the volume of the soil of the slice obtained using the geoslicer. Each soil sample was then finely pulverized. After inorganic C was removed by HCl treatment (Midwood and Boutton Citation1998), the soil was analyzed for C content and stable C isotope ratio (δ13C).
2.5. Soil analyses
Soil C contents were measured with an NC analyzer (Sumigraph NC-22F, Sumika Analysis Service, Ltd). The soil C stock (Mg C ha – 1) was calculated from the soil C content and the bulk density.
For stable C isotope ratios (δ13C), the same soil samples were used that were used for measurement of soil C content. We also used the dried and pulverized foliage from R. stylosa and B. gymnorhiza and several fine root samples (predominantly dead fine roots). The δ13C value of each soil sample was measured by an elemental analysis/stable isotopic ratio mass spectrometer (EA/IRMS) continuous flow system (Thermo Scientific, USA). The δ13C value was calculated from the following formula:
δ13C = [Rsample/Rstandard − 1] × 1000,
where Rsample and Rstandard represent the 13C:12C ratio of the sample and standard, respectively. L-α-Alanine (δ13C = −19.60‰) and glycine (δ13C = −28.70‰) was used as a calibrated standard. The measurement was repeated until the standard deviation became <0.2‰.
3. Results
3.1. Litterfall
Litterfall from May 2013 to November 2016 at litter traps P1 to P6 is shown in . Litterfall ranged from 782.2 to 1146.0 g C m−2 (7.8 to 11.5 Mg C ha−1), and litterfall was 915.4 ± 115.5 (mean ± SD) g C m−2 (9.2 ± 1.2 Mg C ha−1). Foliage was the main component at all points, accounting for 82.9% to 91.3%. The ‘other’ fraction was the second most frequent fraction.
3.2. Below-ground roots
The root C densities at each sampling point are shown in . The root C densities for each 30-cm depth tended to vary depending on the sampling point. Overall, live (coarse and fine) root density showed a trend to decrease with depth, however, not for dead roots. The root C density at 90-cm depth was 27.1 ± 11.3 (mean ± SD) Mg C ha−1 (range, 12.6 to 41.3 Mg C ha−1). Live coarse roots or dead fine roots were the main root fractions, depending on the sampling point, with percentages ranging from 22.6% to 70.8% and from 19.1% to 51.6% of total below-ground root, respectively.
3.3. Soil c stocks
shows the soil C content, bulk density, and soil C density for each 10-cm depth for each sampling point. When viewed throughout, the soil C content for each 10-cm depth was 2.0% to 7.1%; no regular trend corresponding to the depth was found for any point. The bulk density and soil C density at each sample were 0.5 to 0.8 g cm−3, and 16.5 to 44.2 mg cm−3, respectively. The soil C content showed a significant negative correlation with bulk density (r = −0.387, P < 0.005, n = 54). Soil C density (mg cm−3) for each 10-cm increase in depth was a very similar trend to that of soil C content (%).
Figure 4. Soil C content (%), bulk density (g cm−3), and soil C density (mg cm−3) at every depth of 10 cm for each point from P1 to P6
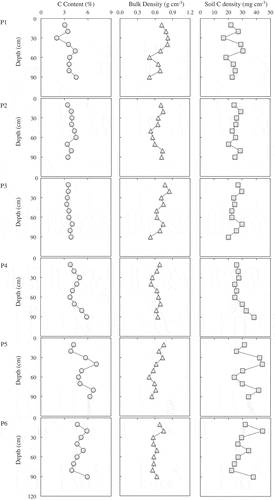
The soil C stocks estimated at each point among soil depths up to 90 cm are shown in . The soil C stocks at each point were 213.7 to 303.9 Mg C ha−1, and the soil C stock were 251.0 ± 34.8 (mean ± SD) Mg C ha−1. The root C densities at 90-cm depth in each sampling point account for 5.9% to 17.9% of total soil C stocks.
3.4. Stable c isotope ratio (δ13C)
shows the results of the measurement of δ13C values for soil for each 10-cm depth at each point. On the whole, there was a difference of only about 2‰ for each, that was from −29.3‰ to −27.0‰. The value was slightly higher in the deeper layers than in the surface layers for every point. The δ13C values of leaf litter from R. stylosa and B. gymnorhiza were −30.8 ± 0.8 (mean ± SD)‰ and −31.0 ± 0.6‰, respectively, and the value was −28.7 ± 0.5‰ for fine roots. These results indicate that the δ13C values for soil are almost the same as the values for mangrove fine roots.
Figure 6. δ13C values (‰) for soil every 10 cm in depth at each sampling point from P1 to P6. Each dotted line indicates the mean δ13C values for leaf of two mangrove species (B. gymnorhiza and R. stylosa) and fine root measured in this study (n = 3), and microalgae, macroalgae, and seagrasses are from literature (Bouillon, Connolly, and Lee Citation2008)
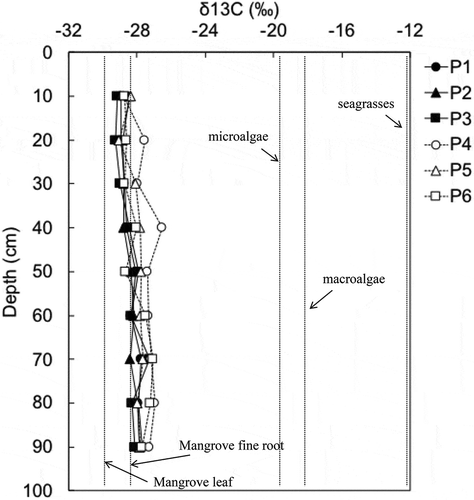
4. Discussion
4.1. Soil c stock in mangrove ecosystems
Although the amount of data on soil C stocks in mangrove ecosystems published in papers is gradually increasing, the quantification of soil C stocks in mangrove ecosystems has been impeded by a lack of concurrent data on soil C content and bulk density, particularly in deep layers (Donato et al. Citation2011). Recently, Atwood et al. (Citation2017) reported global patterns in mangrove soil C stocks to a depth of 100 cm by meta-analysis using data (n = 1230) from 48 countries encompassing 88% of the global mangrove area; however, unfortunately, 55% of data relied heavily on the use of a pedotransfer function for estimating bulk density, and 50% of the countries were missing estimates of soil organic C content for depths greater than 50 cm, which included almost all Japanese mangrove data (e.g., Mfilinge, Atta, and Tsuchiya Citation2002; Sharma et al. Citation2014).
In this study, we estimated soil C stocks in mangrove ecosystem to a depth of 90 cm. We found that the mangrove soil C stock at the study site was 251.0 ± 34.8 (mean ± SD) Mg C ha−1 (range, 213.7 to 303.9 Mg C ha−1) (). Converting our soil C stocks data per 100-cm depth account for 278.8 ± 38.7 Mg C ha−1. According to other data in mangrove ecosystems from tropical and subtropical regions, mangrove soil C stocks varied from 57.3 to 2116 Mg C ha−1 (667.7 Mg C ha−1 in average) at 48- to 365 cm depth (). We also estimated the soil C stocks by converting to a depth of 100 cm; the estimates were from 57.3 to 726.6 Mg C ha−1 (382.3 Mg C ha−1 on average) (). Overall, the soil C stock in our study site seems to be lower value in tropical region (<20°N and S) but it to be higher in the northern limit of mangrove distribution in subtropical East Asia.
Table 1. Pearson’s correlation coefficient between soil C stocks up to 90 cm depth (Mg C ha−1) and litterfall (Mg C ha−1) and below-ground fine root density up to 90 cm depth (Mg C ha−1) among sampling points (n = 6)
Table 2. Above-ground biomass and below-ground soil C stocks in mangrove ecosystems along different latitude
4.2. Relationship between soil c stock and fallen litter and roots in mangrove ecosystems
Donato et al. (Citation2011) reported that soil C stocks were positively, but weakly, correlated with above-ground biomass at 25 sites in the Indian Ocean–Pacific region. Recent meta-analysis by Sanders et al. (Citation2016) and Atwood et al. (Citation2017) also showed the lack of a strong relationship between soil C stocks and above-ground mangrove biomass, which progressively increases toward the tropics. Thus, the relationship between above-ground biomass and soil C stock has been not cleared because it may be extremely difficult to detect it directly on spatial scale. In this study, therefore, we focused on the mass of fallen litter and below-ground roots produced by tree, which may directly influence soil C stocks.
The soil C stocks at this study site varied greatly depending on the sampling point (). In addition, soil C stocks for each 10-cm depth seem to be constant with increasing depth up to 90 cm at all sampling points (). Using Pearson’s correlation coefficient, the relationship between soil C density and both litterfall and below-ground root density among the sampling points was analyzed (), but neither total litter nor foliage (the major litter fraction at the present study site) were significantly positively correlated with soil C stock. Ono et al. (Citation2006) reported that leaf litter on a Micronesian mangrove forest floor did not directly contribute much to C accumulation in below-ground soil because of the rapid decomposition of litter and/or its export to the ocean by daily tidal inundation. We could not observe a fallen litter layer, especially of foliage, on this forest floor, but it existed in the downstream area out of the study plot. This is probably the same situation that occurred in the Micronesian mangrove, and fallen litter may be not be an important source of soil C at our site.
shows the Pearson’s correlation coefficients between soil C stock and each root fraction density for each 30-cm depth. Soil C stock showed significant positive correlations (P < 0.05) with dead roots and dead fine roots, but not live roots, live coarse roots, live fine roots, and dead coarse roots. The density of dead fine roots, even in deeper layers, is almost the same as that in surface layers except for P4 and P5 (). Poungparn et al. (Citation2015) reported a very high production rate of fine roots in Rhizophora spp, which might be the source of fresh organic C in deeper soil in the estuary of the Trat River in eastern Thailand. Ono et al. (Citation2015) also suggested that large amounts of mangrove roots penetrate to at least 80 cm depth of soil by 14C dating in a Micronesian mangrove. These results agreed well with our result, and it strongly suggests that dead roots, especially dead fine roots, directly contribute to C accumulation in soil, not only in the surface layer but also in deeper layers to at least 90 cm at this study site.
Figure 7. Linear correlation coefficients (Pearson’s correlation coefficient) between soil C stock and each root fraction C density for each 30-cm depth (n = 18). Asterisks (*) indicates significance level at P < 0.05
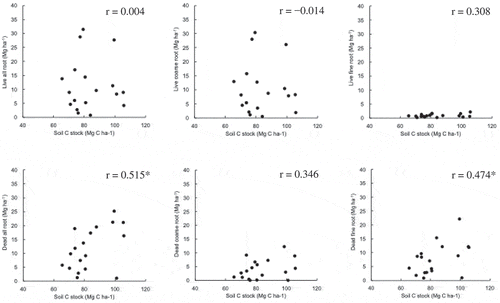
According to Bouillon, Connolly, and Lee (Citation2008), mangrove leaves, microalgae, macroalgae, and seaweeds are particularly important sources of soil organic C in mangroves. The median δ13C values for these sources were −28.1% (n = 495), −20.2% (n = 22), −18.9% (n = 41), and −12.1% (n = 43), respectively, with a large difference between the δ13C value of mangroves and the δ13C values of other major sources (Bouillon, Connolly, and Lee Citation2008). At our study site, the δ13C values of R. stylosa leaves, B. gymnorhiza leaves, and a mixture of R. stylosa and B. gymnorhiza fine roots were −30.8‰, −31.0‰, and −28.7‰, respectively (). All δ13C values of soils measured in this study were around −28.7‰ (from −29.3‰ to −27.0‰) (). Thus, we can strongly speculate that almost all soil C at this study site is derived from tree biomass, especially C in fine roots. The contributions of microalgae, macroalgae, and seaweeds are extremely small. When the root density results are included, dead fine roots may be a major factor controlling soil C stocks at this study site.
5. Conclusions
In this study, we estimated the soil C stock and the relationship between soil C stock and fallen litter and below-ground roots in a mature mangrove forest in the estuary of the Fukido River, Ishigaki Island, in southwestern Japan. The soil C stocks to a depth of 90 cm, stored 251.0 ± 34.8 (mean ± SD) Mg C ha−1. Mangrove ecosystems in the estuary of the Fukido River stored relatively low soil C compared with those in tropical region but higher soil C in the northern limit of mangrove distribution in subtropical East Asian mangrove sites. The soil C stocks were significantly positively correlated with dead roots, especially dead fine roots to a depth of 90 cm. The δ13C values of soil C (from −29.3‰ to −27.0‰) and fine roots (−28.7 ± 0.5‰) also agreed well with these relationships. These results strongly suggest that dead fine roots could be a main factor controlling soil C stocks at this study site.
Acknowledgments
We thank the members of Laboratory of Soil Science, The University of Shiga Prefecture and Laboratory of Vegetation Function, River Basin Research Center, Gufi University for their assistance with field survey. The study was supported by JSPS KAKENHI Grant Number 15K12186, and by The Sumitomo Foundation, Grant for Environmental Research Projects, 2014.
Disclosure statement
No potential conflict of interest was reported by the authors.
Additional information
Funding
References
- Adame, M. F., N. S. Santini, C. Tovilla, A. Vázquez-Lule, L. Castro, and M. Guevara. 2015. “Carbon Stocks and Soil Sequestration Rates of Tropical Riverine Wetlands.” Biogeoscience 12: 3805–3818. doi:10.5194/bg-12-3805-2015.
- Alongi, D. M. 1998. Coastal Ecosystem Processes. New York, USA: CRC Press, 419.
- Alongi, D. M. 2014. “Carbon Cycling and Storage in Mangrove Forests.” Annual Review of Marine Science 6: 195–219. doi:10.1146/annurev-marine-010213-135020.
- Atwood, T. B., R. M. Connolly, H. Almahasheer, P. E. Carnell, C. M. Duarte, C. J. Ewers Lewis, X. Irugoien, et al. 2017. “Global Patterns in Mangrove Soil Carbon Stocks and Losses.” Nature Climate Change 7: 523–528. doi:10.1038/nclimate3326.
- Barr, J. G., V. Engel, J. D. Fuentes, J. C. Zieman, T. L. O’Halloran, T. J. Smith III, and G. H. Anderson. 2010. “Controls on Mangrove Forest-atmosphere Carbon Dioxide Exchanges in Western Everglades National Park.” Journal of Geophysical Research 115: G02020. doi:10.1029/2009JG001186.
- Bouillon, S., R. M. Connolly, and S. Y. Lee. 2008. “Organic Matter Exchange and Cycling in Mangrove Ecosystems: Recent Insights from Stable Isotope Studies.” Journal of Sea Research 59: 44–58. doi:10.1016/j.seares.2007.05.001.
- Donato, D. C., J. B. Kauffman, D. Murdiyarso, S. Kurnianto, M. Stidham, and M. Kanninen. 2011. “Mangroves among the Most Carbon-rich Forests in the Tropics.” Nature Geoscience 4: 293–297. doi:10.1038/ngeo1123.
- Fujimoto, K., A. Imaya, T. Tabuchi, S. Kuramoto, H. Utsugi, and T. Murofushi. 1999. “Belowground Carbon Storage of Micronesian Mangrove Forests.” Ecological Research 14: 409–413. doi:10.1046/j.1440-1703.1999.00313.x.
- Ishihara, S., K. Fujimoto, K. Masahiro, R. Watanabe, and S. Tanaka. 2004. “Relationship between Mangrove Vegetation and Micro-topography, and Carbon Storage of Kandelia Candel (L), Druce Forests in Amami-Ohshima Island, Southwestern Japan.” The Japanese Society of Forest Environment 46: 9–19. (In Japanese with English summary).
- Kato, T., and Y. Tang. 2008. “Spatial Variability and Major Controlling Factors of CO2 Sink Strength in Asian Terrestrial Ecosystems: Evidence from Eddy Covariance Data.” Global Change Biology 14: 2333–2348. doi:10.1111/gcb.2008.14.issue-10.
- Kauffman, J. B., and D. C. Donato. 2012. Protocols for Measurement, Monitoring and Reporting of Structure, Biomass and Carbon Stocks in Mangrove Forests. Bogor, Indonesia: CIFOR.
- Kennedy, H., E. Gacia, D. P. Kennedy, S. Papadimitriou, and C. M. Duarte. 2004. “Organic Carbon Sources to SE Asian Coastal Sediments.” Estuarine, Coastal and Shelf Science 60: 59–68. doi:10.1016/j.ecss.2003.11.019.
- Khan, N. I., Md, R. Suwa, and A. Hagihara. 2007. “Carbon and Nitrogen in a Mangrove Stand of Kandelia Obovata (S., L.) Yong: Vertical Distribution in the Soil-vegetation System.” Wetland Ecology and Management 15: 141–153. doi:10.1007/s11273-006-9020-8.
- Mfilinge, P. L., N. Atta, and M. Tsuchiya. 2002. “Nutrient Dynamics and Leaf Litter Decomposition in a Subtropical Mangrove Forest at Oura Bay, Okinawa, Japan.” Trees 16: 172–180. doi:10.1007/s00468-001-0156-0.
- Midwood, A. J., and T. W. Boutton. 1998. “Soil Carbonate Decomposition by Acid Has Litter Effect on δ13C of Organic Matter.” Soil Biology and Biochemistry 30: 1301–1307. doi:10.1016/S0038-0717(98)00030-3.
- Nakasuga, T., H. Ôyama, and M. Haruki. 1974. “Studies on the Mangrove Community, I. The Distribution of the Mangrove Community in Japan.” Japanese Journal of Ecology 24: 237–246. (In Japanese with English summary).
- Nakaza, E., Y. Watanabe, D. Kawahara, T. Iribe, and R. Savou. 2011. “Characteristics Changes in Mangrove Forest in Ishigaki Island.” Journal of JSCE 67(2): 732–737. (In Japanese with English summary).
- Nellemann, C., E. Corcoran, C. M. Durante, L. Valdes, C. Deyoung, L. Fonseca, and G. Grimditch. 2009. Blue Carbon. United Nations Environment Programme, GRID-Arendal, 78. New York, USA: United Nations Environmental Program.
- Ohtsuka, T., M. Tomotsune, V. Suchewaboripont, Y. Iimura, M. Kida, S. Yoshitake, M. Kondo, and K. Kinjo. 2019. “Stand Dynamics and Aboveground Net Primary Production of a Mature Subtropical Mangrove Forest on Ishigaki Iskland, South-western Japan.” Regional Studies Marine Science 27: 100516. doi:10.1016/j.rsma.2019.100516.
- Ono, K., K. Fujimoto, M. Hiraide, S. Lihpai, and R. Tabuchi. 2006. “Aboveground Litter Production, Accumulation, Decomposition, and Tidal Transportation of Coral Reef-type Mangrove Forest on Pohnpei Islad, Federated States of Micronesia.” Tropics 15 (1): 75–84. doi:10.3759/tropics.15.75.
- Ono, K., S. Hiradate, S. Morita, M. Hiraide, Y. Hirata, K. Fujimoto, R. Tabuchi, and S. Lihpai. 2015. “Assessing the Carbon Composition and Sources of Mangrove Peat in a Tropical Mangrove Forest on Pohnpei Islad, Federated States of Micronesia.” Geoderma 245–246: 11–20. doi:10.1016/j.geoderma.2015.01.008.
- Poungparn, S., T. Charoenphonphakdi, T. Sangtien, and P. Patanaponpaiboon. 2015. “Fine Root Production in Three Zones of Secondary Mangrove Forest in Eastern Thailand.” Trees. doi:10.1007/s00468-015-1220-5.
- Poungparn, S., A. Komiyama, T. Sangteian, C. Maknual, P. Patanaponpaiboon, and V. Suchewaboripont. 2012. “High Primary Productivity under Submerged Soil Raises the Net Ecosystem Productivity of a Secondary Mangrove Forest in Eastern Thailand.” Journal of Tropical Ecology 28: 303–306. doi:10.1017/S0266467412000132.
- Prasad, A. B. K., and A. L. Ramanathan. 2009. “Organic Matter Characterization in a Tropical Estuarine-mangrove Ecosystem of India: Preliminary Assessment by Using Stable Isotopes and Lignin Phenols.” Estuarine, Coastal Shelf Science 84: 617–624. doi:10.1016/j.ecss.2009.07.029.
- Saintilan, N., K. Rogers, D. Mazumder, and C. Woodroffe. 2013. “Allochthonous and Autochthonous Contributions to Carbon Accumulations and Carbon Store in Southeastern Australian Coastal Wetlands.” Estuarine, Coastal and Shelf Science 128: 84–92. doi:10.1016/j.ecss.2013.05.010.
- Sanders, C. J., D. T. Maher, D. R. Tait, D. Williams, C. Holloway, J. Z. Sippo, and I. R. Santos. 2016. “Are Global Mangrove Carbon Stocks Driven by Rainfall?” Journal of Geophysical Research: Biogeosciences 121. doi:10.1002/2016JG003510.
- Sharma, S., J. Yasuoka, T. Nakamura, A. Watanabe, and K. Nadaoka 2014. “The Role of Hydroperiod, Soil Moisture and Distance from the River Mouth on Soil Organic Matter in Fukido Mangrove Forest.” Proceeding of the international conference on Advances in Applied Science and Engineering-ASEE 2014: 44–48.
- Siikamäki, J., J. N. Sanchirico, and S. L. Jardine. 2012. “Global Economic Potential for Reducing Carbon Dioxide Emissions from Mangrove Loss.” PNAS 109: 14369–14374. doi:10.1073/pnas.1200519109.
- Wang, G., D. Guan, M. R. Peart, Y. Chen, and Y. Peng. 2013. “Ecosystem Carbon Stocks of Mangrove Forest in Yingluo Bay, Guangdong Province of South China.” Forest Ecology and Management 310: 539–546. doi:10.1016/j.foreco.2013.08.045.
- Yong, Y., P. Baipeng, C. Guangcheng, and C. Yan. 2011. “Processes of Organic Carbon in Mangrove Ecosystems.” Acta Ecologica Sinica 31: 169–173. doi:10.1016/j.chnaes.2011.03.008.