ABSTRACT
Emission of methane (CH4), a major greenhouse gas, from submerged paddy soils is generally reduced by introducing intermittent drainage in summer, which is a common water management in Japan. However, such a practice is not widely conducted in Hokkaido, a northern region in Japan, to prevent a possible reduction in rice grain yield caused by cold weather. Therefore, the effects of intermittent drainage on CH4 emission and rice grain yield have not been investigated comprehensively in Hokkaido. In this study, we conducted a three-year field experiment in Hokkaido and measured CH4 and nitrous oxide (N2O) fluxes and rice grain yield to elucidate whether the reduction in CH4 emission can be achieved in Hokkaido as well as other regions in Japan. Four experimental treatments, namely, two soil types [soils of light clay (LiC) and heavy clay (HC) textures] and two water management [continuous flood irrigation (CF), and intermittent drainage (ID)], were used, and CH4 and N2O fluxes were measured throughout the rice cultivation periods from 2016 to 2018. Cumulative CH4 emissions in 2016 were markedly low, suggesting an initially low population of methanogens in the soils presumably due to no soil submergence or crop cultivation in the preceding years, which indicates a possible reduction in CH4 emission by introducing paddy-upland crop rotation. Cumulative CH4 emissions in the ID-LiC and ID-HC plots were 21–91% lower than those in the CF-LiC and CF-HC plots, respectively, whereas the cumulative N2O emissions did not significantly differ between the different water managements. The amount of CH4 emission reduction by the intermittent drainage was largest in 2018, with a comparatively long period of the first drainage for 12 days in summer. Rice grain yields did not significantly differ between the different water managements for the entire 3 years, although the percentage of well-formed rice grains was reduced by the intermittent drainage in 2018. These results suggest that CH4 emission from paddy fields can be reduced with no decrease in rice grain yield by the intermittent drainage in Hokkaido. In particular, the first drainage for a long period in summer is expected to reduce CH4 emission markedly.
1. Introduction
Methane (CH4) is a major greenhouse gas, which has 34 times higher global warming potential than carbon dioxide (CO2) in a time horizon of 100 years (Myhre et al. Citation2013).
Rice fields have been identified as a major source of atmospheric CH4 (Wassmann et al. Citation2000b; Myhre et al. Citation2013; Greenhouse Gas Inventory Office of Japan Citation2018) and have been therefore the subject of many previous studies (Yagi, Tsuruta, and Minami Citation1997; Wassmann et al. Citation2000a, Citation2000c; Minamikawa and Yagi Citation2009). The total CH4 emission from paddy soils in Japan in 2016 was estimated to be 556.3 Gg CH4 y−1 (Greenhouse Gas Inventory Office of Japan Citation2018).
In most parts of Japan, paddy soils are commonly drained occasionally in summer, which includes the first drainage, so-called ‘mid-season drainage’, carried out around early summer for generally 5–20 days, and intermittent drainage after the rice panicle formation stage with a sequence of drainage and reflooding within a few days until the end of summer (Greenhouse Gas Inventory Office of Japan Citation2018). The suitable water management practices, including the timing and interval of intermittent drainage, in each region, have been investigated in detail and recommended to farmers by local administrations. The main purposes of intermittent drainage are to prevent over-luxuriant growth, to reduce the number of nonproductive tillers, to prevent root rot caused by oxygen depletion in the soil, and to enhance the lodging resistance of plants by increasing soil hardness. At the same time, such a water management practice is also effective for reducing CH4 emission from the fields both in Japan (Yagi, Tsuruta, and Minami Citation1997; Minamikawa and Yagi Citation2009) and in many other countries in Asia (Wassmann et al. Citation2000a, Citation2000c; Tirol-Padre et al. Citation2018). Itoh et al. (Citation2011) have conducted an intensive collaborative research including nine field experiments in various regions in Japan. Their study revealed that CH4 emission can be reduced with no decrease in rice grain yield in most regions by introducing mid-season drainage longer than conventionally recommended. Although their collaborative study covered paddy fields in various regions in Japan, it included no experimental sites in Hokkaido.
Hokkaido is one of the major regions of rice cultivation, producing 581,800 Mg of rice grain (7.4% of the total production in Japan) in paddy fields of 103,900 ha in 2017 (Hokkaido Government Citation2019). Paddy soils in Hokkaido are conventionally flooded continuously, with no drainage practices from rice transplanting in spring to the end of summer, which is different from other regions in Japan. In the cold Hokkaido region, the growth rate of rice plants is low, and the rice plants potentially suffer from cold-weather-induced damage. Therefore, continuous flooding (CF) has been recommended regionally to keep the soil temperature higher and stable. However, recently, rice cultivars resistant to cold-weather-induced damage have been developed and become widely grown in Hokkaido, and the incidence of a severe decrease in rice grain yield caused by cold weather has been decreasing (Hokkaido Regional Agricultural Administration Office, Ministry of Agriculture, Forestry and Fisheries of Japan Citation2018; Hokkaido Government Citation2019). Therefore, by using such rice cultivars, it may be possible to reduce CH4 emission by introducing intermittent drainage without decreasing rice grain yield in Hokkaido as well as other regions in Japan. Although the effects of intermittent drainage (ID) on the reduction in CH4 emission from paddy soils have been intensively investigated as mentioned above, they have not been investigated intensively in Hokkaido. Although emission of CH4 has also been investigated in some previous studies in paddy fields in Hokkaido (Naser et al. Citation2007, Citation2018; Yoshida et al. Citation2014) and temporary decreases in CH4 flux by intermittent drainage were found (Naser et al. Citation2018; Yoshida et al. Citation2014), to the best of our knowledge, a study by Goto et al. (Citation2004) is the only previous publication in a peer-reviewed journal in which differences in cumulative CH4 emission were directly compared between the two water managements, i.e., those with CF and ID, in the same experimental field in Hokkaido.
Nitrous oxide (N2O) is another major greenhouse gas emitted from soils of croplands, which has 298 times higher global warming potential than CO2 in a time horizon of 100 years (Myhre et al. Citation2013). Among agricultural fields, although upland croplands are recognized to be the major source of N2O (Greenhouse Gas Inventory Office of Japan Citation2018), some previous studies also reported a marked increase in N2O emission from paddy soils during temporary drainage in field experiments in China (Cai et al. Citation1997, Citation1999) and the Philippines (Bronson et al. Citation1997). Therefore, in investigating CH4 emission from paddy soils, especially during temporary drainage, the possible increase in N2O emission as a trade-off of the reduction in CH4 emission should also be investigated simultaneously.
The main purpose of this study was to investigate whether CH4 emission can be reduced in the cold Hokkaido region by introducing intermittent drainage. We also aimed to investigate the effects of intermittent drainage on N2O emission and rice grain yield. We conducted a three-year field experiment on rice cultivation in Hokkaido and compared CH4 and N2O emissions and rice grain yield between the two types of water management, namely, continuous flood irrigation and intermittent drainage.
2. Materials and methods
2.1. Outline of experimental field and crop cultivation
The field experiment was conducted in experimental paddy fields (43°01ʹN, 141°25ʹE) in Hokkaido Agricultural Research Center, National Agriculture and Food Research Organization (HARC/NARO), a northern region in Japan. The averaged mean annual temperature from 1981 to 2010 was 7.1°C, annual precipitation was 938 mm, and the fields were covered with snow generally from November to March. On establishing this experimental field in 1989, the top layer of the original soil, classified as Allophanic Andosols, was removed, and two kinds of soils from other locations were filled to a thickness of approximately 70 cm. The top layer at depths of 0–10 cm of one soil type had a light clay texture (sand, 27.6%; silt, 30.7%; clay, 41.6%), hereafter referred to as LiC soil. The bulk density of the topsoil at depths of 0–10 cm was 0.99 Mg m−3, and the soil pH in H2O was 6.5. The C and N contents of the topsoil (at depths of 0–10 cm) were 16 mg C g soil−1 and 1.4 mg N g soil−1, respectively, and the noncrystalline iron content was 7.7 mg g soil−1. The top layer at depths of 0–15 cm of the other soil type had a heavy clay texture (sand, 5.0%; silt, 47.7%; clay, 47.3%), hereafter referred to as HC soil. The bulk density of the topsoil at depths of 0–10 cm was 0.91 Mg m−3, and the soil pH in H2O was 6.3. The C and N contents of the topsoil (0–10 cm depths) were 26 mg C g soil−1 and 2.1 mg N g soil−1, respectively, and the noncrystalline iron content was 10.9 mg g soil−1.
No flood irrigation or crop cultivation had been conducted in these fields from 2014 to 2015. In this study, rice was cultivated in the fields for three years from 2016 to 2018. The rice cultivar was Nanatsuboshi, one of the major cultivars planted in Hokkaido and is highly resistant to cold-weather damage. The rice seedlings were transplanted in May at intervals of 30 cm × 15 cm (22.2 hills m−2). A commercial compound fertilizer for rice including 14% N, 17% P2O5, and 12% K2O was incorporated into the soil by basal application in the first flood irrigation of the soil. The fertilization levels were 100 kg N ha−1 in 2016 and 85 kg N ha−1 in 2017 and 2018. No additional fertilizer was applied after the basal fertilization. The rice plants grew normally. Flowering was observed around the end of July, and mature rice grains were harvested in September. After the rice harvest in 2016 and 2017, part of the rice straw (500 g air-dried straw m−2) was broadcasted and incorporated into the soil. We investigated two water management practices, CF and ID, the details of which are described below, for each soil type. Therefore, in total, there were four experimental treatments (hereafter, referred to as CF-LiC, ID-LiC, CF-HC, and ID-HC) in this field experiment.
We used two experimental paddy fields for each soil type; each had an area of 138.5 m2 (16.2 m × 8.55 m) surrounded by a water shielding sidewall. The soil in one of the fields was continuously flooded, and that in the other was intermittently drained in summer. The assignment of treatments was changed yearly. Depending on the year, only one of the two fields was used for the experiment by manually dividing it into two parts (for CF and ID) with a vinyl chloride plate as the water shielding sidewall. The rotation of the experimental treatments in 3 years is illustrated in .
Figure 1. Arrangement of the experimental paddy fields in a three-year experiment from 2016 to 2018.
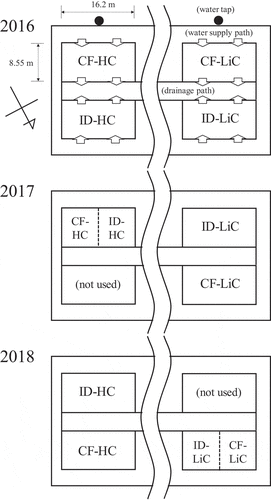
Details of the water management are as follows. In the CF plots, the soil was kept flooded from the first flood irrigation around the middle of May to the final drainage around the end of August. In the ID plots, the soil was kept flooded from the first flood irrigation to around late June. From late June to the beginning of July, the fields were drained (mid-season drainage; the first drainage in summer). The duration of the first drainage in summer was 4 days in 2016 and 2017, whereas it was 12 days in 2018. Thereafter, the fields were flooded again. From late July to the end of August, the ID plots were repeatedly drained and reflooded (intermittent drainage) with a sequence of generally four-day drainage and three-day flooding. The fields, as well as the CF plots, were finally drained around the end of August. When the soil was flooded, the water depth was generally maintained within 5–15 cm by occasional irrigation. The frequency of irrigation differed between the two soil types owing to the difference in their water requirement rate (apparent decreasing rate of the paddy water depth) (LiC soil, 26–29 mm day−1; HC soil, 6 mm day−1). A detailed history of the implemented agricultural management is summarized in .
Table 1. Specific dates of agricultural practices in the experimental fields from 2016 to 2018.
2.2. Measurement of CH4 and N2O fluxes
Methane and N2O fluxes were measured during the rice cultivation period with three replicates per treatment. In 2016, the fluxes were also measured after the rice harvest up to 14 October since a recognizable positive CH4 flux was still observed in some plots even after the rice harvest. The fluxes were measured generally once a week. When the ID plots were intermittently drained, the fluxes were measured more frequently, at least once in each period when the drained or flooded condition was kept in the ID plots.
A chamber consisting of transparent acrylic plates was gently placed on the piles previously driven into the hard subsoil layer. The bottom of the chamber was sealed with the paddy water. When the fields were drained, the sidewall of the chamber was directly inserted into the drained soil to a depth of about 1–3 cm. The chamber had a cross-sectional area of 0.18 m2 (0.6 m × 0.3 m). The height of the chamber was 0.5 m when the rice plants were small. It was increased to 1.0 m by inserting an additional sidewall when the rice plants grew taller. A small electric fan was attached to the top lid of the chamber to mix the air inside. Air was sampled for flux measurement between 9:00 and 12:00. The chamber, including four rice hills inside, was closed for 25 min. The air inside the chamber was drawn three times: 1, 13, and 25 min after closing the chamber using a syringe and immediately injected into an evacuated glass vial of 15 cm3.
A gas chromatograph (GC; GC-14B, Shimadzu, Japan) equipped with a flame ionization detector was used for CH4 measurement. Dinitrogen was used as the carrier gas. Another GC (GC-2014, Shimadzu, Japan) equipped with an electron capture detector and a switching valve for preliminarily separating water vapor was used for N2O measurement. Argon with 5% CH4 mixture gas was used as the carrier gas. Gas fluxes were calculated on the basis of the increasing/decreasing rates of gas concentration during the time when the chamber was closed. Cumulative gas emissions were calculated by integrating the measured instantaneous flux data by linear interpolation.
2.3. Other data measurements
The air temperature inside the chamber was measured using a thermistor thermometer (CT-410WR, Custom, Japan) to calculate the gas fluxes. Hourly data on ambient air temperature and precipitation were provided by the climate data acquisition station of HARC/NARO.
The soil reduction/oxidation potential (Eh) was measured using a portable Eh meter equipped with a reference electrode (PRN-41, Fujiwara Scientific Company, Japan) and a platinum-tipped electrode (Hirose-Rika, Japan). The platinum-tipped electrode was inserted into the soil at a depth of 10 cm (six electrodes per treatment) and left there throughout the rice cultivation period.
For the analysis of the bivalent iron (Fe2+) content, a soil core sample was collected occasionally at a depth of 0–7 cm with a cylindrical plastic tube and immediately sealed with a rubber stopper. Three soil core samples were collected in replicates from each treatment. An extract was obtained from 5 g of a soil sample with 50 cm3 of acetate buffer (pH 3.0) solution. The extract was mixed with the colorimetric agent o-phenanthroline, and the absorbance at 510 nm was analyzed using a spectrophotometer (UV-160A, Shimadzu, Japan).
At rice harvest, five adjacent rice hills were collected from five sites (total, 25 rice hills) in each plot to determine the rice grain yield. The collected five adjacent rice hills were bundled together and air-dried, threshed, and sieved. The weight of the brown rice grains with a width larger than 1.8 mm was converted into the values with the water content of 15% to calculate the brown rice grain yield. The percentage of well-formed rice grains, which is a common criterion in Japan for grading rice grains for food and is referred to as ‘seiryu-buai’, was measured using a rice analyzer (Shizuoka Seiki, ES 1000, Japan, or Satake, RGQI 20A, Japan).
2.4. Statistical analysis
The significance of differences in the cumulative CH4 and N2O emissions and rice grain yield between the CF and ID plots was determined by the two-way analysis of variance (ANOVA) and Tukey’s multiple comparison test, with the water management practice (CF and ID) and the year (2016–2018) as the two factors, and the soil types (i.e., the LiC and HC soils) were the two replicates (n = 2).
3. Results
3.1. Climate
Seasonal changes in air temperature, precipitation, CH4 and N2O fluxes, soil Eh, and soil Fe2+ content are shown in –. The average air temperature from July to August was lower in 2018 (19.6ºC) than in 2016 (20.4ºC) and 2017 (20.2ºC). The cumulative precipitation from July to August was lower in 2017 (165 mm) than in 2016 (369 mm) and 2018 (415 mm). Cumulative sunshine hours from July to August were shorter in 2018 (292 h) than in 2016 (400 h) and 2017 (355 h) (data not shown).
Figure 2. Seasonal change in air temperature, precipitation, CH4 and N2O fluxes, soil reduction/oxidation potential (Eh) at depth of 10 cm, and soil bivalent iron (Fe2+) content in experimental paddy fields during the rice cultivation period in 2016.
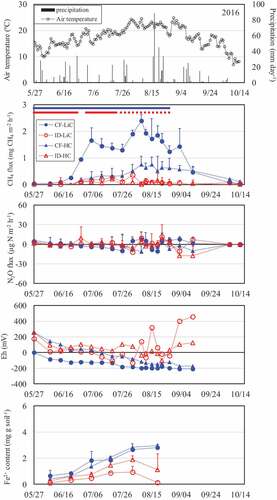
Figure 3. Seasonal change in air temperature, precipitation, CH4 and N2O fluxes, soil reduction/oxidation potential (Eh) at depth of 10 cm, and soil bivalent iron (Fe2+) content in experimental paddy fields during the rice cultivation period in 2017.
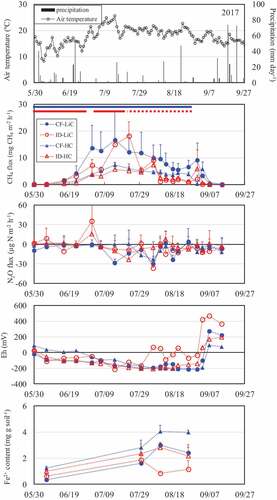
Figure 4. Seasonal change in air temperature, precipitation, CH4 and N2O fluxes, soil reduction/oxidation potential (Eh) at depth of 10 cm, and soil bivalent iron (Fe2+) content in experimental paddy fields during the rice cultivation period in 2018.
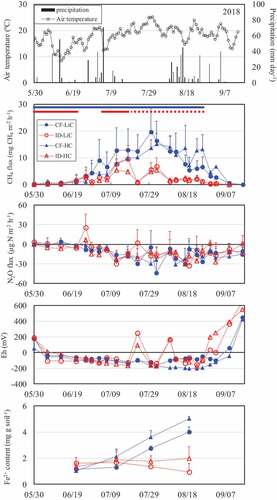
In 2018, there were many rainy days with low sunshine from late June to the beginning of July and from mid to late August in this field. The general trend of the weather was similar for the entire Hokkaido in 2018, with low temperature and sunshine hours from mid-June to mid-July and from mid to late August, which caused severely low regional rice grain yields in the entire Hokkaido region, corresponding to 90% of ordinary years (Hokkaido Regional Agricultural Administration Office, Ministry of Agriculture, Forestry and Fisheries of Japan Citation2018).
3.2. CH4 and N2O fluxes
From –, CH4 flux was low initially and gradually increased during the period with continuous flood irrigation, i.e., from the first irrigation in spring to the first drainage in summer. After the first drainage in summer, the CH4 fluxes in the ID plots became lower than those in the CF plots in 2016 and 2018 for both soil types. In 2017, the differences in CH4 flux between the CF and ID plots were comparatively small; some of the CH4 flux data in the ID plots were higher than those in the CF plots (e.g., 23 July for the LiC soil, 6 August for the HC soil) during the period with intermittent drainage in summer.
After the final drainage around the end of August, the CH4 flux decreased rapidly. In 2016, a recognizable positive CH4 flux was still observed on 12 September in the CF plots; therefore, we continued the CH4 flux measurement after the rice harvest (7 October and 14 October).
As for the comparison among the years, the CH4 flux in 2016 was markedly lower than those in 2017 and 2018 (–). As for the comparison between the soil types, the CH4 fluxes in the plots with the LiC soil were apparently higher than those in the plots with the HC soil in 2016 and 2017, whereas the fluxes were similar for both soil types in 2018.
Soil Eh gradually decreased in all the plots during the period with continuous flood irrigation. In 2016, the progress of decrease in Eh was delayed compared with that in other years, except in the CF-LiC plot. Thereafter, in the CF plots, the soil Eh generally remained low at around −200 mV until the final drainage in all the 3 years, although those in the CF-HC plot in 2016 and in the CF-LiC plot in 2018 were somewhat higher, from −150 to −100 mV. In the ID plots, the soil Eh occasionally increased temporarily to positive values in accordance with intermittent drainage in summer. However, in the ID-HC plot in 2017, no recognizable temporary increases were observed throughout the summer. After the final drainage around the end of August, the soil Eh in all the plots increased to positive values except for the CF plots in 2016. In the CF plots in 2016, the paddy water was poorly drained after the final drainage, which presumably prevented the increase in soil Eh.
The soil Fe2+ content was initially low and gradually increased until the first drainage in summer. Thereafter, the Fe2+ contents in the CF plots became significantly higher than those in the ID plots after late July in all the 3 years and for both soil types.
During the entire experimental periods, the N2O fluxes were small and occasionally showed negative values, indicating slight N2O absorption in the paddy water. In 2017 and 2018, slight temporary increases in N2O flux were observed in the ID plots during the first drainage in summer ( and ; on 2 July 2017 and 26 June 2018), the maximum of which was 36 µg N m−2 h−1. On the other hand, no such temporary increase in N2O flux was observed thereafter (during the period with intermittent drainage in summer) in all the 3 years. After the final drainage, the N2O flux also remained low. In 2016, it also remained low after the rice harvest.
3.3. Cumulative CH4 and N2O emissions
Cumulative CH4 and N2O emissions are summarized in . The cumulative CH4 emission showed large variations ranging from 1 to 186 kg CH4 ha−1. As for the comparison among the years, the CH4 emission in 2016 was significantly lower than those in 2017 and 2018. The cumulative CH4 emission was lower in the ID plots than in the CF plots in all the 3 years and for both soil types. The decrease in CH4 emission by the intermittent drainage was shown to be statistically significant by two-way ANOVA. The extents of the decrease in CH4 emission by the intermittent drainage ranged from 21% to 91%. The amount of CH4 emission reduction by the intermittent drainage was largest in 2018 for both soil types, which were 116 and 119 kg CH4 ha−1 for the LiC and HC soils, respectively.
Table 2. Cumulative CH4 and N2O emissions in the experimental paddy fields from 2016 to 2018.
The cumulative N2O emissions ranged from −0.33 to +0.01 kg N ha−1. Although the emission tended to be higher (i.e., less negative) in the ID plots, there were no statically significant differences between the CF and ID plots. As for the comparison among the years, the N2O emission in 2018 was significantly lower than those in 2016 and 2017, although the differences were small.
3.4. Rice grain yield
Rice grain yields are summarized in . The brown rice grain yields ranged from 640 to 678 in 2016, from 446 to 673 in 2017, and from 486 to 612 g m−2 in 2018. The difference in rice grain yield between the CF and ID treatments was not statistically significant. Although the difference among the years was not statistically significant, either, the rice grain yields in 2016 were somewhat higher than those in 2017 and 2018, presumably mainly owing to the higher fertilization level in 2016.
Table 3. Brown rice grain yields in the experimental paddy fields from 2016 to 2018.
In 2016 and 2017, the percentages of well-formed rice grains (seiryu-buai) were higher than 70% both in the CF and ID plots; such rice grains were classified as first-grade rice grains for food on the basis of the criterion of the Ministry of Agriculture, Forestry and Fisheries of Japan. In 2018, the levels of the percentages of well-formed rice grains were comparatively low, 69% and 68%, (classified as second grade) in the ID-LiC and CF-HC plots, respectively, and even much lower, 58% (classified as third grade), in the ID-HC plot.
4. Discussion
Reduction in CH4 emission from paddy soils by the intermittent drainage has been observed in many previous studies (Yagi, Tsuruta, and Minami Citation1997; Wassmann et al. Citation2000a, Citation2000c; Minamikawa and Yagi Citation2009; Tirol-Padre et al. Citation2018); however, it has not been investigated well in Hokkaido, a region with a cold climate. The decrease in CH4 emission by the drainage is generally accompanied by a temporary increase in soil Eh and a decrease in soil Fe2+ content, which indicates the suppression of soil reduction by the drainage. In this study, we also found decreases in CH4 flux and temporary increases in soil Eh and decreases in soil Fe2+ content (–) induced by the intermittent drainage, which agree well with general findings in many previous studies.
The extent of CH4 emission reduction by the intermittent drainage is, however, highly variable. Goto et al. (Citation2004) investigated the effects of various intermittent drainage practices in a four-year field experiment in Hokkaido, and found that the cumulative CH4 emission was reduced by 5 – 50% by the intermittent drainage compared with the continuous flood irrigation. In this study, the extent of CH4 emission reduction was also highly variable; the cumulative CH4 emission was greatly reduced (reduction by 66 – 91%) by the introduction of intermittent drainage in 2016 and 2018 but not greatly reduced in 2017 (reduction by 21 – 35%).
As for the comparison among the years, the cumulative CH4 emission in 2016 was markedly lower than those in 2017 and 2018 for both soil types. Although the differences in soil Fe2+ content were not clear among the 3 years, comparatively high soil Eh levels throughout the rice cultivation period in 2016 than in 2017 and 2018 (–), except in the CF-LiC plot, indicates the significant suppression of soil reduction in 2016. The low CH4 emission in 2016 may have been closely related to the cultivation history of the fields. In these fields, no flood irrigation or crop cultivation had been conducted from 2014 to 2015. Some previous studies showed that the preceding upland crop cultivation (with no soil flooding) significantly reduced CH4 emission from the flooded paddy soil in the succeeding year (Kumagai and Konno Citation1998; Cai, Tsuruta, and Minami Citation2000; Eusufzai et al. Citation2010; Nishimura et al. Citation2011; Takakai et al. Citation2017; Naser et al. Citation2018). Although we did not prepare for experimental treatments with consecutive paddy rice cultivations as a control in this study, the soils were thought to initially have low CH4 production potentials both in the CF and ID plots in the spring of 2016 (Kumagai and Konno Citation1998; Eusufzai et al. Citation2010), and the conditions may have been effectively maintained by the intermittent drainage in the ID plots. In addition, the absence of crop cultivation in the preceding years may have resulted in the low contents of labile carbon remaining in the soil at the beginning of 2016, which further decreased the initial CH4 production potential in the soils (Yagi and Minami Citation1990; Yan et al. Citation2005). After 2017, the CH4 fluxes increased presumably owing to the CH4 production potential increased by the paddy rice cultivation in the preceding years. In some of the above-mentioned previous studies, the significant reduction in CH4 emission was also observed only in the first year of rice cultivation after the preceding upland crop cultivations (Kumagai and Konno Citation1998; Nishimura et al. Citation2011).
In 2017, differences in CH4 flux between the CF and ID plots were small. During the period with temporary drainage in summer, the CH4 flux in the ID plots occasionally increased during the drainage, reaching values higher than those in the CF plots (e.g., 23 July for the LiC soil, and 6 August for the HC soil). The temporary increases in CH4 flux in the ID plots during the drainage may have been related to the temporary release of CH4 previously accumulated in the gas phase in the soil. Some previous studies also showed the occasional temporary increase in CH4 flux during intermittent drainage (e.g., Wassmann et al. Citation1994; Yagi et al. Citation1996) and suggested its relationship with the CH4 previously accumulated in the soil. On the other hand, in 2018, no such temporary increases in CH4 flux were observed in the ID plots, and the fluxes in the ID plots were consistently lower than those in the CF plots during the summer, which indicates that the amount of CH4 accumulated in the soil of the ID plots remained low level owing to the sufficient oxidation of the soil during the intermittent drainage.
Itoh et al. (Citation2011) analyzed CH4 flux data from various experimental sites and found that cumulative CH4 emission tended to decrease with an increasing number of days without rain during the first summer drainage. Therefore, in this study, the comparatively long first drainage in summer for 12 days in 2018 () may have been effective in reducing the CH4 flux thereafter. In contrast, the first summer drainage for 4 days in 2017 may not have been sufficiently long to supply oxygen into the soil and to effectively reduce the CH4 flux thereafter.
The cumulative CH4 emission was lower in the ID plots than in the CF plots in all the 3 years and for both soil types (), and the difference between the CF and ID plots was shown to be significant at 5% by two-way ANOVA. The results of this study indicate that intermittent drainage in summer is also effective for reducing CH4 emission in Hokkaido as well as other regions in Japan.
The N2O flux was generally low in this study, and the flux sometimes reached small negative values in the latter rice growth periods. Although the reasons for the negative N2O flux data remain unclarified, some other previous studies also showed occasionally such negative N2O fluxes from paddy soils (Toma et al. Citation2015).
Nishimura et al. (Citation2004, Citation2011) found a temporarily high N2O efflux immediately after the first flood irrigation in spring, suggesting N2O production in the soil in relation to the rapid depletion of oxygen in the soil. In this study, however, the first flux measurement was conducted about 2 weeks after the flood irrigation (, –). Therefore, in this study, we may have missed the temporary increase in N2O flux immediately after the first flood irrigation.
In 2017 and 2018, we found temporary increases in N2O flux during the first summer drainage ( and ; on 2 July 2017, and 26 June 2018). On the other hand, we found no such increases in N2O flux thereafter during the succeeding period with intermittent drainage. Some previous studies also showed a small temporary N2O efflux during periods with intermittent drainage. Nishimura et al. (Citation2004) observed a small N2O efflux during periods with intermittent drainage only immediately after supplemental nitrogen (N) fertilization, which indicates that the newly supplied inorganic N at the soil surface became the source of N2O. Although the detailed mechanisms of N2O production during a temporary drainage still remain unclarified, part of the supplied ammonium-N that remained until the first drainage in summer may have been the source of N2O, and only a small amount of ammonium-N remained thereafter for N2O production during the succeeding period with intermittent drainage.
In transplanted rice cultivation in Hokkaido, all the required amount of N fertilizer is commonly applied by basal incorporation, and no additional N fertilizer is applied in summer. Therefore, little N2O efflux may occur during the period with intermittent drainage. This also indicates that the effect of intermittent drainage on cumulative N2O emission is small when no additional fertilization is carried out in summer. In this study, although a temporary small N2O efflux was found during the first drainage in summer in the ID plots, its contribution to the cumulative N2O emissions was small and it did not significantly differ statistically between the CF and ID plots ().
In this study, the effect of the water management practice on rice grain yield was not clear, with no statistically significant difference in the yield between the CF and IC plots (). In the four-year experiment by Goto et al. (Citation2004), significant decreases in rice grain yield caused by the difference in water management were not observed, either (Kamikawa Agricultural Experiment Station Citation2000). On the basis of the results of the previous and present studies, we concluded that when the weather conditions are normal during the rice growth period, the rice grain yield is not decreased by the intermittent drainage of paddy water in summer.
However, in 2018, the percentages of well-formed rice grains became lower than 70% (the threshold for grading rice for food as first grade) in some plots, and that in the ID plots became even lower than that in the CF plots (). As mentioned above, the regional rice grain yield in the entire Hokkaido region in 2018 was very low, which corresponded to 90% of ordinary years, mainly owing to the low temperature and low sunshine hours from mid-June to mid-July and from mid to late August (Hokkaido Regional Agricultural Administration Office, Ministry of Agriculture, Forestry and Fisheries of Japan Citation2018). This result suggests that drainage of paddy water in years with severe weather conditions may have some negative effects on the quality of rice grains. In addition, the comparatively long period of the first drainage in summer in 2018 (12 days) in this study may not have been suitable under such weather conditions. Furthermore, if unusually low temperatures continue around the period of panicle formation of rice plants, drainage of paddy soil under such condition should be avoided to prevent severe damage to the rice panicle formation. Since the effects of intermittent drainage have not been investigated well in Hokkaido, further field experiments for longer years in paddy fields in various locations in Hokkaido should be carried out in the future studies. Also, in future studies, it may be useful to develop some supporting systems with which a suitable paddy water management can be recommended to farmers on the basis of the regional weather forecast of upcoming days.
5. Conclusions
The results of this study indicate that CH4 emission from paddy fields can be effectively decreased by water management with intermittent drainage in the cold Hokkaido region as well as other regions in Japan. In particular, the first drainage for a long period in summer (longer than 10 days) was expected to be highly effective for reducing CH4 emission. No increase in N2O emission, as a possible trade-off of the reduction in CH4 emission, was observed in this study. Moreover, no decrease in rice grain yield by the intermittent drainage was observed in this study, although the quality of rice grains was decreased by the intermittent drainage in a year with severe weather conditions in summer.
In this study, we also found a markedly low CH4 emission in the first year of the field experiment (2016) because no flood irrigation was carried out in the preceding years. In this region, paddy-upland crop rotation is recommended by the local administration to maintain high crop productivity. The results of this study also suggest that the paddy-upland crop rotation can be effective for reducing greenhouse gas emissions owing to the reduction in CH4 emission from paddy soils with a history of upland crop cultivation.
Acknowledgments
The authors would like to thank Ken Nakamura of the Institute for Agro-Environmental Sciences, NARO, for the analysis of iron in the soils. The authors also thank Yumi Ito of HARC/NARO for gas analysis with GC, and Ryo Ohtomo, Kazunari Nagaoka, Sho Morimoto, Motohiro Yoshimura, and the staff of the experimental field management section of HARC/NARO for support in the experiments and field management. This work was supported by the ordinary research fund by NARO. Any information on the experimental data shown in this article is available on request to S. Nishimura.
Disclosure statement
No potential conflict of interest was reported by the authors.
Additional information
Funding
References
- Bronson, K. F., H. U. Neue, U. Singh, and E. B. Abao Jr. 1997. “Automated Chamber Measurements of Methane and Nitrous Oxide Flux in a Flooded Rice Soil: I. Residue, Nitrogen, and Water Management.” Soil Science Society of America Journal 61: 981–987. doi:10.2136/sssaj1997.03615995006100030038x.
- Cai, Z., G. Xing, G. Shen, H. Xu, X. Yan, H. Tsuruta, K. Yagi, and K. Minami. 1999. “Measurements of CH4 and N2O Emissions from Rice Paddies in Fengqiu, China.” Soil Science and Plant Nutrition 45: 1–13. doi:10.1080/00380768.1999.10409320.
- Cai, Z., X. Xing, X. Yan, H. Xu, H. Tsuruta, K. Yagi, and K. Minami. 1997. “Methane and Nitrous Oxide Emissions from Rice Paddy Fields as Affected by Nitrogen Fertilisers and Water Management.” Plant and Soil 196: 7–14. doi:10.1023/A:1004263405020.
- Cai, Z. C., H. Tsuruta, and K. Minami. 2000. “Methane Emission from Rice Fields in China: Measurements and Influencing Factors.” Journal of Geophysical Research 105D: 17231–17242. doi:10.1029/2000JD900014.
- Eusufzai, M. K., T. Tokida, M. Okada, S. Sugiyama, G. C. Liu, M. Nakajima, and R. Sameshima. 2010. “Methane Emission from Rice Fields as Affected by Land Use Change.” Agriculture, Ecosystems & Environment 139: 742–748. doi:10.1016/j.agee.2010.11.003.
- Goto, E., Y. Miyamori, S. Hasegawa, and O. Inatsu. 2004. “Reduction Effects of Accelerating Rice Straw Decomposition and Water Management on Methane Emission from Paddy Fields in a Cold District.” Japanese Journal of Soil Science and Plant Nutrition 75: 191–201. (in Japanese with English summary).
- Greenhouse Gas Inventory Office of Japan. 2018. National Greenhouse Gas Inventory Report of JAPAN 2018. Japan: Greenhouse Gas Inventory Office of Japan, Center for Global Environmental Research, National Institute for Environmental Studies. http://www.cger.nies.go.jp/en/activities/supporting/publications/report/index.html
- Hokkaido Government. 2019. Suiden menseki, suitou sakutsuke menseki, oyobi shuukaku-ryou [Area of Rice Paddy and Rice Grain Yield in Hokkaido]. (in Japanese). http://www.pref.hokkaido.lg.jp/ns/nsk/kome/05_r1.pdf
- Hokkaido Regional Agricultural Administration Office, Ministry of Agriculture, Forestry and Fisheries of Japan. 2018. Nourin-suisan toukei Hokkaido [Statistics of Agriculture, Forestry and Fisheries in Hokkaido]. (in Japanese). http://www.maff.go.jp/hokkaido/toukei/kikaku/sokuho/attach/pdf/index-6.pdf
- Itoh, M., S. Sudo, S. Mori, H. Saito, T. Yoshida, Y. Shiratori, S. Suga, et al. 2011. “Mitigation of Methane Emissions from Paddy Fields by Prolonging Midseason Drainage.” Agriculture, Ecosystems & Environment 141 :359–372. doi:10.1016/j.agee.2011.03.019.
- Kamikawa Agricultural Experiment Station. 2000. Suiden ni okeru metan hassei yokusei no tameno hojou kanri gijutsu [Agricultural Management for Reducing Methane Emission in Paddy Fields]. Hokkaido Nougyou Shiken Kaigi Shiryou. (in Japanese). Pippu, Hokkaido, Japan.
- Kumagai, K., and Y. Konno. 1998. “Methane Emission from Rice Paddy Fields after Upland Farming.” Japanese Journal of Soil Science and Plant Nutrition 69: 333–339. (in Japanese with English summary).
- Minamikawa, K., and K. Yagi. 2009. “Possibility of Water Management for Mitigating Total Emission of Greenhouse Gases from Irrigated Paddy Field.” In Climate Change and Crops, edited by S. N. Singh, 307–328. Berlin: Springer.
- Myhre, G., D. Shindell, F. M. Bréon, W. Collins, J. Fuglestvedt, J. Huang, D. Koch, et al. 2013. “Anthropogenic and Natural Radiative Forcing.” In Climate Change 2013: The Physical Science Basis. Contribution of Working Group I to the Fifth Assessment Report of the Intergovernmental Panel on Climate Change, edited by T. F. Stocker, D. Qin, G. K. Plattner, M. Tignor, S. K. Allen, J. Boschung, A. Nauels, et al., 659–740. Cambridge: Cambridge University Press.
- Naser, H. M., O. Nagata, S. Sultana, and R. Hatano. 2018. “Impact of Management Practices on Methane Emissions from Paddy Grown on Mineral Soil over Peat in Central Hokkaido, Japan.” Atmosphere 9: 212. doi:10.3390/atmos9060212.
- Naser, H. M., O. Nagata, S. Tamura, and R. Hatano. 2007. “Methane Emissions from Five Paddy Fields with Different Amounts of Rice Straw Application in Central Hokkaido, Japan.” Soil Science and Plant Nutrition 53: 95–101. doi:10.1111/j.1747-0765.2007.00105.x.
- Nishimura, S., H. Akiyama, S. Sudo, T. Fumoto, W. Cheng, and K. Yagi. 2011. “Combined Emission of CH4 and N2O from a Paddy Field Was Reduced by Preceding Upland Crop Cultivation.” Soil Science and Plant Nutrition 57: 167–178. doi:10.1080/00380768.2010.551346.
- Nishimura, S., T. Sawamoto, H. Akiyama, S. Sudo, and K. Yagi. 2004. “Methane and Nitrous Oxide Emissions from a Paddy Field with Japanese Conventional Water Management and Fertilizer Application.” Global Biogeochemical Cycles 18 (GB2017). doi:10.1029/2003GB002207.
- Takakai, F., S. Nakagawa, K. Sato, K. Kon, T. Sato, and Y. Kaneta. 2017. “Net Greenhouse Gas Budget and Soil Carbon Storage in a Field with Paddy-upland Rotation with Different History of Manure Application.” Agriculture 7: 49. doi:10.3390/agriculture7060049.
- Tirol-Padre, A., K. Minamikawa, T. Tokida, R. Wassmann, and K. Yagi. 2018. “Site-specific Feasibility of Alternate Wetting and Drying as a Greenhouse Gas Mitigation Option in Irrigated Rice Fields in Southeast Asia: A Synthesis.” Soil Science and Plant Nutrition 64: 2–13. doi:10.1080/00380768.2017.1409602.
- Toma, Y., S. Oomori, A. Maruyama, H. Ueno, and O. Nagata. 2015. “Effect of the Number of Tillages in Fallow Season and Fertilizer Type on Greenhouse Gas Emission from a Rice (Oryza Sativa L.) Paddy Field in Ehime, Southwestern Japan.” Soil Science and Plant Nutrition 62: 69–79. doi:10.1080/00380768.2015.1109999.
- Wassmann, R., R. S. Lantin, H. U. Neue, L. V. Buendia, T. M. Corton, and Y. Lu. 2000a. “Characterization of Methane Emissions from Rice Fields in Asia. III. Mitigation Options and Future Research Needs.” Nutrient Cycling in Agroecosystems 58: 23–36. doi:10.1023/A:1009874014903.
- Wassmann, R., H. U. Neue, R. S. Lantin, J. B. Aduma, M. C. R. Alberto, M. J. Andales, M. J. Tan, et al. 1994. “Temporal Patterns of Methane Emissions from Wetland Rice Fields Treated by Different Modes of N Application.” Journal of Geophysical Research 99 :16457–16462. doi:10.1029/94JD00017.
- Wassmann, R., H. U. Neue, R. S. Lantin, L. V. Buendia, and H. Rennenberg. 2000b. “Characterization of Methane Emissions from Rice Fields in Asia. I. Comparison among Field Sites in Five Countries.” Nutrient Cycling in Agroecosystems 58: 1–12. doi:10.1023/A:1009848813994.
- Wassmann, R., H. U. Neue, R. S. Lantin, K. Makarim, N. Chareonsilp, L. V. Buendia, and H. Rennenberg. 2000c. “Characterization of Methane Emissions from Rice Fields in Asia. II. Differences among Irrigated, Rainfed, and Deepwater Rice.” Nutrient Cycling in Agroecosystems 58: 13–22. doi:10.1023/A:1009822030832.
- Yagi, K., and K. Minami. 1990. “Effect of Organic Matter Application on Methane Emission from Some Japanese Paddy Fields.” Soil Science and Plant Nutrition 36: 599–610. doi:10.1080/00380768.1990.10416797.
- Yagi, K., H. Tsuruta, K. Kanda, and K. Minami. 1996. “Effect of Water Management on Methane Emission from a Japanese Rice Paddy Field: Automated Methane Monitoring.” Global Biogeochemical Cycles 10: 255–267. doi:10.1029/96GB00517.
- Yagi, K., H. Tsuruta, and K. Minami. 1997. “Possible Options for Mitigating Methane Emission from Rice Cultivation.” Nutrient Cycling in Agroecosystems 49: 213–220. doi:10.1023/A:1009743909716.
- Yan, X., K. Yagi, H. Akiyama, and H. Akimoto. 2005. “Statistical Analysis of the Major Variables Controlling Methane Emission from Rice Fields.” Global Change Biology 11: 1131–1141. doi:10.1111/j.1365-2486.2005.00976.x.
- Yoshida, O., K. Yoshida, N. Nakatani, and K. Ushiyama. 2014. “Greenhouse Gases from Early Flooded and Organic Paddy Rice Field around the Lake Miyajimanuma.” Wetland Research 5: 25–33. (in Japanese with English title).