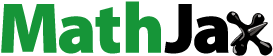
ABSTRACT
Cotton is critical for phosphorus demands and very sensitive for its deficiency. However, identifying the effect of low-phosphorus tolerance on cotton growth, yield, and fiber quality by reducing phosphorus consumption. This may help to develop phosphorus-tolerant high-yielding cotton cultivars. In a two-year repeated (2015 and 2016) hydroponic experiment (using 0.01 and 1 mM KH2PO4), two cotton cultivars with phosphorus sensitivity (Lu 54; a low-phosphorus sensitive and Yuzaomian 9110; a low-phosphorus tolerant) were screened on the base of agronomic traits and physiological indices through correlation analysis, cluster analysis and principal component analysis from 16 cotton cultivars. Low phosphorus nutrition reduced the plant height, leaf number, leaf area, phosphorus accumulation and biomass in various organs of seedlings. The deficiency negatively affected the morphogenesis of seedlings, as well as yield and fiber quality. Further, these screened cultivars were tested in a pot experiment with 0, 50, 100, 150, 200 kg P2O5 ha−1 during 2016 and 2017. It was found to have a significant (P< 0.05) difference in boll number, lint yield, fiber strength, and micronaire at the harvest. Furthermore, after collectively analyzed the characteristics of Lu 54 and Yuzaomian 9110, there were six key indices that could improve the low phosphorus tolerance of cotton cultivars. These were root phosphorus accumulation, stem phosphorus accumulation percentage, leaf and total biomass of seedlings, seed cotton weight per boll and fiber length.
1. Introduction
Phosphorus (P) is one of the least available and least mobile nutrients to the plants in many cropping eco-systems (Goldstein, Baertiein, and Mcdaniel Citation1988). This element plays an important role in plant growth and development, hence considered as critical for sustainable production of fiber worldwide (Pavinato, Dao, and Rosolem Citation2010; Rose et al. Citation2016). Cotton is one of the most critical fiber-producing crops and very sensitive to P (Asher and Loneragan Citation1967; Föhse, Claassen, and Jungk Citation1988). However, cotton cultivars differ for P sensitivity, uptake, and acquisition. Furthermore, P deficiency negatively affects the crop growth, yield, and quality. Previous studies reported that P sensitivity resulted in notable differences in many indices among different genotypes (Fageria et al. Citation2010; Araújo et al. Citation2012 ; Zambrosi et al. Citation2012; Fageria, Knupp, and Moraes Citation2013; Santos et al. Citation2015). Thus, selecting cultivars with low-P tolerance can be a good strategy to enhance productivity.
Cultivar selection for a sustainable production system is considered a basic decision. However, a lot of literature are available on the cultivar evaluation with respect to P sensitivity in various crops (Yan, Lynch, and Beebe Citation1996; Rodríguez et al. Citation1998; Gahoonia and Nielsen Citation2004; Hernández et al. Citation2007; Ramaekers et al. Citation2010), but a very few studies provide information on P sensitivity in cotton cultivars. Previous studies also differed on the base of screening indices in cotton cultivars (Wang et al. Citation2009; Li et al. Citation2010). Furthermore, these studies concluded that biomass and P content are important indices of cultivar evaluation especially, at the seedling stage. Unluckily, the previous studies have not directed that what are the indices indicated efficient evaluation in seedling (Bovill et al. Citation2013; Rose et al. Citation2016), and what are the factors that may affect the final yield and fiber quality at the harvest in cotton. Therefore, in order to select cotton genotype with different P sensitivities, evaluation on the base of difference in agronomic traits at the seedling stage, yield, and fiber quality is important to enhance the low-P tolerance ability of cotton.
Against this backdrop, the objectives of this study were to (1) screen two cotton cultivars (Lu 54 and Yuzaomian 9110) with P sensitivity difference at the seedling stage, (2) analyze the agronomic traits of two cultivars under P deficient conditions in seedling, and (3) assess the difference of yield components and the main fiber properties between the two cultivars at the harvest.
2. Materials and methods
2.1. Nutrient solution culture (a screening test)
The screening test for P sensitivity was conducted in nutrient solution culture using 16 cotton cultivars (Z1: Lu 54, Z2: CCRI 50, Z3: Hannongmianzao 1, Z4: Yu 068, Z5: Lu 7619, Z6: Yuzaomian 9110, Z7: Deltapine, Z8: De 0720, Y1: CCRI 88, Y2: CCRI 69, Y3: CCRI 79, Y4: Huamian 3109, Y5: Lu 6269, Y6: Lumianyan 36, Y7: K836-1, Y8: K836-2) during 2015 and 2016 in a growth cabinet RDN-1000D-4 (Ningbo Southeast Instrument Co., Ltd., Ningbo, China) at Nanjing Agricultural University, situated at Jiangsu Province, China (118°50´E, 32°02´N). Planted dates were December 12, 2015 and February 26, 2016 under two P concentrations (LP: 0.01 mM and HP: 1 mM KH2PO4) adapted from Chen et al. (Citation2014). A diurnal temperature ranged from (22 ± 1) °C to (32 ± 1) °C, relative humidity was 70% ± 2%, and light intensity set at 19,000 lx. The lights were only opened 12 h a day. Each treatment had six pots and each pot had two cotton seedlings. The 50 ml 25% modified Hoagland nutrient solution was supplied in each pot with 3-day interval. After 7 days, the 25% solution replaced by 100% solution. The 100% modified Hoagland nutrient solution (pH adjusted to 6.5 with NaOH, Hoagland and Arnon Citation1950) was composed of 0.01 mM (adding 0.99 mM KCl) or 1 mM KH2PO4, 5 mM KNO3, 5 mM Ca(NO3)2 · 4H2O, 2 mM MgSO4 · 7H2O, 46.24 μM H3BO3, 9.15 μM MnCl2 · 4H2O, 0.77 μM ZnSO4 · 7H2O, 0.32 μM CuSO4 · 5H2O, 0.11 μM H2MoO4·H2O and 20 mΜ FeNaEDTA.
When LP treatments were at the five-leaf cotton stage, all the samples would be collected (January 28, 2016 and April 6, 2016, respectively). Chlorophyll content (SPAD value of functional leaf) was recorded by using a SPAD meter (Konica Minolta, inc., Japan). After getting the SPAD values, samples were divided into two parts. Half of the samples were stored at −80°C for testing the sucrose content and malondialdehyde (MDA) content of functional leaf (the fourth fully expanded true leaf). The rest half of the samples were oven-dried first at 105°C for 30 min then heated at 80°C for 72 h for measuring the biomass and P content (Mo-Sb colorimetric method, Lu Citation1999) after evaluating the plant height, root length, and leaf area. The sucrose was extracted by a modified method of Pettigrew (Citation2001), and its assay was conducted according to the method of Hendrix (Citation1993). The MDA content was measured by the method of Zhao et al. (Citation2013).
2.2. Soil-based pot culture (a fertilization test)
A pot experiment was conducted in the experimental field at the Pailou Experimental Station, Nanjing Agriculture University, Jiangsu Province, China (118°50´E, 32°02´N), in 2016 and 2017. Two cotton cultivars (Lu 54 and Yuzaomian 9110) screened from a hydroponic experiment on the base of agronomic traits, were used. The experimental soil was a clay, mixed, thermic, typic alfisols (udalfs; FAO-UNESCO system; luvisol), with pH 6.7, organic matter for the 17.0 and 17.8 g kg−1, 1.17 and 1.14 g kg−1 total nitrogen, 82.7 and 86.0 mg kg−1 alkali solution nitrogen, 17.0 and 19.1 mg kg−1 Olsen-P, as well as 113.8 and 111.3 mg kg−1 rapidly available potassium at a depth of 0–20 cm prior to transplanting in 2016 and 2017, respectively. Cotton seedlings were sown on May 18th, 2016, and May 1st, 2017, and transplanted at three true leaf stages into the pots (three seedlings per pot). The pot with 23 cm diameter and 49 cm depth, had a capacity of 60 kg soil. The treatments were inclusive of P fertilizer rates: 0, 50, 100, 150, 200 kg P2O5 ha−1, which just in order to create different soil P supply levels for cotton. Each treatment set 36 pots as a repeat. The fertilizer P rate in each pot was calculated by its soil weight. The field soil was calculated by 2.25 × 106 kg ha−1. Nitrogen was applied at 225 kg N ha−1 (40% and 60% at the transplanting stage and flowering stage, respectively), and potassium application at the rate of 225 kg K2O ha−1 with 100% basal dose. Nitrogen, phosphorus, and potassium fertilizers were applied as urea (46% of N), triple superphosphate (46% of P2O5) and potassium chloride (60% of K2O), respectively.
Determinations of yield and biomass were conducted on October 11, 2016 and September 10th, 2017. One-third of pots from each treatment was selected to count boll number, while 10 randomly selected matured plants were picked to determine yield. Seed cotton from these plants was ginned to measure lint yield and lint percentage. Fiber qualities such as length, strength, and micronaire of ginned fiber were analyzed using the USTER HVI MF100 (Uster Technologies AG, Uster, Switzerland).
2.3. Statistical analysis
Agronomic traits were calculated using the following formulas:
The Coefficient of Stress (CS) was measured as (HP-LP)/HP×100%. In order to normalize the indicators of nutrient solution culture and then easy to compare for screening, a subjection value was introduced. The subjection value was calculated as X(ij) (Zhou et al. Citation2003):
X(ij) implied that the subjection value of j cultivar’s i CS. Xij indicated the measured value of the j cultivar’s i CS. Xjmax and Xjmin were the maximum and minimum values of the 16 cultivars, respectively. The CS used in the formula of the subjection value was its absolute value. The subjection value was a decimal between 0 and 1.
Through principal component analysis, 16 cultivars’ subjection values could be divided into some comprehensive indices (CIn), its weight (IW) showed the importance in all comprehensive indices:
pnj indicated j cultivar’s n contribution. And then, the overall P sensitivity (D) evaluated by CIn of each cultivar:
X(nj) indicated the subjection value of j cultivar’s n value, which had the same calculation formula with X(ij).
The experimental data were analyzed using analysis of variance (ANOVA), correlation analysis, cluster analysis (system clustering analyses by the Euclidean distance square method), and principal component analysis in the SPSS 22.0 software (SPSS Software Inc., USA). Figures were drawn by the Origin 2017 software (OriginLab Inc., USA). In addition, the least significant difference (LSD) test was used to compare statistically significant differences at the P< 0.05 level.
3. Results
3.1. Cluster analysis by the subjection value from nutrient solution culture
Biomass was the main index for cotton growth at the seedling stage. The correlation coefficient between the subjection value of total biomass and other 20 indices illustrated that there were most significant correlations between leaf area, leaf biomass, stem biomass, aboveground biomass, root P accumulation, and the total biomass both in 2015 and 2016 (, Supplementary Table S4). Thus, the five indices based on the total biomass had a certain directive function to evaluate cotton seedling growth for low-P tolerance. The stronger P sensitivity of a cultivar index showed higher subjection value. For example, the RPA’s subjection value of Lu 54 (Z1) in 2015 was 1.000, which meant the RPA of Lu 54 was the most low-P sensitive in 2015. Similarly, the RPA’s subjection value of Yuzaomian 9110 (Z6) in 2015 was 0.000, meaning that the RPA of Yuzaomian 9110 was the most low-P tolerant in 2015. The subjection value of leaf area, leaf biomass, stem biomass, aboveground biomass, root P accumulation and total biomass of the 16 cultivars were used for performing system clustering analyses by the Euclidean distance square method (, Supplementary Table S3). According to the cluster results, 16 cultivars could be divided into three types: low-P sensitive cultivars, low-P moderate sensitive cultivars, and low-P tolerant cultivars (). It can be observed that Lu 54 (Z1) was a low-P sensitive cultivar in each index’s clustering, whereas Yuzaomian 9110 (Z6) was a low-P tolerant cultivar. They are steady in this clustering analysis.
Table 1. Subjection value of low-P stress coefficient from nutrient solution culture among 16 cotton cultivars in 2015 and 2016.
Table 2. Clustering results of subjection value of low-P stress coefficient from nutrient solution culture among 16 cotton cultivars in 2015 and 2016.
Figure 1. Correlation coefficients between subjection values of 16 cotton seedlings’ total biomass and other 21 indices from nutrient solution culture in 2015 and 2016. PH: plant height, LN: leaf number, SPAD: SPAD of functional leaf, LA: leaf area, RL: root length, LB: leaf biomass, SB: stem biomass, RB: root biomass, AB: aboveground biomass, TB: total biomass, SLW: specific leaf weight, RSR: root/shoot ratio, LPA: leaf P accumulation, SPA: stem P accumulation, RPA: root P accumulation, APA: aboveground P accumulation, TPA: total P accumulation, PEC: P efficiency coefficient, PUE: P utilization efficiency, MDAC: malondialdehyde content of functional leaf, SC: sucrose content of functional leaf. * indicate significance at 0.05 probability level; ** indicate significance at 0.01 probability level; n = 192, R0.05 = 0.4973, R0.01 = 0.6226.
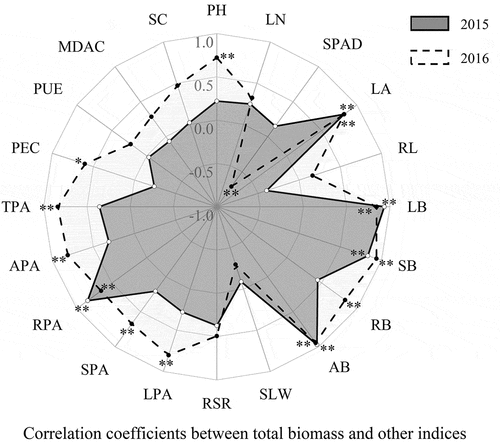
While using another analysis (principal component analysis), the cumulative contributions of six comprehensive indices were 86.03% and 87.69% in 2015 and 2016, respectively (Supplementary Table S5). In 2015, CI1 to CI6 contributed 37.07%, 22.98%, 15.67%, 11.06%, 7.72% and 5.50%, respectively. In 2016, CI1 to CI6 contributed 54.65%, 15.31%, 11.72%, 7.30%, 5.82% and 5.20%, respectively. The D values of Lu 54 in 2015 and 2016 were both at maximum, indicating that its low-P sensitivity was the best compared to other 15 cultivars. The D value of Yuzaomian 9110 in 2015 was at a minimum and in 2016 was the second smallest, which indicated that it could resist low-P stress. The screened two cultivars of principal component analysis were the same as they were in cluster analysis.
3.2. Seedling agronomic traits, lint yield and fiber quality in two cotton cultivars
Plant height (), leaf number (), leaf area (), P accumulation () and biomass in various organs () of LP treatment were relatively lower compared with HP treatment in both two cultivars at the seedling stage. Meanwhile, the P accumulation percentages in various organs changed under P deficient conditions. The P accumulation percentage of the root increased, while the P accumulation percentage of the leaf decreased (). Compared with the agronomic traits of Lu 54, Yuzaomian 9110 exhibited a smaller decline in plant height, leaf number, leaf area and total P accumulation at LP treatment. The root P accumulation of Yuzaomian 9110 retained a relatively high level and the stem P accumulation percentage was stable at 24.16% and 23.52% in 2015 and 2016, respectively. The leaf and total biomass of Yuzaomian 9110 showed no significant difference between HP and LP treatments in both years ().
Table 3. P accumulation of two cotton cultivars under 0.01 mM (LP) and 1 mM KH2PO4 (HP) levels at the seedling stage in 2015 and 2016.
Table 4. Biomass of two cotton cultivars under 0.01 mM (LP) and 1 mM KH2PO4 (HP) levels at the seedling stage in 2015 and 2016.
Figure 2. Plant height of Lu 54 and Yuzaomian 9110 under 0.01 mM (LP) and 1 mM KH2PO4 (HP) levels at the seedling stage in 2015 and 2016. The different letters from each year are significantly different at P = 0.05. Each value denotes the mean of three replications.
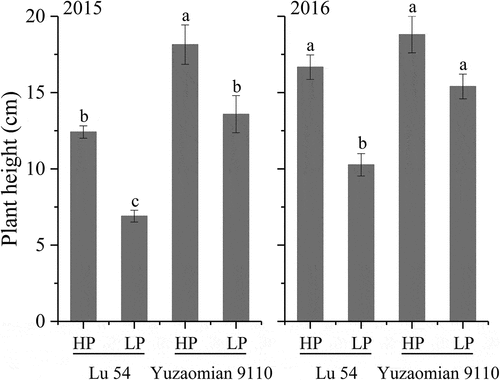
Figure 3. Leaf number of Lu 54 and Yuzaomian 9110 under 0.01 mM (LP) and 1 mM KH2PO4 (HP) levels at the seedling stage in 2015 and 2016. The different letters from each year are significantly different at P = 0.05. Each value denotes the mean of three replications.
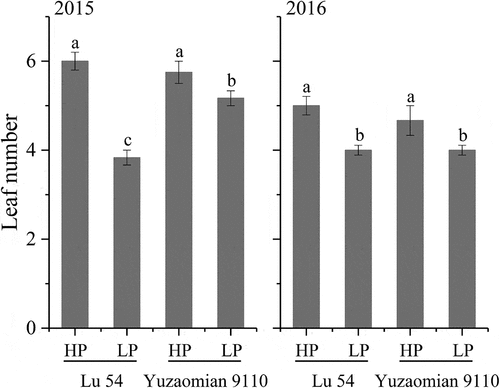
Figure 4. Leaf area of Lu 54 and Yuzaomian 9110 under 0.01 mM (LP) and 1 mM KH2PO4 (HP) levels at the seedling stage in 2015 and 2016. The different letters from each year are significantly different at P = 0.05. Each value denotes the mean of three replications.
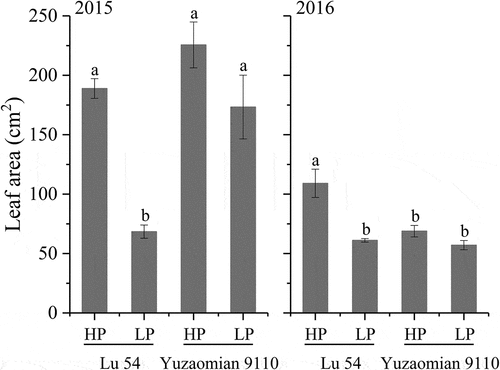
Different P fertilizer rates made different soil Olsen-P. Setting five P fertilizer rates could ensure that they could supply different P levels to cotton. In a two-year pot experiment, low P conditions limited the plant growth and development showing fewer boll numbers and lower lint percentage, resulting a low lint yield (). Meanwhile, low P supply levels decreased the fiber strength and increased the micronaire of both cultivars (). Compared with Lu 54, Yuzaomian 9110 exhibited a smaller decline in boll number, lint percentage as well as lint yield, and could retain a relatively stable seed cotton weight per boll and fiber length.
Table 5. Effects of P application rates on cotton lint yield and yield components during the year 2016 and 2017 in Lu 54 and Yuzaomian 9110.
Figure 5. Effects of P application rates on the main cotton fiber properties during the year 2016 and 2017 in Lu 54 and Yuzaomian 9110 (A: fiber length, B: fiber strength, C: micronaire). Each value denotes the mean of three replications. Three-way analysis of variance (ANOVA) was performed to evaluate the effects of cultivar (C), P rate (P) and their interactions (C × P). NS means non-significant difference; * and ** mean significance at the 0.05 and 0.01 levels of probability.
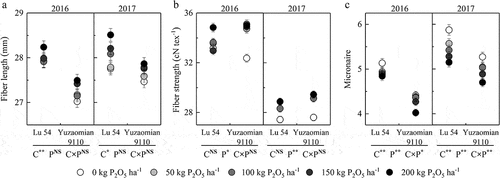
4. Discussion
Cotton is an important industrial crop and entails a great economic significance. The development of predominant cultures is distinct with regard to their phenotype, response to P deficiency and yield attributes. To evaluate the effect of low-P tolerance in cotton growth, yield and fiber quality, screening low-P tolerant and low-P sensitive cultivars is the first. Biomass and P accumulation are important indices to compare a wide range of genotypes at the seedling stage (Wang et al. Citation2009; Li et al. Citation2010; Chen et al. Citation2014). Importantly, biomass is considered as the key index for the morphogenesis of cotton seedlings. In the present study, only five indices’ subjection values were found to be significantly correlated with the subjection value of total biomass in 2015 and 2016 (, Supplementary Table S4). Lu 54 and Yuzaomian 9110 screened by these six indices ( and ) were proved the same in the principal component analysis (Supplementary Table S5).
Moreover, Lu 54 and Yuzaomian 9110 had the greatest difference in terms of agronomic traits at the seedling stage. The P application increased the difference in growth traits between Lu 54 and Yuzaomian 9110 that might be due to different sensitivities. This was clearly demonstrated in contours such as plant height (), leaf number (), leaf area (), P accumulation, and biomass ( and ) in various organs of seedlings. The addition of P significantly increased the P concentration of the two cultivars in the present study (). But the P accumulation in root rather than leaf under the low-P level might be attributed to the restricted P transportation from root to aboveground parts. A similar explanation was documented by Wang et al. (Citation2008) that P deficiency impaired the P acquisition in leaf. This meant that low-P slowed down the growth rate and was unfavorable to the morphogenesis of cotton seedlings. However, compared with Lu 54, Yuzaomian 9110 enhanced the P uptake by roots, increased the root P accumulation under low-P conditions. Similarly, P accumulation percentage in its stem was more stable under LP treatment compared to HP treatment. Furthermore, decreased P accumulation percentage of stem and root might enhance the P accumulation percentage of the leaf. In conclusion, stable stem P accumulation percentage ensured the balance between root growth and development and considered as one of the important factors of low-P tolerance.
The P sensitivity varies among different cotton genotypes. But the response of some indices might not be similar in the same genotypes. Unlike the observations of Wang et al. (Citation2008, 2016), not all cultivars (especially Yuzaomian 9110) root, stem and leaf biomass were found to decrease under P deficient conditions in the present study (). Although plants that exposed to P stress could potentially change the biomass allocation between shoot and roots (Marschner, Römheld, and Cakmak Citation1987), but the response of cotton may not be uniform compared to other crops such as soybean (Jin et al. Citation2006), rice (Li, Xia, and Wu Citation2011), and sorghum (Ma et al. Citation2013). Thus, enough P supply could provide a stable transportation from root to leaf and help in a better photosynthesis. This in turn might regulate the physiological metabolism, seedling growth, and yield formation.
The deficiency of P limits the seedling growth and negatively affects the reproductive growth such as boll number, lint yield, and fiber quality. In the present study, although low-P did the similar and badly affected all aforementioned attributes (), but this might help to screen and breed P-tolerant high yield cotton cultivars on the base of seed cotton weight per boll. Because it showed a greatest difference in Lu 54 and Yuzaomian 9110. As per literature, fewer studies reported the effect of low-P on cotton fiber quality. However, in the present study, reduced fiber strength and increased micronaire with no P application was a clear indication toward the negative effect of P deficiency to some extent (,c)). Similarly, it affected the fiber development as difference observed by fiber length and micronaire between Lu 54 and Yuzaomian 9110 (,c)).
5. Conclusion
Based on correlation analysis, cluster analysis, and principal component analysis, Lu 54 (low-P sensitive) and Yuzaomian 9110 (low-P tolerant) were the ideal cultivars to evaluate the effect of low-P tolerance on cotton growth, yield, and fiber quality. As per the findings of the present study, low-P treatment reduced the seedling growth, P accumulation and biomass of seedlings. Furthermore, a low-P application severely affected the boll number, lint yield, fiber strength and micronaire at the harvest in both cultivars. Compared with Lu 54, Yuzaomian 9110 enhanced the P uptake of roots, increased root P accumulation, established a stable stem P accumulation percentage, leaf and total biomass of seedlings, seed cotton weight per boll, and fiber length. This result further explained the effect of seedling growth and development under low-P conditions and could provide a new direction to breeding P-tolerant high yield cotton cultivars.
Supplemental Material
Download MS Excel (67.2 KB)Acknowledgments
We thank the grant support of the National key R&D Program of China and National Key Technology Support Program, the experimental field support of Nanjing Agricultural University. We also thank Mr J. Wang, Mr H. Zhang and other members of our laboratories.
Disclosure statement
No potential conflict of interest was reported by the authors.
Supplementary Material
Supplemental data for this article can be accessed here.
Additional information
Funding
References
- Araújo, É. D. O., E. F. D. Santos, G. Q. D. Oliveira, M. A. Camacho, and D. M. Dresch. 2012. “Nutritional Efficiency Of Cowpea Varieties in The Absorption Of Phosphorus.” Agronomia Colombiana 30 (30): 419–424.
- Asher, C. J., and J. F. Loneragan. 1967. “Response of Plants to Phosphate Concentration in Solution Culture: I. Growth and Phosphorus Content.” Soil Science 103 (4): 225–233. doi:10.1097/00010694-196704000-00001.
- Bovill, W. D., C. Y. Huang, and G. K. Mcdonald. 2013. “Genetic Approaches to Enhancing Phosphorus-use Efficiency (PUE) in Crops: Challenges and Directions.” Crop and Pasture Science 64 (3): 179–198. doi:10.1071/CP13135.
- Chen, B. L., J. D. Sheng, P. A. Jiang, W. H. Li, and L. Wang. 2014. “Differences of Phosphorus Efficiency Characteristics and Root Morphology between Two Cotton Genotypes.” Cotton Science 26 (6): 506–512. (in Chinese).
- Fageria, N. K., A. M. Knupp, and M. F. Moraes. 2013. “Phosphorus Nutrition of Lowland Rice in Tropical Lowland Soil.” Communications in Soil Science and Plant Analysis 44 (44): 2932–2940. doi:10.1080/00103624.2013.829485.
- Fageria, N. K., V. C. Baligar, A. Moreira, and T. A. Portes. 2010. “Dry Bean Genotypes Evaluation for Growth, Yield Components and Phosphorus Use Efficiency.” Journal of Plant Nutrition 33 (14): 2167–2181. doi:10.1080/01904167.2010.519089.
- Föhse, D., N. Claassen, and A. Jungk. 1988. “Phosphorus Efficiency of Plants. 1. External and Internal P Requirement and P Uptake Efficiency of Different Plant Species.” Plant and Soil 110 (1): 101–109. doi:10.1007/BF02143545.
- Gahoonia, T. S., and N. E. Nielsen. 2004. “Barley Genotypes with Long Root Hairs Sustain High Grain Yields in low-P Field.” Plant and Soil 262 (1–2): 55–62. doi:10.1023/B:PLSO.0000037020.58002.ac.
- Goldstein, A. H., D. A. Baertiein, and R. G. Mcdaniel. 1988. “Phosphate Starvation Inducible Metabolism in Lycopersicon Esculentum: I. Excretion of Acid Phosphatase by Tomato Plants and Suspension-cultured Cells.” Plant Physiology 87 (3): 711–715. doi:10.1104/pp.87.3.711.
- Hendrix, D. L. 1993. “Rapid Extraction and Analysis of Nonstructural Carbohydrates in Plant Tissues.” Crop Science 33 (6): 1306–1311. doi:10.2135/cropsci1993.0011183X003300060037x.
- Hernández, G., M. Ramírez, O. Valdéslópez, M. Tesfaye, M. A. Graham, T. Czechowski, and A. Schlereth. 2007. “Phosphorus Stress in Common Bean: Root Transcript and Metabolic Responses.” Plant Physiology 144 (2): 752–767. doi:10.1104/pp.107.096958.
- Hoagland, D. R., and D. I. Arnon. 1950. “The Water-culture Method for Growing Plants without Soil.” California Agricultural Experiment Station Circular 347: 35–37.
- Jin, J., G. H. Wang, X. B. Liu, X. L. Chen, and X. G. Li. 2006. “Effect of Different Phosphorus Regimes on Root Morphological Characteristics of Soybean Seedling.” Soybean Science 25 (4): 360–364. (in Chinese).
- Li, H. B., M. Xia, and P. Wu. 2011. “Effect of Phosphorus Deficiency Stress on Rice Lateral Root Growth and Nutrient Absorption.” Chinese Bulletin of Botany 43 (11): 1154–1160. (in Chinese).
- Li, W. H., J. D. Sheng, B. L. Chen, D. L. Fu, and J. Li. 2010. “Studies on Screening Cotton Varieties by High Efficient Use of Phosphorous Nutrient.” Journal of Xinjiang Agricultural University 33 (2): 109–115. (in Chinese).
- Lu, R. K. 1999. Method for Agro-chemical Analyses of Soil. Beijing: Agricultural, Science and Technology Press of China. (in Chinese).
- Ma, J. H., Y. G. Wang, Y. Sun, M. Q. Yin, C. Q. Niu, and Y. J. Yang. 2013. “Effects of Low Phosphorous Stress on the Morphologies and Physiological Indices of Different Sorghum Cultivars at Seedling Stage.” Journal of Plant Nutrition and Fertilizer 19 (5): 1083–1091. (in Chinese).
- Marschner, H., V. Römheld, and I. Cakmak. 1987. “Root-induced Changes of Nutrient Availability in the Rhizosphere.” Journal of Plant Nutrition 10 (9): 1175–1184. doi:10.1080/01904168709363645.
- Pavinato, P. S., T. H. Dao, and C. A. Rosolem. 2010. “Tillage and Phosphorus Management Effects on Enzyme-labile Bioactive Phosphorus Availability in Cerrado Oxisols.” Geoderma 156 (3–4): 207–215. doi:10.1016/j.geoderma.2010.02.019.
- Pettigrew, W. T. 2001. “Environmental Effects on Cotton Fiber Carbohydrate Concentration and Quality.” Crop Science 41 (4): 1108–1113. doi:10.2135/cropsci2001.4141108x.
- Ramaekers, L., R. Remans, I. M. Rao, M. W. Blair, and J. Vanderleyden. 2010. “Strategies for Improving Phosphorus Acquisition Efficiency of Crop Plants.” Field Crops Research 117 (2–3): 169–176. doi:10.1016/j.fcr.2010.03.001.
- Rodríguez, D., M. M. Zubillaga, E. L. Ploschuk, W. G. Keltjens, J. Goudriaan, and R. S. Lavado. 1998. “Plant Leaf Area Expansion and Assimilate Production in Wheat (Triticum Aestivum L.) Growing under Low Phosphorus Conditions.” Plant and Soil 200 (2): 227–240. doi:10.1023/A:1004310217694.
- Rose, T. J., A. Mori, C. C. Julia, and M. Wissuwa. 2016. “Screening for Internal Phosphorus Utilisation Efficiency: Comparison of Genotypes at Equal Shoot P Content Is Critical.” Plant and Soil 401 (1–2): 79–91. doi:10.1007/s11104-015-2565-7.
- Santos, E., N. Marcante, T. Muraoka, and M. Camacho. 2015. “Phosphorus Use Efficiency in Pima Cotton (Gossypium Barbadense L.) Genotypes.” Chilean Journal of Agricultural Research 75 (2): 210–215. doi:10.4067/S0718-58392015000200010.
- Wang, G., C. S. Zheng, P. C. Li, J. R. Liu, A. Z. Liu, M. Sun, X. H. Zhao, X. K. Yu, and H. L. Dong. 2016. “Effects of Soil Olsen-P Levels on the Dry Matter Accumulation and Carbon and Nitrogen Metabolism of Cotton Plant at Seedling Stage.” Cotton Science 28 (6): 609–618. (in Chinese).
- Wang, S. J., X. F. Wang, Z. Y. Ma, G. Y. Zhang, X. H. Li, and L. Q. Wu. 2009. “Screening of Low Phosphorus Tolerant Cottons with Fusarium and Verticillium Wilts Resistance at Seedling Stage.” Cotton Science 21 (3): 168–174. (in Chinese).
- Wang, X., C. Tang, C. N. Guppy, and P. W. G. Sale. 2008. “Phosphorus Acquisition Characteristics of Cotton (Gossypium Hirsutum L.), Wheat (Triticum Aestivum L.) And White Lupin (Lupinus Albus L.) Under P Deficient Conditions.” Plant and Soil 312 (1/2): 117–128. doi:10.1007/s11104-008-9589-1.
- Yan, X., J. P. Lynch, and S. E. Beebe. 1996. “Utilization of Phosphorus Substrates by Contrasting Common Bean Genotypes.” Crop Science 36 (4): 936–941. doi:10.2135/cropsci1996.0011183X003600040020x.
- Zambrosi, F. C. B., D. Mattos Jr, P. R. Furlani, J. A. Quaggio, and R. M. Boaretto. 2012. “Efficiency of Phosphorus Uptake and Utilization in Citrus Rootstocks.” Maneuvering 36 (2): 485–496.
- Zhao, J. Q., F. Q. Zhao, G. L. Jian, Y. X. Ye, W. W. Zhang, J. S. Li, and F. J. Qi. 2013. “Intensified Alternaria Spot Disease under Potassium Deficiency Conditions Results in Acceleration of Cotton (Gossypium Hirsutum L.) Leaf Senescence.” Australian Journal of Crop Science 7 (2): 241–248.
- Zhou, G. S., F. Z. Mei, Z. Q. Zhou, and X. T. Zhu. 2003. “Comprehensive Evaluation and Forecast on Physiological Indices of Water-logging Resistance of Different Wheat Varieties.” Scientia Agricultura Sinica 36 (1): 1378–1382. (in Chinese).