ABSTRACT
Cadmium (Cd) and lead (Pb) are toxic trace elements which are not essential for plants but can be easily taken up by roots and accumulated in various organs, and cause irreversible damages to plants. A pot experiment was carried out to investigate the individual and combined effects of Cd (0, 10, 20 mg kg−1) and Pb (0, 500, 1000 mg kg−1) level in a calcareous soil on the status of mineral nutrients, including K, P, Ca, Mg, S, Fe, Mn, Cu, and Zn, in alfalfa (Medicago sativa L.) plants. Soil Pb level considerably (P ≤ 0.05) affected the concentrations of more elements in plants than soil Cd level did, and there were combined effects of soil Cd level and Pb level on the concentrations of some nutrients (Ca, Mg, and Cu) in plants. The effects of soil Cd level and Pb level on plant nutrient concentrations varied among plant parts. Cd and Pb contamination did not considerably affect the exudation of carboxylates in the rhizosphere. An increase in rhizosphere pH and exudation of significant amounts of carboxylates (especially oxalate) in the rhizosphere might contribute to the exclusion and detoxification of Cd and Pb. Neither shoot dry mass nor root dry mass was significantly influenced by soil Cd level, but both of them were considerably reduced (by up to 25% and 45% on average for shoot dry mass and root dry mass, respectively) by increasing soil Pb level. The interaction between soil Cd level and Pb level was significant for root dry mass, but not significant for shoot dry mass. The results indicate that alfalfa is tolerant to Cd and Pb stress, and it is promising to grow alfalfa for phytostabilization of Cd and Pb on calcareous soils contaminated with Cd and Pb.
1. Introduction
Cadmium (Cd) and lead (Pb) are toxic trace elements which have no biological functions and are naturally present in soils at low concentrations. However, they have become common and abundant toxic elements in soils and caused environmental and human problems in many parts of the world, due to the contamination of soils with Cd and Pb resulting from anthropogenic activities such as mining and processing of metals, and application of fertilizers (Clemens et al. Citation2013; Kushwaha et al. Citation2018). Although Cd and Pb are not essential for plants, they can be easily taken up by roots and accumulated in various organs, and cause irreversible damages to plants, including stunted plant growth and reproduction, altered uptake and distribution of mineral nutrients, etc. (Gielen, Vangronsveld, and Cuypers Citation2017; Gupta et al. Citation2017; Li et al. Citation2012; Zahedifar et al. Citation2016).
In the soil-plant system, many elements have similar chemical properties and interact with each other, and there is often a multi-element response in plants to an environmental stimulus, which usually creates a co-ordinated response of a series of physiological processes starting in the rhizosphere and ending with evapotranspiration and phloem recycling (Baxter Citation2015; Moosavi, Mansouri, and Zahedifar Citation2015; Moosavi, Zahedifar, and Mansouri Citation2018; alt, Baxter, and Lahner Citation2008). There are a few strategies plants employ to tolerate Cd and Pb, including exuding organic acids to sequester Cd and Pb (Kim et al. Citation2010; Pidatala et al. Citation2016), increasing rhizosphere pH to reduce the bioavailability of Cd and Pb (Kim et al. Citation2010), etc. Exudation of organic acids and alteration of the rhizosphere pH are likely to affect the bioavailability of multiple mineral nutrients in soils (Baxter Citation2015; Lambers, Pons, and Chapin Citation2008; Podar, Ramsey, and Hutchings Citation2004).
When rice (Oryza sativa) seedlings were treated with exogenous Cd, the uptake of calcium (Ca) and potassium (K) was impaired, Ca and K concentrations in both roots and shoots were significantly reduced (Li et al. Citation2012). Cadmium exposure has been found to induce copper (Cu) deficiency in plants such as Arabidopsis thaliana (Gielen, Vangronsveld, and Cuypers Citation2017). For A. thaliana and tobacco (Nicotiana tabacum) seedlings, Cd treatment increased iron (Fe) concentrations in roots but reduced Fe concentrations in shoots, and caused Fe-deficiency-like symptoms on plants; Cd increased Fe retention in roots and hampered its translocation to shoots (Xu, Lin, and Lai Citation2015; Yoshihara et al. Citation2006). The concentration of Fe in shoots of Indian mustard (Brassica juncea) was found to decrease proportionately with increase in Cd toxicity (Sharmila et al. Citation2017). With an increase in Pb supply, zinc (Zn) concentrations were increased in various parts of cabbage (Brassica oleracea) grown in refined sand with complete nutrient solution, whereas phosphorus (P), sulfur (S), Fe, manganese (Mn), and Cu concentrations were reduced (Sinha et al. Citation2006).
There are a number of studies on the individual effects of Cd and Pb on the status of mineral nutrients (Gielen, Vangronsveld, and Cuypers Citation2017; Li et al. Citation2012; Sinha et al. Citation2006). However, soil contamination is often a multi-element rather than a single-element problem, the effects of combined toxic trace elements contamination on mineral nutrients uptake and distribution by plants may be quite different from those of individual element contamination due to the interactions between elements in the soil-plant system (An et al. Citation2004; Luan, Cao, and Yan Citation2008). Therefore, it is necessary to study the combined effect of toxic trace elements on the status of mineral nutrients.
Alfalfa (Medicago sativa L.) is an important component of the agroecosystem on the Chinese Loess Plateau, where most soils are typically alkaline and calcareous, and it is often used for vegetation restoration and rehabilitation in this area because of its good growth performance (He et al. Citation2017). In this study, we carried out a pot experiment to investigate the individual and combined effects of Cd and Pb contamination on the status of mineral nutrients by alfalfa grown on a calcareous soil on the Loess Plateau, and to assess the potential of using alfalfa for vegetation rehabilitation on calcareous soils contaminated with Cd and Pb.
2. Materials and methods
2.1. Soil preparation
A calcareous soil was collected from the top 40-cm layer of an undisturbed site (39°47′35″N, 111°17′19″E) in the Jungar Banner of Inner Mongolia Autonomous Region, in the northern part of the Chinese Loess Plateau, and used for the pot experiment. The area has a semi-arid temperate continental climate, the mean annual precipitation is 404 mm, and the mean daily temperature is 7.2°C (Zhen et al. Citation2015). The soil was air-dried and screened to pass through a 2-mm sieve before physicochemical characterization. The distribution of soil particle size was analyzed on a Mastersizer 2000 laser diffraction particle size analyzer (Malvern Instruments, Malvern, UK) (Lamorski et al. Citation2014), and field capacity of the soil was determined by the indoor cutting-ring weighing method. Electrical conductivity (EC) and pH of the soil were measured in a 1:5 soil:water (w:v) suspension. Organic carbon (C) concentration in the soil was determined using the potassium dichromate titrimetric method (Mebius Citation1960). Ammonium-nitrogen (NH4+-N) and nitrate-nitrogen (NO3–N) concentrations were analyzed on a segmented-flow analyzer after extraction of the soil by a 2 M potassium chloride (KCl) solution [soil:solution = 1:10 (w:v)] (Kachurina et al. Citation2000). Concentrations total K, P, Ca, Mg, S, Fe, Mn, Cu, Zn, Cd, and Pb in the soil were analyzed on a inductively coupled plasma mass spectrometer (ICP-MS, ELAN 9000, PerkinElmer Instruments, Shelton, CT, USA) following a modified aqua regia digestion procedure (Reimann et al. Citation2015). The physicochemical properties of the soil are presented in .
Table 1. Physicochemical properties of the loessial soil.
The pot experiment was set in a two-factorial completely randomized design with three replications. Soil Cd level was designed as 0, 10, and 20 mg Cd kg−1 soil (hereafter referred to as Cd0, Cd10, and Cd20 treatment, respectively), and soil Pb level was designed as 0, 500, and 1000 mg Pb kg−1 soil (hereafter referred to as Pb0, Pb500, and Pb1000 treatment, respectively), to make a total of 16 (4 × 4) treatments, and there were six pots for each treatment. PVC tubes of 15-cm diameter and 20-cm height were used for the pots to grow plants. Each pot was first filled with 4 kg of the sieved soil, and then the soil was watered to 21% gravimetric soil water content (about 60% field capacity of the filled soil) with deionized (DI) water. To spike the soil with Cd and Pb at the designated levels, the total required amount of Pb(NO3)2 and CdCl2 was weighed and dissolved in DI water separately with a known volume; then, the volume of the solution required for each pot was calculated according to the treatment, and the solution was applied to the soil at the calculated volume.
To attain the quasi-equilibrium distribution of Cd and Pb, the spiked soil was subsequently placed at ambient temperature under a rain shelter and left undisturbed, except that the soil was watered to 21% gravimetric soil water content with DI water weekly during the spring, summer, and autumn, but the water was withheld during the winter. After 12-month equilibration, the soil in each pot was taken out and put in a plastic bag to be air-dried and sieved, and the sieved soil in the six pots of the same treatment was mixed thoroughly and split into six equal parts, with each part being 4 kg. To facilitate plant growth, phosphorus (P) was mixed with the soil at 20 mg P kg−1 soil in the form of calcium superphosphate, which was ground to a fine powder before mixing. The P required for each pot was mixed separately with the soil. Before filling, each pot was lined with a transparent plastic bag inside first and the bottom was covered with a layer of gravels; then, 4 kg of the thoroughly mixed soil was filled to the pot and watered to 21% gravimetric soil water content with DI water, and left undisturbed in a glasshouse for 1 week before seeds were sown.
2.2. Plant cultivation
Seeds of Medicago sativa L. cv Golden Empress were first sterilized for 5 min in 30% (v:v) hydrogen peroxide (H2O2) solution, then rinsed with cold sterile DI water three times and placed on moistened filter papers in petri dishes overnight (Kereszt et al. Citation2007). In early October 2016, three pots of each treatment were sown with 30 seeds per pot, and the remaining three pots of each treatment were not sown with seeds and served as blank pots. Seedlings in each pot were thinned to 20 plants 4 weeks after sowing. The experiment was carried out from early October 2016 to late February 2017 for 100 days in a glasshouse in the Institute of Soil and Water Conservation, Yangling, Shaanxi Province, China. During the experiment, the soil in all pots was watered to 60% field capacity with DI water every 3 days.
2.3. Plant biomass measurement and rhizosphere carboxylates analysis
Plants were harvested 100 days after sowing. Shoots were cut at the stem base; then, the plastic bags were lifted out of the pots, and the soil was gently pressed to loosen the soil and separate the roots from the soil. Rhizosphere extracts of Cd0 and Cd10 in Pb0 and Pb500 were selected for the analysis of rhizosphere carboxylates. For each pot with plants, about 1.0 g fresh fine roots and the attached rhizosphere soil was soaked in about 20 mL of 0.2 mM CaCl2 aqueous solution with known volume in a glass beaker for 20 min to extract the rhizosphere carboxylates (He et al. Citation2017). To ensure cell integrity and remove the rhizosphere soil, roots were gently stirred in the solution while soaked. A subsample of the rhizosphere extract was filtered through a 0.22-μm syringe filter into a 1-mL HPLC vial; then, the filtered extract was acidified by a drop of concentrated phosphoric acid and kept in a − 20°C freezer until analysis. The remaining unfiltered extract was measured using a pH meter to obtain the pH. Roots in the extract were collected and washed with tap water, then oven-dried at 60°C for 48 h and weighed to obtain the dry mass of soaked roots. The unfiltered extract was oven-dried together with the beaker at 105°C for 12 h and weighed to obtain the dry weight of the rhizosphere soil.
After harvesting, leaves were separated into young leaves and old leaves, with the top one-third of all leaves on the shoot taken as young leaves, and the remaining taken as old leaves. Roots not soaked for rhizosphere carboxylates extraction were washed thoroughly with tap water first to remove soil particles and then rinsed with DI water. Young leaves, old leaves, stems, and roots were oven-dried at 60°C for 72 h and weighed. Shoot dry mass was calculated as the sum of the dry mass of young leaves, old leaves, and stems; root dry mass was calculated as the sum of the dry mass of soaked roots and un-soaked roots. Root mass ratio was calculated as root dry mass divided by the sum of root and shoot dry mass.
The analysis of carboxylates was performed using a Waters E2695 HPLC equipped with a Waters 2998 detector and Waters X select columns T3 (Waters, Milford MA, USA), and the data were collected at 210 nm (He et al. Citation2017). The working standards for the analysis included acetic acid, oxalic acid, malic acid, malonic acid, citric acid, tartaric acid, and succinic acid. However, only oxalate, malate, malonate, and citrate were detected for all analyzed samples. The amounts of rhizosphere carboxylates were expressed on root dry mass basis and on rhizosphere soil dry mass basis as well.
2.4. Analyses of mineral nutrients in plant parts
Oven-dried young leaves, old leaves, stems, and un-soaked roots were finely ground using a ceramic mortar. To extract total K, P, Ca, magnesium (Mg), S, Fe, Mn, Cu, and Zn from the ground plant samples, about 0.1 g of each ground sample was digested with aqua regia and H2O2 (Lomonte et al. Citation2008; Sastre et al. Citation2002), and the digested solution was made to a final volume of 25 mL. Concentrations of the above-mentioned elements in the digested solution were analyzed using a NexION 300 Inductively Coupled Plasma Mass Spectrometer (ICP-MS) (PerkinElmer, Inc., Waltham, MA, USA).
2.5. Soil pH measurement
When plants were harvested, all bulk soil in each pot with plants and the soil in each blank pot was collected and air-dried, then passed through a 2-mm sieve. Subsamples of the sieved bulk soil and blank pot soil were taken to measure the pH in a 1:5 soil:water suspension using a pH meter (Little Citation1992).
2.6. Statistical analysis
The effects of soil Cd level, soil Pb level, and their interaction on shoot dry mass, root dry mass, root mass ratio, and the amounts of rhizosphere carboxylates were examined by performing a two-way (Cd × Pb) ANOVA. For concentrations of nutrients in plant parts, a three-way (Cd х Pb х plant part) ANOVA was performed to evaluate the influences of soil Cd level and soil Pb level, to show the variations among plant parts, and to investigate whether there was any interaction among the three factors. The variations in soil and rhizosphere pH were tested with a three-way ANOVA, including factors of soil Cd level, soil Pb level, and medium type (i.e., blank pot soil, bulk soil in pot with plants, and rhizosphere extract). All ANOVAs mentioned above were performed using the general linear model in the IBM SPSS Statistics 22.0 software package (IBM, Montauk, New York, USA). The differences were determined to be significant at P ≤ 0.05, and the least significant difference (LSD) test for post-hoc means comparisons was performed when there were significant differences.
3. Results
3.1. Biomass accumulation and partitioning
Neither shoot dry mass (SDM), root dry mass (RDM), nor root mass ratio (RMR) was significantly influenced by soil Cd level, but all of them were considerably affected by soil Pb level (, ). The interaction between soil Cd level and Pb level was significant for RDM and RMR, but not significant for SDM. SDM significantly (P = 0.002 and P < 0.001, respectively) decreased in Pb500 and Pb1000, which had an average of 17% and 25% less SDM than Pb0 ()). However, SDM did not differ considerably between Pb500 and Pb1000. In Pb0, RDM decreased by 9% in Cd10, but did not change in Cd20, compared with Cd0 ()); in Pb500, it increased by 19% and 7% in Cd10 and Cd20, respectively, compared with Cd0; while in Pb1000, it decreased with increasing soil Cd level, being 22% and 68% less in Cd10 and Cd20, respectively, compared with Cd0. In Cd0, RDM in Pb500 and Pb1000 was 35% and 25% less than in Pb0; in both Cd10 and Cd20, it decreased with increasing soil Pb level, being 15% and 35% less in Pb500 and Pb1000 than in Cd10, and 31% and 76% less in Pb500 and Pb1000 than in Cd20, respectively. RMR did not change with soil Cd level in Pb0 ()), but it rose by 9% and 4% in Cd10 and Cd20 than in Cd0 in Pb500, while it declined by 12% and 41% in Cd10 and Cd20 than in Cd0 in Pb1000. In Cd0, RMR declined by 11% in Pb500, but did not change in Pb1000, compared with Pb0; in Cd10, it was 3% and 13% lower in Pb500 and Pb1000, respectively, compared with Pb0; while in Cd20, it declined by 7% and 41% in Pb500 and Pb1000, respectively, compared with Pb0.
Table 2. Statistical level of significance (P value) of the ANOVAs for dry mass accumulation and partitioning (two-way ANOVA, Cd × Pb), and concentrations of various mineral nutrients in plant parts (three-way ANOVA, Cd × Pb × plant part).
Figure 1. Biomass accumulation and partitioning of alfalfa plants grown on a calcareous soil spiked with different levels of cadmium (Cd) and lead (Pb). (a) Shoot dry mass, (b) Root dry mass, and (c) Root mass ratio. Cd0, Cd10, and Cd20 represent soil Cd level of 0, 10, and 20 mg kg−1, respectively; Pb0, Pb500, and Pb1000 represents soil Pb level of 0, 500, and 1000 mg kg−1, respectively. Data are presented as means ± SE (n = 3). Different lower-case letters above the bars indicate significant (P ≤ 0.05) differences among treatments according to the results of LSD test of two-way (Cd × Pb) ANOVA.
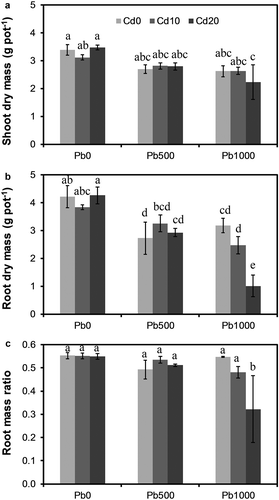
3.2. Concentrations of mineral nutrients in plant parts
Concentration of none of the nutrients measured was significantly influenced by soil Cd level, only S (P = 0.032) and Cu (P = 0.046) concentrations were markedly influenced by soil Pb level (–, ). There were significant interactions between soil Cd and Pb level for Ca (P = 0.003), Mg (P = 0.024), and Cu (P = 0.004) concentrations. Concentrations of all nutrients measured varied considerably among plant parts (all P < 0.001). The interaction between soil Cd level and plant part was only significant for P concentration (P = 0.037). There were significant interactions between soil Pb level and plant part for all nutrients measured except P and S. The interactions among soil Cd level, soil Pb level, and plant part were significant for Ca (P = 0.007), Fe (P = 0.001), Cu (P = 0.001), and Zn (P = 0.011) concentrations.
Figure 2. Concentrations of (a) potassium (K), and (b) phosphorus (P) in different parts of alfalfa plants grown on a calcareous soil spiked with different levels of cadmium (Cd) and lead (Pb). Cd0, Cd10, and Cd20 represent soil Cd level of 0, 10, and 20 mg kg−1, respectively; Pb0, Pb500, and Pb1000 represents soil Pb level of 0, 500, and 1000 mg kg−1, respectively. Data are presented as means ± SE (n = 3). Different lower-case letters above the bars indicate significant (P ≤ 0.05) differences among combinations of Cd × Pb × Plant part according to the results of LSD test of three-way ANOVA.
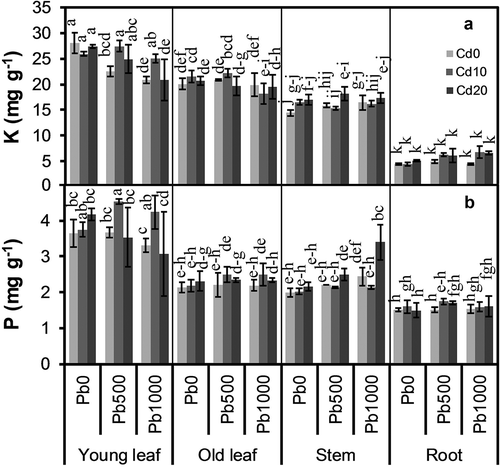
Figure 3. Concentrations of (a) calcium (Ca), (b) magnesium (Mg), and (c) sulfur (S) in different parts of alfalfa plants grown on a calcareous soil spiked with different levels of cadmium (Cd) and lead (Pb). Cd0, Cd10, and Cd20 represent soil Cd level of 0, 10, and 20 mg kg−1, respectively; Pb0, Pb500, and Pb1000 represents soil Pb level of 0, 500, and 1000 mg kg−1, respectively. Data are presented as means ± SE (n = 3). Different lower-case letters above the bars indicate significant (P ≤ 0.05) differences among combinations of Cd × Pb × plant part according to the results of LSD test of three-way ANOVA.
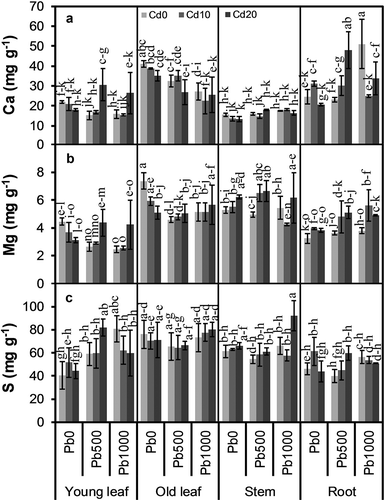
Figure 4. Concentrations of (a) iron (Fe), (b) manganese (Mn), (c) copper (Cu), and (d) zinc (Zn) in different parts of alfalfa plants grown on a calcareous soil spiked with different levels of cadmium (Cd) and lead (Pb). Cd0, Cd10, and Cd20 represent soil Cd level of 0, 10, and 20 mg kg−1, respectively; Pb0, Pb500, and Pb1000 represents soil Pb level of 0, 500, and 1000 mg kg−1, respectively. Data are presented as means ± SE (n = 3). Different lower-case letters above the bars indicate significant (P ≤ 0.05) differences among combinations of Cd × Pb × plant part according to the results of LSD test of three-way ANOVA.
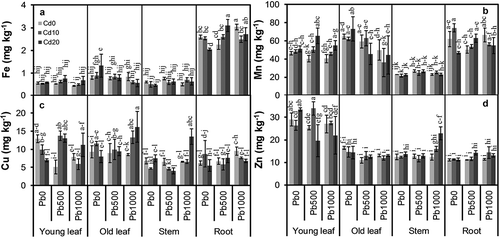
Mean Ca concentration in stems was significantly lower than those in other parts (P ≤ 0.048), while old leaves had considerably higher Ca concentrations than young leaves (P < 0.001) ()); for Pb500 and Pb1000, Ca concentrations in young leaves in Cd20 were higher than those in Cd0 and Cd10; mean Ca concentration in old leaves decreased with increasing soil Pb level, as well as increasing soil Cd level; Ca concentration in roots increased with increasing soil Cd level in Pb500. Mean Fe concentration was considerably higher in roots than in other parts (P < 0.001), while young leaves and stems had similarly the lowest Fe concentrations among all parts ()); Fe concentration in young leaves in Pb500 and Pb1000, in old leaves in Pb0, and in roots in Pb500 increased with increasing soil Cd level, but Fe concentration in old leaves in Pb1000 and in stems in Pb0 decreased with increasing soil Cd level; while Fe concentration in young leaves in Cd0, in old leaves in Cd10 and Cd20 decreased with increasing soil Pb level, but Fe concentration in stems in Cd10 increased with increasing soil Pb level. Mean Cu concentrations in young leaves and old leaves were similar and significantly higher than those in stems and roots (P ≤ 0.001), which were also similar ()); Cu concentration in young leaves in Pb0, in stems in Pb500, and in roots in Pb1000 decreased with increasing soil Cd level, but that in old leaves in Pb1000 increased with increasing soil Cd level. Mean Zn concentration in young leaves was significantly higher than those in other parts (P < 0.001), which were similar ()); in most cases, Zn concentration did not vary considerably with soil Cd level or Pb level, only Zn concentration in stems increased with increasing soil Cd level in Pb1000.
Mean Mg concentrations in old leaves and stems were similar, and considerably higher than those in young leaves and roots (P ≤ 0.001) ()); Mg concentrations in young leaves and old leaves decreased with increasing soil Cd level in Pb0, but in most cases in Pb500 and Pb1000, Mg concentrations in Cd10 and Cd20 were higher than those in Cd0. Mean K concentration was the highest in young leaves, followed by old leaves, stems, and roots ()); young leaves and old leaves had lower K concentrations in Pb1000 than in Pb0 and Pb500, but stems and roots had higher K concentrations in Pb500 and Pb1000 than in Pb0. Among all plant parts, young leaves had the highest P concentration on average, followed by old leaves and stems, which had similar P concentrations, and roots had the lowest P concentration ()); Cd20 resulted in a higher P concentration in stems than Cd0 and Cd10, but not in other parts. Mean Mn concentration was similarly the highest in old leaves and roots, and the lowest in stems ()); mean Mn concentration in old leaves decreased with increasing soil Pb level, but not in other parts. Mean S concentration was the lowest in roots, stems had a similar mean S concentration with both mature leaves and old leaves, while old leaves had a considerably higher mean S concentration than young leaves ()); mean S concentration in Pb1000 was considerably higher than that in Pb0 and Pb500 (P = 0.017 and P = 0.017, respectively), which had similar S concentrations.
3.3. pH of the soil and rhizosphere extract
The difference among medium types was significant (P < 0.001) (); pH of the rhizosphere extract was markedly higher than that of the soil in blank pots (P < 0.001) and bulk soil (P < 0.001), but there was no significant difference between the soil in blank pots and the bulk soil in pots with plants. Neither soil Cd level nor Pb level significantly affected the pH of the soil and rhizosphere extract. There was no significant interaction between any two of the three factors, i.e., soil Cd level, soil Pb level, and medium type, and there was no significant interaction among the three factors.
Table 3. pH of the blank pot soil, bulk soil, and rhizosphere extract. Data are presented as means ± SE (n = 3). Different lower-case letters after the SE indicate significant differences among combinations of Cd × Pb × medium type according to the results of three-way ANOVA.
3.4. Rhizosphere carboxylates
Plants mainly exuded oxalate, malate, citrate, and malonate into the rhizosphere, of which the average amount was 184, 55, 62, and 53 μmol g−1 root dry mass, respectively ()), and 9.2, 2.8, 3.2, and 2.8 μmol g−1 rhizosphere soil, respectively ()). Neither soil Cd level nor Pb level significantly influenced the amount of any of the four carboxylates mentioned above in the rhizosphere, and there was no significant interaction between soil Cd level and Pb level for any of the four carboxylates, regardless of the calculation method.
Figure 5. Rhizosphere carboxylates exuded by alfalfa plants grown on a calcareous soil spiked with different levels of cadmium (Cd) and lead (Pb). (a) Amounts of rhizosphere carboxylates calculated on root dry mass basis, and (b) Amounts of rhizosphere carboxylates calculated on rhizosphere soil dry mass basis. Cd0 and Cd10 represent soil Cd level of 0 and 10 mg kg−1, respectively; Pb0 and Pb500 represent soil Pb level of 0 and 500 mg kg−1, respectively. Data are presented as means + SE (n = 3). The same lower-case letters above the bars indicate no significant (P ≤ 0.05) differences among treatments according to the results of LSD test of two-way (Cd × Pb) ANOVA.
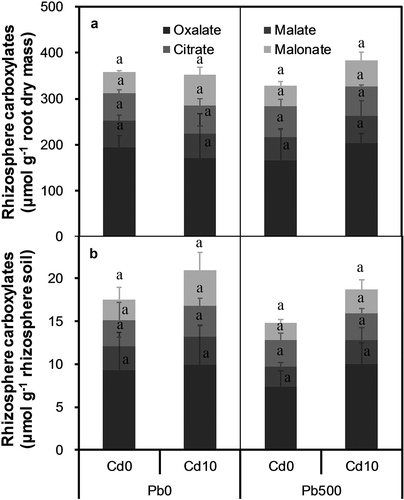
4. Discussion
Cadmium and Pb often inhibit plant growth and result in decreased dry mass in a dose-dependent manner (Gupta et al. Citation2010; Kopittke et al. Citation2007; Perfus-Barbeoch et al. Citation2002). In our study, soil Cd level did not significantly affect plant growth, while soil Pb level affected root dry mass more significantly than shoot dry mass. The significant interaction between soil Cd level and Pb level for alfalfa root dry mass and root mass ratio suggests that the negative effect of Pb on plant growth was exacerbated in the presence of Cd. A number of studies have shown that plant growth suppression under the stress of toxic metals, including Cd and Pb, is linked to the negative impact of toxic metals on the ionic homeostasis in plants (Moosavi, Mansouri, and Zahedifar Citation2015; Moosavi, Zahedifar, and Mansouri Citation2018; Sharmila et al. Citation2017; Yoshihara et al. Citation2006), and the same might be applicable to the present study.
Disturbances in ionic homeostasis of some plant-essential elements in plants exposed to Cd and Pb are often reported, and such disturbances can be caused by ion competition between certain plant-essential element and Cd or Pb during their uptake and/or translocation (Lopes Júnior, Mazzafera, and Zezzi Arruda Citation2014; Sinha et al. Citation2006; Wu et al. Citation2012). The effects of soil Cd level on the concentrations of S and Cu in alfalfa plants were less significant than those of soil Pb level in the present study. The increased S concentrations in young leaves under higher soil Pb levels, and under the interactive effect of higher soil Cd level and soil Pb level were possibly related to the increased phytochelatins (PCs) and/or glutathione (GSH) levels (Ernst et al. Citation2008; Gielen, Vangronsveld, and Cuypers Citation2017; Gupta et al. Citation2010). It has been reported that Cd increased the levels of PCs that can complex both Cd and Cu, thus resulting in Cu deficiency in A. thaliana plants (Gielen, Vangronsveld, and Cuypers Citation2017). Our results showed that Cu concentration in young leaves decreased with increasing soil Cd level when no Pb was added. However, no significant reduction in Cu concentration was observed for other treatment, or for other plant parts; instead, Cu concentrations in young leaves in Pb500 and in old leaves in Pb1000 increased markedly when Cd was added to the soil, the increased Cu concentrations were likely due to the bioconcentration effect resulted from inhibited plant growth. For the alfalfa plants we studied, there was no observed Cd-induced Fe deficiency as that in tobacco seedlings reported by Yoshihara et al. (Citation2006), and there was no observed Cd-induced Zn deficiency as that in A. thaliana reported by Weber, Trampczynska, and Clemens (Citation2006). Although Zn is the chemical analogue of Cd, Zn concentrations in alfalfa plants were relatively constant and not increased by increasing soil Cd level in most cases, very likely because Zn uptake is regulated by the physiological demand of plants (Smolders and Mertens Citation2013).
The results of our study suggest that the effects of soil Cd and Pb contamination on the concentrations of mineral nutrients are dependent on the plant parts, consistent with the results of a number of studies (Hart et al. Citation2005; Rauser Citation2003; Wu, Sato, and Ma Citation2015; Yoshihara et al. Citation2006). For alfalfa plants studied by López et al. (Citation2007), Pb exposure reduced Ca concentrations in stems and leaves of alfalfa plants, Fe and Zn concentrations in roots, and Mn and Mo concentrations in roots and stems, but increased Cu concentrations in roots and stems. A few studies have reported reductions in plant P concentrations (Begonia et al. Citation1998; Geebelen et al. Citation2002; Sinha et al. Citation2006) and speculated that the formation of insoluble Pb-P complexes under Pb excess conditions contributed to P deficiency in plants (Begonia et al. Citation1998). We did not observe P deficiency or a reduction in P concentration in alfalfa plants in the present study, very likely because P was supplied at the optimal level in the studied soil for plant growth (He et al. Citation2017).
Rhizosphere processes such as changes in pH and exudation of organic acids can significantly affect the bioavailability of Cd, Pb, and some mineral nutrients (Kim et al. Citation2010; Lambers, Pons, and Chapin Citation2008; Podar, Ramsey, and Hutchings Citation2004; Wenzel Citation2009). Under Cd and Pb contamination, rhizosphere pH was significantly higher than bulk soil pH in the present study. However, our previous study on the same alfalfa cultivar growing in the same calcareous soil showed that rhizosphere pH was considerably lower than bulk soil pH under P deficiency (He et al. Citation2017). The differences in the pH change between the two studies suggest that rhizosphere pH is greatly affected by other soil chemical properties such as the levels of mineral nutrients and toxic metals. It is very likely that the higher rhizosphere pH, in comparison with bulk soil pH, reduced the bioavailability of Cd and Pb to some degree, thus mitigating the potential toxic effects of Cd and Pb to plants (Kim et al. Citation2010; Smolders and Mertens Citation2013; Steinnes Citation2013).
The amounts and compositions of rhizosphere carboxylates in the present study were different from those under P deficiency in our previous study (He et al. Citation2017), the total amounts of carboxylates exuded by plants in this study were much higher than those under P deficiency. In this study, oxalate accounted for the highest proportion (49–54%) of the total carboxylates measured, while malate, citrate, and malonate accounted for 16%, 18%, and 15%, on average, of the total carboxylates measured. However, malate accounted for the highest proportion (42% on average) of the total carboxylates measured at different soil P levels, followed by oxalate (34% on average) and malonate (23% on average) (He et al. Citation2017). The differences in the amounts and compositions of rhizosphere carboxylates between the two studies suggest that the exudation of carboxylates is strongly affected by soil chemistry, although the amounts and compositions of rhizosphere carboxylates did not vary considerably with soil Cd and Pb levels in this study. Furthermore, the exudation of carboxylates might also vary with the development stage and growth status of the plants.
The results of the present study showed that roots had considerably higher Cd and Pb concentrations than the aerial parts (Figure S1), and only small proportions of Cd and Pb were translocated to the aerial parts (Figure S2). We speculated that the secretion of oxalate played an important role in the exclusion and detoxification of Cd and Pb. The oxalate exuded by roots can immobilize free ions of Cd and Pb in the rhizosphere by producing oxalate-metal complexes, thus helping to exclude Cd and Pb from entering the roots (Wu et al. Citation2016; Zhu et al. Citation2011). Furthermore, Cd and Pb might be sequestered in calcium oxalate (CaOx) crystals in the vacuoles of root cells and thus showed reduced toxicity (Carvalho et al. Citation2015; He et al. Citation2014). It is very likely that the exudation of carboxylates was coincided with the release of cations other than protons (Roelofs et al. Citation2001), as the rhizosphere pH was higher than bulk soil pH, although it is reported that rhizosphere acidification often accompanies carboxylates exudation (Hinsinger et al. Citation2003). The co-release of cations other than protons and carboxylates might have advantage in exclusion and detoxification of toxic metals over the exudation of carboxylic acids, because it has been found that carboxylic acids such oxalic acid and citric acid facilitated the uptake and translocation of some toxic metals (Li et al. Citation2014).
5. Conclusions
Pb contamination considerably affected the concentrations of more mineral nutrients in plants than Cd contamination did, and there were combined effects of soil Cd level and Pb level on the concentrations of some nutrients in plants. The effects of Cd and Pb contamination on plant nutrient concentrations varied among plant parts. Cd and Pb contamination did not considerably affect the exudation of carboxylates in the rhizosphere. An increase in rhizosphere pH and exudation of significant amounts of carboxylates (especially oxalate) in the rhizosphere might contribute to the exclusion and detoxification of Cd and Pb. Neither shoot dry mass nor root dry mass was significantly influenced by soil Cd level, but both of them were considerably reduced by increasing soil Pb level. The interaction between soil Cd level and Pb level was significant for root dry mass, but not significant for shoot dry mass. The results indicate that alfalfa is tolerant to Cd and Pb stress, and it is promising to grow alfalfa for phytostabilization of Cd and Pb on calcareous soils contaminated with Cd and Pb.
Supplemental Material
Download Zip (298.4 KB)Acknowledgments
We sincerely thank Dr. Jiayin Pang from The University of Western Australia for her valuable comments and suggestions during the preparation of this article.
Disclosure statement
The authors have no conflict of interests to disclose.
Supplementary Material
Supplemental data for this article can be accessed here.
Additional information
Funding
References
- An, Y. J., Y. M. Kim, T. I. Kwon, and S. W. Jeong. 2004. “Combined Effect of Copper, Cadmium, and Lead upon Cucumis sativus Growth and Bioaccumulation.” Science of the Total Environment 326: 85–93. doi:10.1016/j.scitotenv.2004.01.002.
- Baxter, I. 2015. “Should We Treat the Ionome as a Combination of Individual Elements, or Should We Be Deriving Novel Combined Traits?” Journal of Experimental Botany 66: 2127–2131. doi:10.1093/jxb/erv040.
- Begonia, G. B., C. D. Davis, M. F. T. Begonia, and C. N. Gray. 1998. “Growth Responses of Indian Mustard [Brassica juncea (L.) Czern.] And Its Phytoextraction of Lead from a Contaminated Soil.” Bulletin of Environmental Contamination and Toxicology 61: 38–43. doi:10.1007/s001289900726.
- Carvalho, M. T. V., P. Pongrac, R. Mumm, J. van Arkel, A. van Aelst, L. Jeromel, P. Vavpetic, P. Pelicon, and M. G. M. Aarts. 2015. “Gomphrena claussenii, a Novel Metal-hypertolerant Bioindicator Species, Sequesters Cadmium, but Not Zinc, in Vacuolar Oxalate Crystals.” New Phytologist 208: 763–775. doi:10.1111/nph.13500.
- Clemens, S., M. G. M. Aarts, S. Thomine, and N. Verbruggen. 2013. “Plant Science: The Key to Preventing Slow Cadmium Poisoning.” Trends in Plant Science 18: 92–99. doi:10.1016/j.tplants.2012.08.003.
- Ernst, W. H. O., G. J. Krauss, J. A. C. Verkleij, and D. Wesenberg. 2008. “Interaction of Heavy Metals with the Sulphur Metabolism in Angiosperms from an Ecological Point of View.” Plant Cell and Environment 31: 123–143. doi:10.1111/j.1365-3040.2007.01746.x.
- Geebelen, W., J. Vangronsveld, D. C. Adriano, L. C. Van Poucke, and H. Clijsters. 2002. “Effects of Pb-EDTA and EDTA on Oxidative Stress Reactions and Mineral Uptake in Phaseolus vulgaris.” Physiologia Plantarum 115: 377–384. doi:10.1034/j.1399-3054.2002.1150307.x.
- Gielen, H., J. Vangronsveld, and A. Cuypers. 2017. “Cd-induced Cu Deficiency Responses in Arabidopsis thaliana: Are Phytochelatins Involved?” Plant Cell and Environment 40: 390–400. doi:10.1111/pce.12876.
- Gupta, D. K., H. G. Huang, X. E. Yang, B. H. N. Razafindrabe, and M. Inouhe. 2010. “The Detoxification of Lead in Sedum alfredii H. Is Not Related to Phytochelatins but the Glutathione.” Journal of Hazardous Materials 177: 437–444. doi:10.1016/j.jhazmat.2009.12.052.
- Gupta, D. K., L. B. Pena, M. C. Romero-Puertas, A. Hernandez, M. Inouhe, and L. M. Sandalio. 2017. “NADPH Oxidases Differentially Regulate ROS Metabolism and Nutrient Uptake under Cadmium Toxicity.” Plant Cell and Environment 40: 509–526. doi:10.1111/pce.12711.
- Hart, J. J., R. M. Welch, W. A. Norvell, J. M. Clarke, and L. V. Kochian. 2005. “Zinc Effects on Cadmium Accumulation and Partitioning in Near-isogenic Lines of Durum Wheat that Differ in Grain Cadmium Concentration.” New Phytologist 167: 391–401. doi:10.1111/j.1469-8137.2005.01416.x.
- He, H., Q. Peng, X. Wang, C. Fan, J. Pang, H. Lambers, and X. Zhang. 2017. “Growth, Morphological and Physiological Responses of Alfalfa (Medicago sativa) to Phosphorus Supply in Two Alkaline Soils.” Plant and Soil 416: 565–584. doi:10.1007/s11104-017-3242-9.
- He, H., E. J. Veneklaas, J. Kuo, and H. Lambers. 2014. “Physiological and Ecological Significance of Biomineralization in Plants.” Trends in Plant Science 19: 166–174. doi:10.1016/j.tplants.2013.11.002.
- Hinsinger, P., C. Plassard, C. X. Tang, and B. Jaillard. 2003. “Origins of Root-mediated pH Changes in the Rhizosphere and Their Responses to Environmental Constraints: A Review.” Plant and Soil 248: 43–59. doi:10.1023/a:1022371130939.
- Kachurina, O. M., H. Zhang, W. R. Raun, and E. G. Krenzer. 2000. “Simultaneous Determination of Soil Aluminum, Ammonium- and Nitrate-nitrogen Using 1 M Potassiumchloride Extraction.” Communications in Soil Science and Plant Analysis 31: 893–903. doi:10.1080/00103620009370485.
- Kereszt, A., D. Li, A. Indrasumunar, C. D. T. Nguyen, S. Nontachaiyapoom, M. Kinkema, and P. M. Gresshoff. 2007. “Agrobacterium Rhizogenes - Mediated Transformation of Soybean to Study Root Biology.” Nature Protocols 2: 948–952. doi:10.1038/nprot.2007.141.
- Kim, K. R., G. Owens, R. Naidu, and S. I. Kwon. 2010. “Influence of Plant Roots on Rhizosphere Soil Solution Composition of Long-term Contaminated Soils.” Geoderma 155: 86–92. doi:10.1016/j.geoderma.2009.11.028.
- Kopittke, P. M., C. J. Asher, R. A. Kopittke, and N. W. Menzies. 2007. “Toxic Effects of Pb2+ on Growth of Cowpea (Vigna unguiculata).” Environmental Pollution 150: 280–287. doi:10.1016/j.envpol.2007.01.011.
- Kushwaha, A., N. Hans, S. Kumar, and R. Rani. 2018. “A Critical Review on Speciation, Mobilization and Toxicity of Lead in Soil Microbe-plant System and Bioremediation Strategies.” Ecotoxicology and Environmental Safety 147: 1035–1045. doi:10.1016/j.ecoenv.2017.09.049.
- Lambers, H., T. L. Pons, and F. S. Chapin. 2008. Plant Physiological Ecology. New York: Springer.
- Lamorski, K., A. Bieganowski, M. Ryzak, A. Sochan, C. Slawinski, and W. Stelmach. 2014. “Assessment of the Usefulness of Particle Size Distribution Measured by Laser Diffraction for Soil Water Retention Modelling.” Journal of Plant Nutrition and Soil Science 177: 803–813. doi:10.1002/jpln.201300594.
- Li, H., Y. Liu, G. Zeng, L. Zhou, X. Wang, Y. Wang, C. Wang, X. Hu, and W. Xu. 2014. “Enhanced Efficiency of Cadmium Removal by Boehmeria nivea (L.) Gaud. In the Presence of Exogenous Citric and Oxalic Acids.” Journal of Environmental Sciences-China 26: 2508–2516. doi:10.1016/j.jes.2014.05.031.
- Li, S., J. Yu, M. Zhu, F. Zhao, and S. Luan. 2012. “Cadmium Impairs Ion Homeostasis by Altering K+ and Ca2+ Channel Activities in Rice Root Hair Cells.” Plant Cell and Environment 35: 1998–2013. doi:10.1111/j.1365-3040.2012.02532.x.
- Little, I. P. 1992. “The Relationship between Soil pH Measurements in Calcium Chloride and Water Suspensions.” Australian Journal of Soil Research 30: 587–592. doi:10.1071/sr9920587.
- Lomonte, C., D. Gregory, A. J. M. Baker, and S. D. Kolev. 2008. “Comparative Study of Hotplate Wet Digestion Methods for the Determination of Mercury in Biosolids.” Chemosphere 72: 1420–1424. doi:10.1016/j.chemosphere.2008.05.033.
- Lopes Júnior, C. A., P. Mazzafera, and M. A. Zezzi Arruda. 2014. “A Comparative Ionomic Approach Focusing on Cadmium Effects in Sunflowers (Helianthus annuus L.).” Environmental and Experimental Botany 107: 180–186. doi:10.1016/j.envexpbot.2014.06.002.
- López, M. L., J. R. Peralta-Videa, T. Benitez, M. Duarte-Gardea, and J. L. Gardea-Torresdey. 2007. “Effects of Lead, EDTA, and IAA on Nutrient Uptake by Alfalfa Plants.” Journal of Plant Nutrition 30: 1247–1261. doi:10.1080/01904160701555143.
- Luan, Z. Q., H. C. Cao, and B. X. Yan. 2008. “Individual and Combined Phytotoxic Effects of Cadmium, Lead and Arsenic on Soybean in Phaeozem.” Plant Soil and Environment 54: 403–411. doi:10.17221/409-PSE.
- Mebius, L. J. 1960. “A Rapid Method for the Determination of Organic Carbon in Soil.” Analytica Chimica Acta 22: 120–124. doi:10.1016/S0003-2670(00)88254-9.
- Moosavi, A. A., S. Mansouri, and M. Zahedifar. 2015. “Effect of Soil Water Stress and Nickel Application on Micronutrient Status of Canola Grown on Two Calcareous Soils.” Plant Production Science 18: 377–387. doi:10.1626/pps.18.377.
- Moosavi, A. A., M. Zahedifar, and S. Mansouri. 2018. “The Uptake and Partitioning of Nickel and Some Nutrient Elements in Canola Grown in Two Differently Textured Soils as Influenced by Nickel and Soil Moisture Conditions.” International Journal of Environmental Studies 77: 30–47. doi:10.1080/00207233.2018.1517969.
- Perfus-Barbeoch, L., N. Leonhardt, A. Vavasseur, and C. Forestier. 2002. “Heavy Metal Toxicity: Cadmium Permeates through Calcium Channels and Disturbs the Plant Water Status.” Plant Journal 32: 539–548. doi:10.1046/j.1365-313X.2002.01442.x.
- Pidatala, V. R., K. Li, D. Sarkar, W. Ramakrishna, and R. Datta. 2016. “Identification of Biochemical Pathways Associated with Lead Tolerance and Detoxification in Chrysopogon zizanioides L. Nash (Vetiver) by Metabolic Profiling.” Environmental Science & Technology 50: 2530–2537. doi:10.1021/acs.est.5b04725.
- Podar, D., M. H. Ramsey, and M. J. Hutchings. 2004. “Effect of Cadmium, Zinc and Substrate Heterogeneity on Yield, Shoot Metal Concentration and Metal Uptake by Brassica juncea: Implications for Human Health Risk Assessment and Phytoremediation.” New Phytologist 163: 313–324. doi:10.1111/j.1469-8137.2004.1122.x.
- Rauser, W. E. 2003. “Phytochelatin-based Complexes Bind Various Amounts of Cadmium in Maize Seedlings Depending on the Time of Exposure, the Concentration of Cadmium and the Tissue.” New Phytologist 158: 269–278. doi:10.1046/j.1469-8137.2003.00735.x.
- Reimann, C., P. Englmaier, K. Fabian, L. Gough, P. Lamothe, and D. Smith. 2015. “Biogeochemical Plant-soil Interaction: Variable Element Composition in Leaves of Four Plant Species Collected along a South-north Transect at the Southern Tip of Norway.” Science of the Total Environment 506: 480–495. doi:10.1016/j.scitotenv.2014.10.079.
- Roelofs, R. F. R., Z. Rengel, G. R. Cawthray, K. W. Dixon, and H. Lambers. 2001. “Exudation of Carboxylates in Australian Proteaceae: Chemical Composition.” Plant Cell and Environment 24: 891–903. doi:10.1046/j.1365-3040.2001.00741.x.
- Salt, D. E., I. Baxter, and B. Lahner. 2008. “Ionomics and the Study of the Plant Ionome.” Annual Review of Plant Biology 59: 709–733. doi:10.1146/annurev.arplant.59.032607.092942.
- Sastre, J., A. Sahuquillo, M. Vidal, and G. Rauret. 2002. “Determination of Cd, Cu, Pb and Zn in Environmental Samples: Microwave-assisted Total Digestion versus Aqua Regia and Nitric Acid Extraction.” Analytica chimica acta 462: 59–72. doi:10.1016/s0003-2670(02)00307-0.
- Sharmila, P., P. K. Kumari, K. Singh, N. V. S. R. K. Prasad, and P. Pardha-Saradhi. 2017. “Cadmium Toxicity-induced Proline Accumulation Is Coupled to Iron Depletion.” Protoplasma 254: 763–770. doi:10.1007/s00709-016-0988-5.
- Sinha, P., B. K. Dube, P. Srivastava, and C. Chatterjee. 2006. “Alteration in Uptake and Translocation of Essential Nutrients in Cabbage by Excess Lead.” Chemosphere 65: 651–656. doi:10.1016/j.chemosphere.2006.01.068.
- Smolders, E., and J. Mertens. 2013. “Cadmium.” In Heavy Metals in Soils-Trace Metals and Metalloids in Soils and Their Bioavailability, edited by B. J. Alloway, 283–311. London: Springer.
- Steinnes, E. 2013. “Lead.” In Heavy Metals in Soils-Trace Metals and Metalloids in Soils and Their Bioavailability, edited by B. J. Alloway, 395–409. London: Springer.
- Weber, M., A. Trampczynska, and S. Clemens. 2006. “Comparative Transcriptome Analysis of Toxic Metal Responses in Arabidopsis thaliana and the Cd2+-hypertolerant Facultative Metallophyte Arabidopsis Halleri.” Plant Cell and Environment 29: 950–963. doi:10.1111/j.1365-3040.2005.01479.x.
- Wenzel, W. W. 2009. “Rhizosphere Processes and Management in Plant-assisted Bioremediation (Phytoremediation) of Soils.” Plant and Soil 321: 385–408. doi:10.1007/s11104-008-9686-1.
- Wu, D., K. Sato, and J. F. Ma. 2015. “Genome-wide Association Mapping of Cadmium Accumulation in Different Organs of Barley.” New Phytologist 208: 817–829. doi:10.1111/nph.13512.
- Wu, H., C. Chen, J. Du, H. Liu, Y. Cui, Y. Zhang, Y. He, et al. 2012. “Co-overexpression FIT with AtbHLH38 or AtbHLH39 in Arabidopsis-Enhanced Cadmium Tolerance via Increased Cadmium Sequestration in Roots and Improved Iron Homeostasis of Shoots.” Plant Physiology 158: 790–800. doi:10.1104/pp.111.190983.
- Wu, J., C. M. Geilfus, B. Pitann, and K. H. Muehling. 2016. “Silicon-enhanced Oxalate Exudation Contributes to Alleviation of Cadmium Toxicity in Wheat.” Environmental and Experimental Botany 131: 10–18. doi:10.1016/j.envexpbot.2016.06.012.
- Xu, S. S., S. Z. Lin, and Z. X. Lai. 2015. “Cadmium Impairs Iron Homeostasis in Arabidopsis thaliana by Increasing the Polysaccharide Contents and the Iron-binding Capacity of Root Cell Walls.” Plant and Soil 392: 71–85. doi:10.1007/s11104-015-2443-3.
- Yoshihara, T., H. Hodoshima, Y. Miyano, K. Shoji, H. Shimada, and F. Goto. 2006. “Cadmium Inducible Fe Deficiency Responses Observed from Macro and Molecular Views in Tobacco Plants.” Plant Cell Reports 25: 365–373. doi:10.1007/s00299-005-0092-3.
- Zahedifar, M., A. A. Moosavi, M. Shafigh, Z. Zarei, and F. Karimian. 2016. “Cadmium Accumulation and Partitioning in Ocimum basilicum as Influenced by Application of Various Potassium Fertilizers.” Archives of Agronomy and Soil Science 62: 663–673. doi:10.1080/03650340.2015.1074187.
- Zhen, Q., W. Ma, M. Li, H. He, X. Zhang, and Y. Wang. 2015. “Effects of Vegetation and Physicochemical Properties on Solute Transport in Reclaimed Soil at an Opencast Coalmine Site on the Loess Plateau, China.” Catena 133: 403–411. doi:10.1016/j.catena.2015.06.009.
- Zhu, X. F., C. Zheng, Y. T. Hu, T. Jiang, Y. Liu, N. Y. Dong, J. L. Yang, and S. J. Zheng. 2011. “Cadmium-induced Oxalate Secretion from Root Apex Is Associated with Cadmium Exclusion and Resistance in Lycopersicon esulentum.” Plant Cell and Environment 34: 1055–1064. doi:10.1111/j.1365-3040.2011.02304.x.