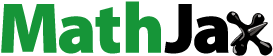
ABSTRACT
Understanding how plants use of various nitrogen (N) sources is important for improving plant N use efficiency in organic farming systems. This study investigated the effects of farming management practices (organic and conventional) on pakchoi short-term uptake of glycine (Gly), nitrate (NO3−) and ammonium (NH4+) under two N level conditions. Results showed that plant N uptake rates and N contributions from the three N forms in the low N (0.15 μg N g−1 dry soil) treatment did not significantly differ between the organic and conventional soils, except the significantly greater Gly contribution in organic soil at 24 h after tracer addition. Under high N (15 μg N g−1 dry soil) conditions, the N uptake rates, uptake efficiencies, and N contributions of Gly and NH4+-N were significantly greater in pakchoi cultivated in the organic soil compared to conventional soil, whereas the N uptake rates and N contributions from NO3–-N decreased in pakchoi cultivated in the organic soil. The greater Gly-N uptake in plants grown in high-N treated organic soil may be related to the greater gross N transformation, Gly turnover rate and the increased expression of an amino acid transporter gene BcLHT1. Intact Gly contributed at most 6% to Gly-derived N at 24 h after tracer additions, which accounting for about 1.24% of the total N uptake in organic soil. Our study suggested that Gly-N and other organic source N might serve as a more important compensatory N source for plants in organic farming.
1. Introduction
Nitrogen (N), particularly nitrate (NO3−) is one of the most important inorganic nutrients that is essential for sustaining plant growth. It is known that plants can adjust their use of different N forms to cope with environmental changes. For example, plants can reduce competition for N with soil microbes and coexisting plants by taking up different forms of N (Harrison, Bol, and Bardgett Citation2008). Other studies have shown that plants adjust their usage of different forms of N according to changes in soil N supply (Warren Citation2009), light intensity (Xu et al. Citation2008), crude oil contamination (Nie et al. Citation2011) and agronomic measures (Jiang et al. Citation2016). In particular, changes in agricultural practices (i.e., organic farming vs. conventional farming) are known to greatly alter the soil N cycle. Consumer concerns regarding the impacts of conventional agriculture on the environment and human health have spurred the growth of organic farming systems. Unlike high-input, chemical intensive agricultural systems, organic agriculture relies primarily on green manures and/or composts and animal manures to meet crop N demands. However, to our knowledge, little information is available about the effects of organic management on the competition for organic and inorganic N by plants in the soil environment.
Although organic management has been recommended for improving soil physical properties (Papadopoulos et al. Citation2014) and microbial diversity (Reeve et al. Citation2010; Orr et al. Citation2011, Citation2012; Ge et al. Citation2013; Perez et al. Citation2014; Khalil et al. Citation2015), organic agriculture is often criticized for being low yielding in comparison to conventional systems because of low inorganic N levels available to the plants (Rippy et al. Citation2004). Researchers have presumed that improving the plant’s ability to uptake organic N may be beneficial for improving plant N use efficiency, considering that organic N predominates in organic systems (Murphy et al. Citation2007; Baresel, Zimmermann, and Reents Citation2008; Reeve et al. Citation2009; Reid et al. Citation2009; Van Bueren et al. Citation2011). However, the competition uptake of organic N molecules and its contribution to plant growth has rarely been studied in organic farming (Dion et al. Citation2018).
To understand the issue mentioned above, we selected pakchoi (Brassica campestris L. ssp. chinensis Makino var. communis Tsen et Lee) as our study species because it is an important leaf vegetable in China. This vegetable is widely cultivated in both conventional and organic management systems and is consumed during most of the year. Our previous studies showed that soluble organic nitrogen (SON) was the more abundant N source in the organic system for pakchoi production compared to that in the conventional system (Wang et al. Citation2013), and pakchoi glycine (Gly) uptake efficiency in organic soil was significantly higher than that in conventional soil (Wang et al. Citation2015). Thus, we think that there might be significant differences in pakchoi N preference between conventional and organic soils. Understanding how pakchoi adjust their use of various N sources is important for improving crop N use efficiency and crops breeding in organic farming. Moreover, knowledge of total N concentrations on the uptake of organic N in organic farming and assessment of the expression of root amino acid transporter genes in response to soil environment changes is still missing. In this controlled study, we conducted experiments using pakchoi to examine how soil management affects plant uptake of inorganic N (nitrate: NO3–N; ammonium: NH4+-N) and Gly under different N regimes, and interpret these results in the context of data from soil N turnover and the patterns of expression of transporter genes in pakchoi.
2. Materials and methods
2.1. Site description and sampling
Organic soil samples were obtained from the Chongbentang Organic Vegetable Farm, Shanghai, China (30°57′ N, 121°21′E). This farm has been organically cultivated for more than 6 years. The conventional site is located close to the organic plots and has been conventionally cultivated for more than 20 years. The climate is humid subtropical, and 70% of the annual precipitation (1255 mm) occurs between May and September. The mean annual air temperature is 17.5°C, and the average total annual sunshine is 1778 h. The soil is a Fuvisol developing toward a Cambisol and contains approximately 8% sand, 71% silt, and 21% clay to a soil depth of 40 cm.
The organic field was fertilized with a pre-plant–incorporated organic fertilizer [Lijiang®, a commercial product; 1.5% N, 0.6% P, 3% K; organic matter >30%] at an application rate of 80 t ha−1 y−1. The conventional field received NPK compound fertilizer (N: P: K = 14:10:12) and urea at rates of 1.1 t ha−1 y−1 and 0.2 t ha−1 y−1, respectively. A diverse selection of vegetables is usually planted within one consecutive year in both the organic and conventional fields. The main crops cultivated were cabbage (Brassica oleracea L.), pakchoi (var. communis Tsen et Lee and var. rosularis Tsen et Lee), and onion (Allium fistulosum L.). Plants were irrigated using overhead sprinklers. Weeds were controlled manually in the organic fields and chemically in the conventional fields. Pesticides were not used in the organic system because no significant insect damage occurred. Synthetic pesticides were generally applied in the conventional plots based on the farmers’ experience. Soil from three separate fields (approximately 225 m2 each) was sampled at a depth of 0–20 cm from both the organic and conventional systems during the pakchoi harvest period. The soil samples were sieved (2 mm mesh), cleared of plant matter and earthworms, homogenized, and used for soil property analyses and pot experiments (Wang et al. Citation2015). Selected properties of the soils are shown in .
Table 1. Selected soil properties of two different management systems.
2.2. Soil chemical and biological analyses
Soil pH and electrical conductivity (EC) were determined in 1:1 (v/v) soil: deionized water extracts using standard electrodes. Total carbon (C) and total N were determined using a Vario ELIII elemental analyzer (Elementar Analysensysteme GmbH, Germany). The concentrations of total soluble nitrogen (TSN), NO3–N, NH4+-N, and SON were determined in 1:5 (v/v) soil: 2 M KCl extracts. TSN was measured using the alkaline persulfate oxidation method (Muruganandam, Israel, and Robarge Citation2009), while soluble inorganic N (NO3–N and NH4+-N) was determined using an automated flow injection analyzer (Smartchem 200, WestCO Scientific Instruments Inc., Italy). SON was calculated as the difference between TSN and soluble inorganic N. Free amino acids (FAA) were extracted with distilled water (20 g fresh weight [FW] soil: 100 mL distilled water) by shaking the soil suspension for 6 h at 4°C and centrifuging at 12,000 rpm for 10 min at 4°C. The supernatant was filtered, collected, freeze-dried, and suspended in 0.02 M hydrochloric acid (HCl) before being analyzed with an L-8900 high-speed amino acid analyzer (Hitachi High Technologies Corporation, Japan). The total FAA concentrations were calculated as the sum of all amino acids measured (histidine, lysine, leucine, phenylalanine, tyrosine, glycine, serine, threonine, aspartic acid, and glutamic acid). Soil microbial biomass C and N were measured using a fumigation and extraction technique (Witt et al. Citation2000).
2.3. Plant culture
The method of seedling cultivation was identical to that employed by Wang et al. (Citation2015). Briefly, three pakchoi (cv. ‘Huawang’) seedlings were transplanted into 250 mL pots filled with 154 g of dry soil. After 14 days of growth, the plants were transferred to a growth chamber (a 14 h daytime period with 800 µmo lm−2 s−1 light intensity; daytime and nighttime temperatures were 25°C and 20°C, respectively) for an additional 1 day of growth in order to acclimate plants to the growth chamber environment and to perform the labeling experiments under strictly controlled conditions. Watering was terminated 3 days before labeling in order to slightly dry the soil and facilitate application of the treatment solutions.
2.4. 15N uptake
The 15N tracer addition consisted of a combination of an identical amount of the three N forms (NH4+-N, NO3–N, and Gly-N) with only one form 15N-labeled and the remaining two forms unlabeled for each treatment. Gly was dual labeled with 13C (1–13C or 2–13C) and 15N to predict the intact Gly uptake by the 13C/15N ratios. The 15N compounds were 15NH4Cl (99% of atom), K15NO3 (99% of atom), 1–13C, 15N-glycine, and 2–13C, 15N-glycine (15N, 99% of atoms; 13C, 99% of atoms) (Cambridge Isotope Laboratories, Inc., MA, USA). A control treatment contained all three N compounds with the natural abundance of their N isotopes (Sigma-Aldrich). The N amount added to each pot was 0.05 (low N treatment) or 5 μg N g−1 dry soil (high N treatment) for each N form (together, a full treatment was 0.15 or 15 μg N g−1 dry soil) (). The low N concentration (0.05 μg N g−1 soil) represented the minimum application amount needed for substance-specific measurements. The concentration was defined a priori by means of the precision of the measurement and the size of the standard error in the field replicates, which should be small enough to enable detection of any significant differences between the labeled and background samples. The high N concentration (15 μg N g−1 soil) represents the maximum amount of Gly in the same sampled soil and is consistent with our previous result (Wang et al. Citation2013).
Figure 1. Experimental design of labeling different 15N forms in relation to N concentration treatments, farm management treatments, and time treatments.
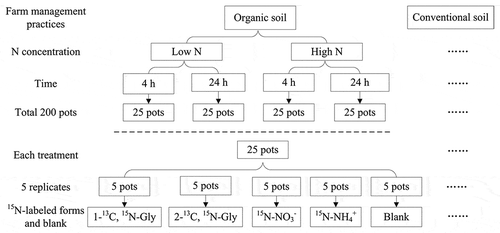
The nutrient solutions (30 mL) were spread onto the surface of the soil with a pipette. Any solution that leaked out of the bottom of the pot (maximum 1–2 mL) was collected in a Petri dish and spread again onto the soil surface. The relatively high volume of the applied solution in comparison to the dry weight of the soil and the fast occurrence of leakage out of the bottom of the pots should assure relatively fast distribution of the label within the system. Five replicates (five pots with three plants each) were used for each treatment and the control. A total of 200 pots (2 added N concentrations × 2 soil management types × 5 15N-labeled forms (1 non-labeled pot as a control) × 2 treatment times × 5 replicates) for each treatment were randomly placed in the growth chamber ().
All samples were harvested at 4 and 24 h after N application. The 4 and 24 h sampling times were chosen because of the fast kinetics of amino acid turnover in the soil and the quick post-uptake metabolism in the plants. After the shoots had been removed, the plant roots were manually separated from the soil and rinsed in 0.01 M CaCl2 before they were washed with deionized water. The plant shoots and roots were freeze-dried, weighed to determine their dry weight, and then milled into a fine powder using a Precellys 24 homogenizer (Bertin Technologies, Orléans, France). The total contents of N, 15N, C, and 13C in the plant tissues were determined using a Vario-EL III IRMS (Elementar Analysensysteme GmbH, Hanau, Germany). The δ15N and δ13C of the reference were calibrated against atmospheric N2 and Peedee Belemnite, respectively.
2.5. Gross nitrogen transformation, soil respiration rate, and Gly turnover rate
For the determination of the soil respiration rate (SRR), gross mineralization rate (GMR) and gross nitrification rate (GNR), root-free soil samples were collected from non-labeled pots (blanks) as soon as 15N tracer was added to the labeled pots. The SRR was measured based on alkali absorption of CO2 at 28°C for 24 h, followed by titration of the residual OH− with a standardized HCl solution (Yim, Joo, and Nakane Citation2002). Gly mineralization estimated as half-life was determined by adding a 15N labeled Gly (5 μg N g−1 dry soil) to soil and their subsequent absorption in micrograms after 24 h incubation as described in Henry and Jefferies (Citation2003). Gross N mineralization and nitrification rates were measured using a 15N pool dilution technique (Cookson et al. Citation2006). Briefly, at the same time as the 15N-labeling uptake experiment, 1 ml of 15NH4+-N or 15NO3–N labeled solution was thoroughly mixed with a sub-sample of root-free soil that was collected from the non-labeled pots. The 15N tracer addition consisted of two N forms (NH4+ and NO3−) in combination (1 μg N g−1 dry soil for each N form), with only one 15N-labeled form (15NH4Cl, 99% of atoms, or K15NO3, 99% of atoms) and the other unlabeled. After a 4 or 28 h incubation period, sub-samples of the soils were immediately extracted with 0.5 M K2SO4 (1:4 soil to solution ratio), and NH4+ and NO3− concentrations of the extracts were determined. The 15N content of NH4+ and NO3− in the K2SO4 extracts was measured separately using a two-stage diffusion method (Brooks et al., Citation1989), and the GMR and GNR were calculated using the following EquationEquation (1)(1)
(1) as described by Kirkham and Bartholomew (Citation1955).
In the above formula, m is GMR or GNR (μg N g−1 d−1); M0 the initial total N pool (μg N g−1); M is the post-incubation total N pool; t is the length of the incubation in days; H0 is the initial 15N pool (μg N g−1); and H is the post-incubation 15N pool (μg N g−1).
2.6. Quantitative real-time PCR
Though many amino acid transporters have been functionally characterized recently, a direct role in root organic N uptake has only been demonstrated for two amino acid transporters, Arabidopsis amino acid permease 1 (AtAAP1) and lysine histidine transporter 1 (AtLHT1) (Hirner et al. Citation2006; Lee et al. Citation2007). For nitrate uptake, Arabidopsis nitrate transporter 1.1 (AtNRT1.1) and Brassica nitrate transporter 2.1 (BnNRT2.1) function in dual-affinity and high-affinity nitrate uptake from soil to root, respectively (Liu et al. Citation2014; Sun and Zheng Citation2015). To demonstrate the possible role of these transporters in pakchoi N uptake, a quantitative real-time PCR (qRT-PCR) assay was performed on pakchoi root after 24 h N treatments to determine their relative expression.
Total RNA was extracted using TRIzol reagent (Invitrogen, Carlsbad, CA, USA) according to the manufacturer’s instructions, and was reverse transcribed using the PrimeScript™ RT Master Mix kits (TAKARA, Tokyo, Japan). The qRT-PCR was performed using a SYBR® Premix Ex Taq™ II kit (TAKARA, Tokyo, Japan) on an ABI 7500 Real-Time PCR System (Applied Biosystems, USA). The primer pairs used were listed in Table S1. The relative quantitation of gene expression was measured via the 2−ΔCt method, with GAPDH used as the endogenous reference gene.
2.7. Calculations and statistics
15N calculations were based on percent atom excess, calculated as the difference between 15N-treated and control plants as described by Näsholm, Huss-Danell, and Högberg (Citation2000). The N uptake in plants was corrected for dilution of the added 15N in the soil Gly-N, NH4+-N, and NO3–-N pool to approximate the true N uptake of the N forms. The uptake rate of Gly-N, NH4+-N, or NO3–-N over the treatment period was calculated using EquationEquations (2)(2)
(2) and (Equation3
(3)
(3) ).
In the above formula, Nuptake is the amount of a given N source (Gly-N, NH4+-N, or NO3–-N) taken up into the whole pakchoi seedling, 15Nplant is the 15N amount taken up by the plant tissue, and Ntotal in soil/15Nsoil is the ratio of total soil N amount (the sum of the initial total N content in soil before 15N addition and N input in soil through 15N addition) to the 15N amount of a given N source (from Gly-N, NH4+-N, or NO3–-N) after 15N addition.
N uptake efficiency (EquationEquation 4(4)
(4) ) is the proportion of Gly-N, NH4+-N, or NO3–-N taken up by the whole pakchoi seedling based on the total soil N amount of a given N source after 15N addition. Nsoil is the total amount of Gly-N, NH4+-N, or NO3–-N in soil after initiation of the assay. Ncontribution is the proportion of total N taken up as Gly-N, NH4+-N, or NO3–-N by whole pakchoi seedlings, and Ntotal in plants is the total N amount in the pakchoi (EquationEquation 5
(5)
(5) ).
No correction was made to account for N transformation processes (e.g., microbial competition, Gly ammonification, and mineralization) after initiation of the 15N labeling experiment, so the calculated uptakes and uptake efficiencies may overestimate N uptake at ambient concentrations. Despite this potential overestimation, the total 15N excess in soil after initiation of the assay did not significantly differ between the two types of soil within the concentrations (Table S2). Thus, we assumed that the potential enrichment and dilution of the N pools did not affect the comparison of the N uptake rates for the two types of soil, and that the total N uptake rates could represent the actual N uptake of the plants in each treatment.
The experiments were set up as a randomized block design. One-way ANOVA was used to identify significant differences in soil properties of soil from different farm management systems. Multiple factor ANOVA was performed to test the effects of N form, added N concentration, soil management type, treatment time, and their interactions on N uptake, plant biomass, total N content, and gene expression. All results were considered significant at the P<= 0.05 level using Tukey’s HSD tests with fixed factor models. Statistical calculations were performed using SPSS software version 13.0 (SPSS Inc., USA).
3. Results
3.1. Plant biomass and total N content
Pakchoi dry mass and total N content displayed different patterns in response to farm management and N concentration treatments after 24 h in the isotopic labeling experiment (). The shoot dry mass in the organic soil was significantly higher than that in the conventional soil (P< 0.05), while no differences were found between high and low N treatments. The dry mass of the roots did not differ among any of the treatments. The high N treatment significantly increased the total N content in the shoots and in conventionally cultivated roots. No significant differences in total N content were observed between the organic and conventional treatments, except for a significantly greater total N content in conventionally cultivated roots compared to organically cultivated roots (P< 0.05).
Figure 2. Biomass (a) and total N contents (b) in shoots and roots of pakchoi seedlings under different farm management types and N concentration treatments. Different lower-case letters indicate significant differences between farm management and nitrogen level treatments for a given plant tissue. Values are mean ± SE.
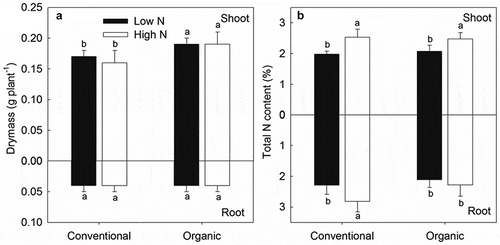
3.2. N turnover in the soil
The gross N transformation, soil respiration rates, and Gly turnover rates are shown in . In conventional soil, the GMR, GNR, and SRR in the high N treatments were approximately 1.50-, 1.83, and 1.32- folds higher than that under low N conditions, respectively. The GMR, GNR, and SRR of organic soil in the high N treatments were 1.8-, 1.3- and 1.4-folds greater than those in the low N treatment, respectively. The GMR, GNR, and SRR in organic soil were generally greater than those in conventional soil, except for the SRR under low N conditions and GNR under high N conditions; these differences were not statistically significant between organic and conventional soil. Turnover of 15N-Gly was slowest in low N-treated conventional soil (half-life was 3.89 h), fastest in high N-treated organic soils (half-life was 1.74 h), but did not differ significantly between high N-treated conventional soil and low N-treated organic soil (aver. half-lives were 2.76 h).
3.3. N uptake rate and N uptake efficiency
Multiple factor analyses of variance indicated significant effects of soil management type, treatment time, N concentration, and N form, as well as their interactions, on N uptake rate and N uptake efficiency by pakchoi seedlings (P< 0.05, ).
Table 2. Results of multiple factor ANOVA for the effects of farm management type, N concentration, treatment time, and N form on N uptake rates (mg N g−1 dry mass h−1), N uptake efficiencies (%), and N contribution (%) of different N forms by pakchoi.
As shown in , the uptake rates of the three N forms by pakchoi under low N conditions showed no significant difference between organic and conventional soil during the whole experimental period. While under high N treatments, the uptake rates of Gly-N and NH4+-N were significantly higher (aver. 1.61- and 1.79-folds, respectively) in the organic soil than those in the conventional soil, in contrast to the uptake rate of NO3–-N, which was lower in conventional soil. Irrespective of farm management type, the N uptake rates of the three N forms were clearly increased with increasing concentrations of added N for all treatments and generally decreased with a longer treatment time (except for Gly under the low N treatment). Moreover, the uptake rates of NO3–-N (aver. 1.16 mg N g−1 h−1) were greater than those of Gly-N (aver. 0.14 mg N g−1 h−1) or NH4+-N (aver. 0.20 mg N g−1 h−1) for all treatments.
Figure 4. The N uptake rates (mg N g−1 dry mass h−1) in whole plant of pakchoi at 4 h under low N treatment (a), 24 h under low N treatment (b), 4 h under high N treatment (c), and at 24 h under high N treatment (d). Different lower-case letters indicate significant differences between all treatments at 0.05 level. Values are mean ± SE.
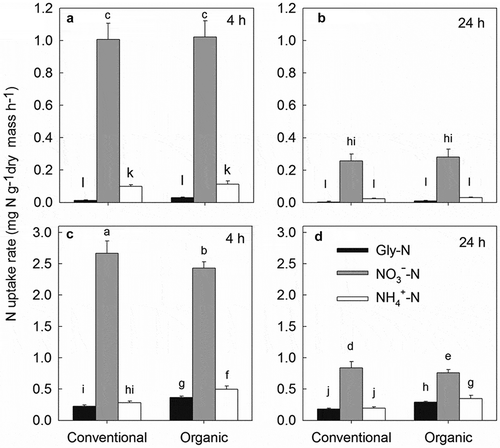
The uptake efficiencies of the three N forms under low N conditions were all greater in the organic soil than in the conventional soil at both 4 h and 24 h after trace addition (). Under high N conditions, the N uptake efficiencies of Gly-N and NH4+-N were generally greater in the organic soil, being 2.50 and 1.70 times higher than those in the conventional soil, respectively. However, the NO3–-N uptake efficiencies did not significantly differ between the organic and conventional soil during the whole experimental period. Moreover, although the mean value of the uptake efficiency of Gly was lower than NO3–-N and NH4+-N (), the uptake efficiency of Gly was greatly enhanced by organic management compared to those of NO3–-N, being 1.92 (4 h)-3.39 (24 h) and 1.60 (4 h)-1.59 (24 h) times higher in the organic than in the conventional soil under low and high N conditions, respectively. The plant N uptake efficiencies generally increased with increasing added N concentrations and prolonged treatment time for all treatments, except for Gly uptake efficiency in the conventional soil.
Figure 5. N uptake efficiencies of different N forms in whole plant of pakchoi at 4 h under low N treatment (a), 24 h under low N treatment (b), 4 h under high N treatment (c) and at 24 h under high N treatment (d). Different lower-case letters indicate significant differences between all treatments at 0.05 level. Values are mean ± SE.
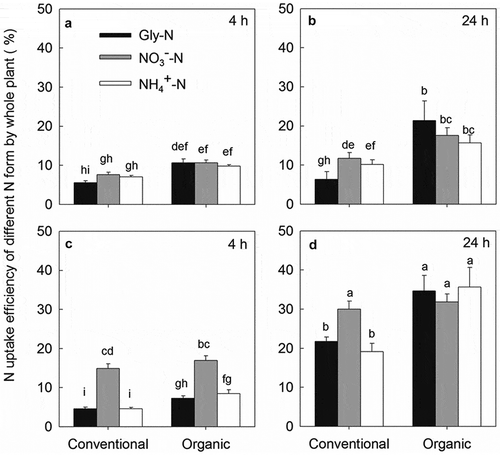
The N contributions of the three N forms were also significantly affected by farm management and applied N concentrations, as well as their interactions ( and ). During the whole experimental period, the N contributions of Gly-N and NH4+-N under low N conditions showed an increasing trend in the organic soil, though only the N contribution of Gly-N significantly increased in the organic soil at 24 h after tracer addition, with a 7.8-fold increase compared to the conventional soil. The high N treatment decreased the N contribution of NO3–-N at 4 h but increased N contributions of Gly-N and NH4+-N at 24 h in the organic soil. Correspondingly, the percent N contributions of Gly-N and NH4+-N were significantly higher in the organic soil (20.71% and 25.00%, respectively) compared to the conventional soil (11.95% and 17.82%, respectively), whereas the reverse was observed for NO3–-N (70.23% in the conventional and 54.29% in the organic soil; Figure S1). For all treatments, the N contributions of NO3–-N (2.62–12.77%) to the plants were far greater than those of Gly-N (0.04–4.87%) and NH4+-N (0.28–5.88%).
3.4. 13C/15N ratios in plants
The values of 13C/15N ratios in the plants were used to predict the proportion of assimilated organic N as an intact amino acid. As shown in , different N concentrations and farm management practices had no clear effects on the 13C/15N ratio for each C position of Gly. The 13C/15N ratio of the C-1 position was significantly lower than that of the C-2 position. The average 13C/15N ratio in pakchoi among all treatments was 0.06 and 0.19 for the C-1 and C-2 positions, respectively.
Table 3. Ratios of 13C(μmol excess 13C g−1)/15N (μmol excess 15N g−1) of different Gly C positions in pakchoi plants.
3.5. Gene expression analysis
As shown in , the high N treatment generally increased the transcript levels of root BcAPP1, BcLHT1, and BcNRT1.1 of plants grown in both conventional and organic soil, but decreased the root BcNRT2.1 mRNA abundances in the plants grown in conventional soil. Organic management generally increased the levels of BcAPP1 transcripts in low N-treated pakchoi roots and the transcripts of BcLHT1 and BcNRT2.1 in high N-treated roots. The great abundance of BcLHT1 transcripts in plants grown in high N-treated organic soil was about five times higher than those grown in high N-treated conventional soil.
4. Discussion
Pakchoi is well known for its preference for NO3–-N (Wang, Tang, and Huang Citation2014), so it is not surprising that NO3–-N was preferred by pakchoi in all treatments, with the highest N uptake rates and greater N contributions from NO3–-N compared to the other two N sources. In spite of this, the pakchoi uptake of Gly-N at 4 h (0.002–0.37 mg N g−1 h−1) was comparable to the uptake rates published for other species, such as 0.03–0.06 mg N g−1 h−1 in maize (Xu et al. Citation2008), 0.03–1.35 mg N g−1 h−1 in Cyperaceae (Raab, Lipson, and Monson Citation1999), and 0.02 mg N g−1 h−1 in barley (Jamtgard, Näsholm, and Huss-Danell Citation2008). This finding clearly indicates that pakchoi is able to access organic N even in an inorganic N enriched soil environment. This is in agreement with previous studies on plant uptake of amino acids in the presence of inorganic N (Ge et al. Citation2009; Gioseffi, de Neergaard, and Schjoerring Citation2012; Dion et al. Citation2018). Moreover, pakchoi Gly-N uptake was very similar to NH4+ uptake, especially in high N conditions. According to the findings by Jones et al. (Citation2004), applied amino acids are quickly either immobilized by microbes or fixed by the soil matrix. The similarity between plant uptake of NH4+-N and Gly-N may be explained by a rapid decomposition of Gly by microorganisms to inorganic N, thereafter affecting the uptake of N from Gly in the form of NH4+. It is worth noting that, the similarity of uptake rates between NH4+-N and Gly-N by pakchoi was also found in a sterile hydroponics experiment with a similar total N concentration of 250 μmol L−1 (approximately equivalent to 3.89 g N kg−1 dry soil, based on the hypothesis that soil water content was 10% and soil bulk density was 1.0 g cm−3; Cao et al. Citation2015). This seems to suggest that pakchoi has an inherently similar ability to uptake Gly-N with NH4+-N.
Consistent with our hypothesis, the plant N use was different between organically and conventionally managed soils. Organic management generally led to a significant increase in N uptake (except the uptake rate of NO3–-N) and N uptake efficiency for all three N forms compared to the conventional soil (). Increasing the concentration of the N supply generally led to a significant increase in N uptake from the three N forms because plant N uptake is concentration dependent (Thornton and Robinson Citation2005; Warren Citation2009; Jones et al. Citation2005; Sauheitl, Glaser, and Weigelt Citation2009). More importantly, we observed a significant interaction between farm management and soil N concentration on pakchoi N uptake. Briefly, the uptake rates and N contributions of Gly-N and NH4+-N were clearly enhanced in the high N-treated organic soil, whereas under low N conditions, they did not significantly differ between the two types of soils (except the Gly contribution at 24 h after tracer addition; and ). This indicates the importance of the levels of applied N in plant N uptake in response to farm management system changes. Previous studies have found that the pakchoi glycine uptake rate increased with increasing applied N concentrations (Wang et al. Citation2015) and that plants became more competitive for amino acids at high amino acid concentrations (>1 mmol L−1) where the capacity of soil microbes to take up amino acids is saturated (Vinolas et al. Citation2001). However, in this study, there was no significant difference in the total concentrations of Gly-N and NH4+-N between the organically and conventionally managed soil after the initial 15 μg N g−1 N addition (Table S3), and the Gly concentration in this study was far lower than 1 mmol L−1 (approximately equivalent to 15.56 g N kg−1 dry soil). This highlights that the increased uptake of Gly-N that we observed for pakchoi grown in the organic soil was not solely a consequence of the relatively high Gly concentration. The reason for the higher Gly uptake was probably due to relatively high N turnover rate and microbial activity in high-N treated organic soil. It has been reported that soil N-cycling microbial activity (Perez et al. Citation2014), soil respiration, microbial biomass (Ge et al. Citation2011) and mineralization rate of amino acids (Ge et al. Citation2010) were higher under organic management systems, indicating that organic farming can influence the dynamics, availability, and balance of soil N. Similarity, this study also found a greater GMR, GNR, SSR, and Gly turnover rate in organic horticultural soil, especially in high N-treated organic soil. The increased microbial activities which related to decomposition and ammonification in organic soil seem to suggest that the majority of added 15N-Gly might be mineralized in the organic soil, and that was mainly taken up as inorganic N, which is more easily available for plants. Another possibility is that the internal selective absorption of N forms by the plant might be changed in response to different soil environments. Membrane-bound transporters are required for active uptake of N from the soil. In pakchoi, a putative amino acid transporter encoding gene BcLHT1 showed significantly enhanced expression in high N-treated organic soil. This indicated that enhanced levels of BcLHT1 may be somehow related to greater Gly uptake. However, we did not find a similar correlation between the Gly uptake and BcAPP1 expression, suggesting BcAPP1 may fulfill different physiological functions with its Arabidopsis homologs. This is not surprising because functional divergence of different members of the amino acid/auxin permeases protein family (including AAP subfamily and LHT subfamily) across plant species has been reported (Hu et al. Citation2014). The candidate genes/proteins for pakchoi amino acid uptake still need to be identified by large-scale omics analyses and verified by functional analyses.In contrast to Gly-N and NH4+-N, the uptake and Ncontribution of NO3–-N decreased in the organic soil under high Nconditions. This finding may be due to the relatively low concentration of NO3–-N or the relatively low ratio of inorganic N: organic Nin the high N-treated organic soil (Table S3). Another explanation is that the uptake of one form of Nmay affect the uptake of the other forms. Previous studies have reported that the addition of Gly decreased the uptake of NH4+-N and NO3–-N in amixed solution compared with asingle nutrient solution (Thornton and Robinson Citation2005) and that NH4+-N can inhibit the uptake of NO3–-N (Miller and Bowman Citation2003). Gioseffi, de Neergaard, and Schjoerring (Citation2012) also found nitrate uptake was decreased when both Gly and nitrate were present, compared to nitrate alone at the same Nconcentration. However, in this study, the expression of two nitrate transporter genes NRT1.1 and NRT2.1 was not down-regulated in high N-treated organic soil. Their relatively high expression may contribute to the uptake of mineralized organic N, wherein greater organic Nwas mineralized to inorganic Nin high N-treated organic soil. Considering the complexity and variation of gene function between NRT members, the putative function of these homologs in pakchoi Nabsorption needs to be further investigated.
The application of 13C and 15N-Gly allowed a rough estimation of intact Gly-to-Gly-derived 15N acquisition by pakchoi based on the 13C to 15N ratios. Using position-specific labeling, we found relatively higher 13C/15N ratios with the C-2 position (0.19) compared to the C-1 position (0.06). The preferential oxidation of C1 and preferential incorporation of C2 by microorganisms is likely the cause of the loss of C-1 and the accumulation of C-2 in plant-soil system (Moranzuloaga et al. Citation2015). Considering that a molecule of Gly could only be taken up intact when all C positions were incorporated into the plant, the minimum 13C/15N ratio (0.06) reflects the maximum intact uptake of Gly (Moranzuloaga et al. Citation2015). Based on this value, at most, 6% of Gly-N was taken up as intact Gly in pakchoi. Therefore, our finding suggests that the Gly is mainly taken up in the form of molecule fragments. It should be noted that we did not consider the absorption of mineralized 13CO2 by plants and the loss of Gly-derived 13C due to respiration; thus, the intact uptake value calculated here may be roughly estimated. Combined the use of isotopic labeling with compound-specific isotope analysis will be needed for further study. According to the intact uptake ratio, the intact Gly-N may contribute to about 1.24% (multiplying the intact uptake ratio [6%] by the maximum percentage N contribution of Gly-derived N in the organic soil at the 24 h treatment [20.71%]) to the total plant N nutrition in the organic soil. A low contribution of amino acid-N was also found in cucumber grown in greenhouse organic horticulture, with 0.94%-6.84% of the total N was taken up from Ala (Dion et al. Citation2018). Considering that there are probably more than 20 other small organic N compounds being taken up at a similar rate in soil, the total uptake of organic N may be much larger than for Gly on its own (Thornton and Robinson Citation2005).
5. Conclusions
This study highlights the fact that a plant has great flexibility to respond to land use changes, and pakchoi tends to increase N uptake from glycine in organic farming, particularly when available N is enriched, although organic N uptake appears to have only a marginal role in the total crop N budget. A more efficient usage of N sources through agronomic measures and plant breeding, may be helpful in meeting plant N demand and improving plant N use efficiency in organic farming. Irrespective of their uptake mechanisms, the importance of N supply from Gly-N and other organic source N in organic farming should not be neglected.
Supplemental Material
Download Zip (202.8 KB)Disclosure statement
No potential conflict of interest was reporter by the authors.
Supplementary material
Supplemental data for this article can be accessed here.
Additional information
Funding
References
- Baresel, J. P., G. Zimmermann, and H. J. Reents. 2008. “Effects of Genotype and Environment on N Uptake and N Partition in Organically Grown Winter Wheat (Triticum Aestivum L.) In Germany.” Euphytica 163 (3): 347–354. doi:10.1007/s10681-008-9718-1.
- Brooks, P. D., J. M. Stark, B. B. Mcinteer, and T. Preston. 1989. “A Diffusion Method to Prepare Soil Extracts for Automated Nitrogen-15 Analysis.” Soil Science Society of America Journal 53: 1707–1711. doi:10.2136/sssaj1989.03615995005300060016x.
- Cao, X. C., L. Wu, L. Yuan, X. Li, Y. Zhu, and Q. Jin. 2015. “Uptake and Uptake Kinetics of Nitrate, Ammonium and Glycine by Pakchoi Seedlings (Brassica Campestris L. Ssp. Chinensis L. Makino).” Scientia Horticulturae 186: 247–253. doi:10.1016/j.scienta.2015.02.010.
- Cookson, W., C. Muller, P. A. Obrien, D. V. Murphy, and P. F. Grierson. 2006. “Nitrogen Dynamics in an Australian Semiarid Grassland Soil.” Ecology 87: 2047–2057. doi:10.1890/0012-9658(2006)87[2047:NDIAAS]2.0.CO;2.
- Dion, P. P., S. Jamtgard, A. Bertrand, S. Pepin, and M. Dorais. 2018. “Organic Nitrogen Uptake and Assimilation in Cucumis Sativus Using Position-specific Labeling and Compound-specific Isotope Analysis.” Frontiers in Plant Science 9: e1596. doi:10.3389/fpls.2018.01596.
- Ge, T., X. Chen, H. Yuan, B. Li, H. Zhu, P. Peng, K. L. Li, D. L. Jones, and J. Wu. 2013. “Microbial Biomass, Activity, and Community Structure in Horticultural Soils under Conventional and Organic Management Strategies.” European Journal of Soil Biology 58: 122–128. doi:10.1016/j.ejsobi.2013.07.005.
- Ge, T. D., D. Huang, P. Roberts, D. L. Jones, and S. Song. 2010. “Dynamics of Nitrogen Speciation in Horticultural Soils in Suburbs of Shanghai, China.” Pedosphere 20: 261–272. doi:10.1016/S1002-0160(10)60014-8.
- Ge, T. D., S. Nie, J. Wu, J. Shen, H. Xiao, C. Tong, D. Huang, Y. Hong, and K. Iwasaki. 2011. “Chemical Properties, Microbial Biomass, and Activity Differ between Soils of Organic and Conventional Horticultural Systems under Greenhouse and Open Field Management: A Case Study.” Journal of Soils and Sediments 11: 25–36. doi:10.1007/s11368-010-0293-4.
- Ge, T. D., S. W. Song, P. Roberts, D. L. Jones, D. F. Huang, and K. Iwasaki. 2009. “Amino Acids as a Nitrogen Source for Tomato Seedlings: The Use of Dual-labeled (13C, 15N) Glycine to Test for Direct Uptake by Tomato Seedlings.” Environmental and Experimental Botany 66: 357–361. doi:10.1016/j.envexpbot.2009.05.004.
- Gioseffi, E., A. de Neergaard, and J. K. Schjoerring. 2012. “Interactions between Uptake of Amino Acids and Inorganic Nitrogen in Wheat Plants.” Biogeosciences 9 (4): 1509–1518. doi:10.5194/bg-9-1509-2012.
- Harrison, K. A., R. Bol, and R. D. Bardgett. 2008. “Do Plant Species with Different Growth Strategies Vary in Their Ability to Compete with Soil Microbes for Chemical Forms of Nitrogen?” Soil Biology and Biochemistry 40: 228–237. doi:10.1016/j.soilbio.2007.08.004.
- Henry, H. A. L., and R. L. Jefferies. 2003. “Plant Amino Acid Uptake, Soluble N Turnover and Microbial N Capture in Soils of a Grazed Arctic Salt Marsh.” Journal of Ecology 91: 627–636. doi:10.1046/j.1365-2745.2003.00791.x.
- Hirner, A., F. Ladwig, H. Stransky, S. Okumoto, M. Keinath, A. Harms, W. B. Frommer, and W. Koch. 2006. “Arabidopsis LHT1 Is a High-affinity Transporter for Cellular Amino Acid Uptake in Both Root Epidermis and Leaf Mesophyll.” The Plant Cell 18: 1931–1946. doi:10.1105/tpc.106.041012.
- Hu, L. Z., W. B. Yin, Y. H. Chen, and Z. M. Hu. 2014. “Functional Divergence and Evolutionary Dynamics of the Putative AAAP Gene Family in Brassica Rapa.” Plant Molecular Biology Reporter 32: 517–530. doi:10.1007/s11105-013-0671-3.
- Jamtgard, S., T. Näsholm, and K. Huss-Danell. 2008. “Characteristics of Amino Acid Uptake in Barley.” Plant and Soil 302: 221–231. doi:10.1007/s11104-007-9473-4.
- Jiang, L. L., S. P. Wang, Z. Pang, C. S. Wang, P. Kardol, X. Q. Zhou, Y. C. Rui, Z. C. Lan, Y. F. Wang, and X. L. Xu. 2016. “Grazing Modifies Inorganic and Organic Nitrogen Uptake by Coexisting Plant Species in Alpine Grassland.” Biology and Fertility of Soils 52: 211–221. doi:10.1007/s00374-015-1069-1.
- Jones, D. L., D. Shannon, T. Junvee-Fortune, and J. F. Farrarc. 2005. “Plant Capture of Free Amino Acids Is Maximized under High Soil Amino Acid Concentrations.” Soil Biology and Biochemistry 37: 179–181. doi:10.1016/j.soilbio.2004.07.021.
- Jones, D. L., D. K. Shannon, D. V. Murphy, and J. F. Farrar. 2004. “Role of Dissolved Organic Nitrogen (DON) in Soil N Cycling in Grassland Soils.” Soil Biology and Biochemistry 36: 749–756. doi:10.1016/j.soilbio.2004.01.003.
- Khalil, M. A., A. I. M. Alassiuty, N. M. Van Straalen, and B. A. Alassiuty. 2015. “Changes in Soil Oribatid Communities Associated with Conversion from Conventional to Organic Agriculture.” Experimental and Applied Acarology 68: 183–196. doi:10.1007/s10493-015-9979-z.
- Kirkham, D., and W. V. Bartholomew. 1955. “Equations for following Nutrient Transformations in Soil, Utilizing Tracer Data: II.” Soil Science Society of America Journal 19: 189–192. doi:10.2136/sssaj1955.03615995001900020020x.
- Lee, Y.-H., J. Foster, J. Chen, L. M. Voll, A. P. M. Weber, M. Tegeder. 2007. “AAP1 Transports Uncharged Amino Acids into Roots of Arabidopsis.” The Plant Journal 50 (2): 305–319. DOI:10.1111/j.1365-313X.2007.03045.x.
- Liu, T. K., W. Dai, F. F. Sun, X. D. Yang, A. S. Xiong, and X. L. Hou. 2014. “Cloning and Characterization of the Nitrate Transporter Gene BraNRT2.1 In Non-heading Chinese Cabbage.” Acta Physiologiae Plantarum 36: 815–823. doi:10.1007/s11738-013-1460-1.
- Miller, A. E., and W. D. Bowman. 2003. “Alpine Plants Show Species-level Differences in the Uptake of Organic and Inorganic Nitrogen.” Plant and Soil 250: 283–292. doi:10.1023/A:1022867103109.
- Moranzuloaga, D., M. Dippold, B. Glaser, and Y. Kuzyakov. 2015. “Organic Nitrogen Uptake by Plants: Reevaluation by Position-specific Labeling of Amino Acids.” Biogeochemistry 125: 359–374. doi:10.1007/s10533-015-0130-3.
- Murphy, K. M., K. G. Campbell, S. R. Lyon, and S. S. Jones. 2007. “Evidence of Varietal Adaptation to Organic Farming Systems.” Field Crops Research 102: 172–177. doi:10.1016/j.fcr.2007.03.011.
- Muruganandam, S., D. W. Israel, and W. P. Robarge. 2009. “Activities of Nitrogen-mineralization Enzymes Associated with Soil Aggregate Size Fractions of Three Tillage Systems.” Soil Science Society of America Journal 73: 751–759. doi:10.2136/sssaj2008.0231.
- Näsholm, T., K. Huss-Danell, and P. Högberg. 2000. “Uptake of Organic Nitrogen in the Field by Four Agriculturally Important Plant Species.” Ecology 81: 1155–1161. doi:10.2307/177188.
- Nie, M., M. Lu, Q. A. Yang, X. D. Zhang, M. Xiao, L. F. Jiang, J. Yang, C. M. Fang, J. K. Chen, and B. Li. 2011. “Plants’ Use of Different Nitrogen Forms in Response to Crude Oil Contamination.” Environmental Pollution 159: 157–163. doi:10.1016/j.envpol.2010.09.013.
- Orr, C., A. M. James, C. Leifert, J. Cooper, and S. P. Cummings. 2011. “Diversity and Activity of Free-living Nitrogen-fixing Bacteria and Total Bacteria in Organic and Conventionally Managed Soils.” Applied and Environmental Microbiology 77: 911–919. doi:10.1128/AEM.01250-10.
- Orr, C. H., C. Leifert, S. P. Cummings, and J. M. Cooper. 2012. “Impacts of Organic and Conventional Crop Management on Diversity and Activity of Free-living Nitrogen Fixing Bacteria and Total Bacteria are Subsidiary to Temporal Effects.” PloS One 7: e52891. doi:10.1371/journal.pone.0052891.
- Papadopoulos, A., N. R. A. Bird, A. P. Whitmore, and S. J. Mooney. 2014. “Does Organic Management Lead to Enhanced Soil Physical Quality.” Geoderma 213: 435–443. doi:10.1016/j.geoderma.2013.08.033.
- Perez, P. G., J. Ye, S. Wang, X. Wang, and D. Huang. 2014. “Analysis of the Occurrence and Activity of Diazotrophic Communities in Organic and Conventional Horticultural Soils.” Applied Soil Ecology 79: 37–48. doi:10.1016/j.apsoil.2014.03.006.
- Raab, T. K., D. A. Lipson, and R. K. Monson. 1999. “Soil Amino Acid Utilization among Species of the Cyperaceae: Plant and Soil Processes.” Ecology 80: 2408–2419. doi:10.1890/0012-9658(1999)080[2408:SAAUAS]2.0.CO;2.
- Reeve, J. R., C. W. Schadt, L. Carpenterboggs, S. Kang, J. Zhou, and J. P. Reganold. 2010. “Effects of Soil Type and Farm Management on Soil Ecological Functional Genes and Microbial Activities.” Isme Journal 4: 1099–1107. doi:10.1038/ismej.2010.42.
- Reeve, J. R., J. L. Smith, L. Carpenter-Boggs, and J. P. Reganold. 2009. “Glycine, Nitrate, and Ammonium Uptake by Classic and Modern Wheat Varieties in a Short-term Microcosm Study.” Biology and Fertility of Soils 45: 723–732. doi:10.1007/s00374-009-0383-x.
- Reid, T. A., R. Yang, D. F. Salmon, and D. Spaner. 2009. “Should Spring Wheat Breeding for Organically Managed Systems Be Conducted on Organically Managed Land.” Euphytica 169: 239–252. doi:10.1007/s10681-009-9949-9.
- Rippy, J. F. M., M. M. Peet, F. J. Louws, P. V. Nelson, D. B. Orr, and K. A. Sorensen. 2004. “Plant Development and Harvest Yields of Greenhouse Tomatoes in Six Organic Growing Systems.” Hortscience 39: 223–229. doi:10.21273/HORTSCI.39.2.223.
- Sauheitl, L., B. Glaser, and A. Weigelt. 2009. “Uptake of Intact Amino Acids by Plants Depends on Soil Amino Acid Concentrations.” Environmental and Experimental Botany 66: 145–152. doi:10.1016/j.envexpbot.2009.03.009.
- Sun, J., and N. Zheng. 2015. “Molecular Mechanism Underlying the Plant NRT1.1 Dual-affinity Nitrate Transporter.” Frontiers in Physiology 6: 386. doi:10.3389/fphys.2015.00386.
- Thornton, B., and D. Robinson. 2005. “Uptake and Assimilation of Nitrogen from Solutions Containing Multiple N Sources.” Plant Cell Environment 28: 813–821. doi:10.1111/j.1365-3040.2005.01332.x.
- Van Bueren, E. T. L., S. S. Jones, L. Tamm, K. M. Murphy, J. R. Myers, C. Leifert, and M. M. Messmer. 2011. “The Need to Breed Crop Varieties Suitable for Organic Farming, Using Wheat, Tomato and Broccoli as Examples: A Review.” Njas-Wageningen Journal Life Science 58: 193–205. doi:10.1016/j.njas.2010.04.001.
- Vinolas, L. C., J. R. Healey, and D. L. Jones. 2001. “Kinetics of Soil Microbial Uptake of Free Amino Acids.” Biology and Fertility of Soils 33: 67–74. doi:10.1007/s003740000291.
- Wang, X., D. Tang, and D. Huang. 2014. “Proteomic Analysis of Pakchoi Leaves and Roots under Glycine-nitrogen Conditions.” Plant Physiology and Biochemistry 75: 96–104. doi:10.1016/j.plaphy.2013.12.012.
- Wang, X., R. Han, D. Tang, and D. Huang. 2015. “Comparison of Glycine Uptake by Pak Choi in Organic and Conventional Soil under Different Glycine Concentrations: A Pot Study.” Journal of Plant Nutrition and Soil Science 178: 768–775. doi:10.1002/jpln.201400587.
- Wang, X. L., J. Ye, P. G. Perez, D. M. Tang, and D. F. Huang. 2013. “The Impact of Organic Farming on the Soluble Organic Nitrogen Pool in Horticultural Soil under Open Field and Greenhouse Conditions: A Case Study.” Soil Science and Plant Nutrition 59: 1–12. doi:10.1080/00380768.2013.770722.
- Warren, C. R. 2009. “Uptake of Inorganic and Amino Acid Nitrogen from Soil by Eucalyptus Regnans and Eucalyptus Pauciflora Seedlings.” Tree Physiology 29: 401–409. doi:10.1093/treephys/tpn037.
- Witt, C., J. L. Gaunt, C. C. Galicia, J. C. G. Ottow, and H. U. Neue. 2000. “A Rapid Chloroform-fumigation Extraction Method for Measuring Soil Microbial Biomass Carbon and Nitrogen in Flooded Rice Soils.” Biology and Fertility of Soils 30: 510–519. doi:10.1007/s003740050030.
- Xu, X. L., C. F. Stange, A. Richter, W. Wanek, and Y. Kuzyakov. 2008. “Light Affects Competition for Inorganic and Organic Nitrogen between Maize and Rhizosphere Microorganisms.” Plant and Soil 304: 59–72. doi:10.1007/s11104-007-9519-7.
- Yim, M. H., S. J. Joo, and K. Nakane. 2002. “Comparison of Field Methods for Measuring Soil Respiration: A Static Alkali Absorption Method and Two Dynamic Closed Chamber Methods.” Forest Ecology and Management 170: 189–197. doi:10.1016/S0378-1127(01)00773-3.