ABSTRACT
Chitin, which is the polymer of N-acetylglucosamine (GlcNAc) linked by β1,4 glycoside bonds, has been reported as a soil amendment to mitigate plant soil diseases, increasing the population of chitin-degrading bacteria, and chitinolytic enzymatic activity in the soil. In some chitin-degrading bacteria, whose chitinolytic systems have been intensively studied, the chitin degradation product N,Nʹ-diacetylchitobiose {(GlcNAc)2} induces expression of genes for chitinases whereas GlcNAc does not. To evaluate the effects of these mono- and disaccharides on the population and activity of chitinolytic bacteria in soil, we investigated the chitinolytic enzyme activity and bacterial community structure in an incubated upland soil supplemented with GlcNAc or (GlcNAc)2. The added GlcNAc and (GlcNAc)2 (2 mg g−1) were consumed within 1 d when incubated at 25°C. Chitinase activity was induced by (GlcNAc)2 and chitin after 1-d and 7-d incubation, respectively, but not by GlcNAc. N-acetylglucosaminidase (GlcNAcase) activity was induced by GlcNAc but was lower than those by (GlcNAc)2 and chitin. Amplicon sequencing analysis targeting 16S rRNA genes demonstrated that both GlcNAc and (GlcNAc)2 significantly increased the rate of the order Bacillales, but the compositions of Bacillales differed from each other: the family Planococcaceae significantly increased in either GlcNAc- or (GlcNAc)2-added soil, but the genera Bacillus and Paenibacillus were increased mainly by GlcNAc and (GlcNAc)2, respectively. The family Streptomycetaceae of the order Actinomycetales was significantly increased by (GlcNAc)2 and chitin, but GlcNAc did not. Thus, GlcNAc and (GlcNAc)2, which were promptly consumed in the incubated soil, indicated partly similar but distinctive effects on chitinolytic enzyme activity and bacterial communities. Both aminosugars increased GlcNAcase activity and the population size of Planococcaceae. GlcNAc increased Bacillus. Chitinase activity and the populations of Paenibacillus and Streptomycetaceae, a number of strains of which are known as potent chitin-degraders, were increased by (GlcNAc)2, but not by GlcNAc.
KEY WORDS:
1. Introduction
Chitin, which is a polymer of N-acetyl-D-glucosamine (GlcNAc), is the second most abundant biopolymer in nature after cellulose (Muzzarelli Citation1977; Deshpande Citation1986; Gooday Citation1991). Chitin is found in the shells of crabs and shrimps as well as in the cell walls of fungi and cuticles of insects. The amendment of soil with chitin has been shown to suppress plant-pathogenic fungi (Mitchell and Alexander Citation1962; Buxton, Khalifa, and Ward Citation1965; Khalifa Citation1965; Henis, Sneh, and Katan Citation1967; Sneh, Katan, and Henis Citation1971; Sneh and Henis Citation1972; Van Eck Citation1978; Murakami et al. Citation2009) and nematodes (Mian et al. Citation1982; Sarathchandra et al. Citation1996). In most, but not all cases, the mechanisms behind this suppressive activity are often related to a change in the structure and/or activity of the microbiota in the soil, which subsequently suppresses plant pathogens (Mendes et al. Citation2011; Weller et al. Citation2002). Presumably, chitinolytic microorganisms, which are capable of hydrolyzing the chitinous hyphae of pathogenic fungi, increased their numbers and/or activities in response to the chitin added. Alternatively, secondary responders to the added chitin confer pathogen suppression (Cretoiu et al. Citation2013).
16S rRNA gene analysis showed that Actinobacteria and Oxalobacteraceae populations increased in chitin-treated soils {1.8% of shrimp waste chitin (20 tons ha−1)} (Cretoiu et al. Citation2013). Another study reported that chitin enrichment (2 mg g−1 and 20 mg g−1 colloidal chitin) led to an increase of Actinobacteria, γ-Proteobacteria, and β-Proteobacteria, suggesting the specific selection of these chitin-degrading bacteria (Jacquiod et al. Citation2013). A study indicated that various chiA genotypes including β-Proteobacteria, γ-Proteobacteria, Actinobacteria, Acidobacteria, Bacteroidetes, Firmicutes, Planctomycetes, and Chloroflexi responded to chitin supplementation in soil slurries (0.2 g 80 mL−1) (Wieczorek, Hetz, and Kolb Citation2014). Our recent study indicated the temporal changes of bacterial community structure in an incubated upland soil treated with chitin (60 g kg−1), in which Streptomyces increased drastically and dominated in the bacterial community structure soon after the addition of chitin (Kumeta et al. Citation2018; Iwasaki, Ichino, and Saito Citation2020).
In order to utilize chitinolytic bacteria and enzymes to control plant disease, a number of chitin-degrading bacterial strains were isolated. In some chitinolytic soil bacterial strains of the genera Streptomyces, Serratia, and Paenibacillus, intensive studies have been conducted to elucidate chitinolytic systems including the properties and structures of the corresponding enzymes and the regulation of the genes encoding the chitinolytic enzymes (Itoh and Kimoto Citation2019, for review). These bacteria produce multiple extracellular chitinases, which hydrolyze chitin into mainly (GlcNAc)2. Interestingly, (GlcNAc)2 has been commonly identified as the smallest unit of chitin degradation product that induces chitinase production in those chitinolytic bacterial strains (Miyashita, Fujii, and Saito Citation2000; Saito et al. Citation2000; Uchiyama et al. Citation2003; for review, see Itoh and Kimoto Citation2019). (GlcNAc)2 is taken up into the cells of chitinolytic bacteria via specific transporters and induces chitinase genes (Uchiyama et al. Citation2003; Saito et al. Citation2007; Iinuma et al. Citation2018). In contrast, GlcNAc does not induce and rather repress the promoter of the gene for chitinase A in Streptomyces lividans (Miyashita, Fujii, and Saito Citation2000). In Paenibacillus sp. FPU-7, GlcNAc exerted a severe inhibitory effect on the synthesis of secreted chitinases (Itoh et al. Citation2013).
It is thus assumed that (GlcNAc)2 would induce chitinase activity in soil, although the assumption has not been experimentally investigated. Here we report the effects of (GlcNAc)2 on chitinolytic enzyme activity and bacterial community structure in an incubated upland soil, which were compared with those of GlcNAc and chitin. The consumption profiles of (GlcNAc)2 and GlcNAc in the incubated upland soil are also investigated.
2. Materials and methods
2.1. Preparation of soil samples
The surface soil of Brown Forest soil (Light Clay) in an upland field at the Center for Education and Research, Field Sciences (at a latitude of 34.905502 north and a longitude of 138.272157 east), Faculty of Agriculture, Shizuoka University, was sampled in 2013 (Kumeta et al. Citation2018; Iwasaki, Ichino, and Saito Citation2020). After air-drying at room temperature, the soil sample was passed through a sieve with 2 mm meshes. The physicochemical properties of the dried soil are shown in . Chitin powder and GlcNAc were purchased from Wako Chemicals, Japan. (GlcNAc)2 was prepared and kindly supplied by Yaizu Suisankagaku Industry. To 154 g of the air-dried sieved soil in a box, 0.4 g of autoclaved chitin powder or 4 mL of 10% (w/v) GlcNAc or (GlcNAc)2 solution [final ca. 2.0 g kg−1] was added and mixed. The water content was kept at 60% of the maximum water holding capacity. The box was covered with a lid with pine halls and incubated at 25°C. For analyses, the soil was taken from 10 distinctive points in the box, mixed, and stored at −80°C.
Table 1. Selected physicochemical properties of the air-dried Brown Forest soil examined in this study and used in our previous report (Kumeta et al. Citation2018).
2.2. Measurement of GlcNAc and (GlcNAc)2 in soil by gas chromatography – mass spectrometry (GC/MS)
For standards, 230 μL of sterilized MilliQ water containing a series of GlcNAc or (GlcNAc)2 at different contents were added to 0.77 g dried soil to adjust the water content to 60% of the maximum water holding capacity and to give the final contents (0, 0.5, 1.0, 1.5, and 2.0 g kg−1) of GlcNAc or (GlcNAc)2. The soil samples for each content of GlcNAc or (GlcNAc)2 were prepared in triplicate and subjected to the following extraction process. To extract GlcNAc and (GlcNAc)2 from soil samples, 0.5 g soil sample was suspended in 2 mL of sterilized MilliQ water containing 5 μg xylitol as the inner standard and was shaken at 200 rpm for 30 min on ice. The suspension was centrifuged (15,600 g, 5 min, 4°C), and the supernatant was treated as soil extract. The soil extracts were freeze-dried and subjected to a methoxime-trimethylsilylation reaction as follows. Fifty microliters of methoxyme reagent (20 mg mL−1 methoxyamine hydrochloride in pyridine) were added to the freeze-dried soil extract and incubated at 30°C for 90 min. Then, 100 μL of N-methyl-N-trimethylsilyltrifluoroacetamide (MSTFA) (GL Science) was added and kept at 39°C for 30 min. After 900 μL of hexane was added, the mixture was centrifuged at 17,900 g for 4 min at 4°C. The supernatant was analyzed in a GC/MS system GCMS-QP2020 (Shimadzu) equipped with a DB-5 capillary column (Agilent, Santa Clara, CA, USA). The temperature conditions were as follows: 150°C (2 min, constant) - (5°C/min) - 250°C (1 min, constant) - (30°C/min) - 320°C (4 min, constant). Xylitol, GlcNAc, and (GlcNAc)2 were detected and identified at 217, 319, and 420 m/z at their corresponding retention times, respectively. GlcNAc and (GlcNAc)2 contents were calculated based on the ratio of the peak areas to that of xylitol.
2.3. Measurement of ammonium, nitrite, and nitrate contents
To measure the ammonium nitrogen (NH4+-N), nitrite nitrogen (NO2–N), and nitrate nitrogen (NO3–N) contents, one gram of each soil sample was suspended in 10 mL of 2 mol L−1 potassium chloride (KCl), shaken at 250 rpm for 1 h, and centrifuged at 3,000 rpm for 1 min, and the supernatant was subjected to assays. NH4+-N content was measured by the indophenol method. NO2–N content was measured by the Greiss reaction. NO3− was reduced using hydradine sulfate to measure the NO3–N content.
2.4. Chitinase and N-acetylglucosaminidase assay
Two grams of each of soil sample was suspended in 10 mL of 0.1 mol L−1 potassium phosphate buffer (pH 7.0), shaken at 250 rpm for 30 min, and centrifuged at 1,500 g for 10 min. The supernatant was passed through filter paper. Chitinase activity was measured by using 4-methylumbelliferyl-N,N’-diacetylchitobioside (Sigma-Aldrich) according to a previously described method (Miyashita, Fujii, and Sawada Citation1991). N-acetylglucosaminidase activity was evaluated using 4-methylumbelliferyl-N-acetyl-β-D-glucosamine (Sigma) as the substrate as reported previously (Saito et al. Citation2013). One unit of chitinase or N-acetylglucosaminidase activity was defined as the amount of enzyme that liberated 1 × 10−6 mol of 4-metylumbelliferone from the corresponding substrate in one minute at 37°C.
2.5. Analysis of bacterial community structure
DNA was extracted from 0.4 g of each soil sample using a FastDNA SPIN Kit for Soil (MP Biomedicals), according to a previously reported method (Takada-Hoshino and Matsumoto Citation2004). PCR was performed to amplify the V4 region of the 16 S rRNA gene using the primers 515F (5ʹ-ACACTCTTTCCCTACACGACGCTCTTCCGATCT-GTGCCAGCMGCCGCGGTAA-3ʹ) and 806R (5ʹ-GTGACTGGAGTTCAGACGTGTGCTCTTCCGATCT-GGACTACHVGGGTWTCTAAT-3ʹ) containing sequencer adopter regions (Caporaso et al. Citation2011) with ExTaq DNA polymerase (Takara Bio, Shiga, Japan). The PCR products of the corresponding sizes were purified using a Wizard SV Gel and PCR Clean-Up Kit (Promega). PCR fragments (15 ng) were subjected to paired-end sequencing using an Illumina MiSeq platform (Illumina) at Fasmac (Atsugi, Japan). Sequences were quality filtered and analyzed with the Quantitative Insights Into Microbial Ecology 2 (QIIME2) pipeline (version 2018.8 [Bolyen et al. Citation2019]). The remaining sequences (see Table S1 for the detailed number of sequences) were clustered into OTUs at the 97% level and classified using the Greengenes 13–8 reference database (McDonald et al. Citation2012), as implemented in QIIME2. Close relatives of representative OTUs were identified by a BLASTn analysis and phylogenetic analysis using the SILVA database (SILVA SSU Ref NR_128 database) and backbone tree (tree_SSURefNR99_1200slv_128) in the ARB program for sequence analysis (Ludwig et al. Citation2004). Mann–Whitney U test was used to evaluate the significance of the differences in the abundance of bacteria at the level of order, family, and genus.
Illumina sequencing data were deposited in the DDBJ/ENA/GenBank database under BioProject ID PRJDB9100 and BioSample ID SAMD00198556-00198567 and SAMD00207327-SAMD00207346.
2.6. Bacterial strains and cultivation
Paenibacillus glucanolyticus NBRC 15330 T, P. ehimensis NBRC 15659 T, P. chitinolyticus NBRC 15660 T, P. humicus NBRC 102415 T, P. taihuensis NBRC 108766 T, P. lemnae NBRC 109972 T, Bacillus subtilis NBRC 13719 T (= Marburg), B. licheniformis NBRC 12200 T, B. circulans NBRC 13626 T, B. megaterium NBRC 15308 T, and B. amyloliquefaciens NBRC 15535 T were supplied by the Biological Resource Center, NITE (NBRC, Japan). The strains were inoculated into minimal medium (MM) (10 mM K2HPO4, 10 mM KH2PO4, 1 mM CaCl2, 0.5 mM MgCl2 supplemented with 0.1% [v/v] trace element solution) containing 0.1% (w/v) carbon source: GlcNAc, (GlcNAc)2, glucose, or colloidal chitin. Cultures were done at 30°C with shaking at 150 rpm for 4 d. Growth was observed by the increment in turbidity of media supplemented with GlcNAc, (GlcNAc)2, or glucose, whereas growth was detected by the decrease in turbidity of medium supplemented with colloidal chitin. The ability of bacteria strain to utilize GlcNAc, (GlcNAc)2, glucose, or colloidal chitin as carbon source was judged from the growth. Yeast extract, peptone, and/or soy peptone [0.01% (w/v)] were added as supplements for bacterial strains that did not grow in MM.
3. Results
3.1. Quantification of GlcNAc and (GlcNAc)2 added to soil by GC/MS
To examine if GC/MS is applicable for determining GlcNAc and (GlcNAc)2 contents in soil, GlcNAc and (GlcNAc)2 added to soil were extracted and treated with methoxyme reagent followed by MSTFA to hydrophobize the amino sugars by methoxymation and trimethylsilylation. The derivatized compounds in the soil extract were then analyzed with GC/MS as described in the Materials and Methods. We used the ratio between the peak areas of the GlcNAc or (GlcNAc)2 derivative(s) and that of the internal control (xylitol) in order to prepare a standard curve for measuring the amount of GlcNAc and (GlcNAc)2 in soil. The ratios correlated nicely (R2 = 0.990 and 0.982 for GlcNAc or (GlcNAc)2, respectively) in the range below 2 mg g−1 (). The slope of the approximate line for GlcNAc was about four times higher than that of (GlcNAc)2, indicating that the detection sensitivity for GlcNAc is higher than that for (GlcNAc)2. In fact, the ratios between the peak areas of GlcNAc- and xylitol-derivatives correlated nicely (R2 = 0.998) in the range of 0.1–300 μg g−1, while the peak ratios of (GlcNAc)2 and xylitol also correlated (R2 = 0.955) but in the higher range (> 10 μg g−1) (data not shown). We thus concluded that GlcNAc and (GlcNAc)2 contents were measurable at least in the range of 0.1 μg g−1 – 2 mg g soil−1 and 10 μg g−1 – 2 mg g soil−1, respectively, with the derivatization conditions and GC/MS system we used.
Figure 1. Relationship between contents in soil and ratios of peaks detected by GC/MS analysis of GlcNAc (left) and (GlcNAc)2 (right). The averages of measured contents of standard soil samples prepared in triplicate are plotted. The result of linear approximation is shown by a formula with its determination coefficient.
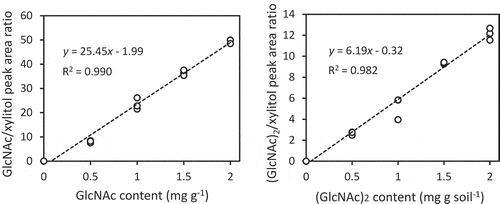
3.2. Consumption profiles of GlcNAc or (GlcNAc)2 added to incubated soil
The consumption of GlcNAc or (GlcNAc)2 was investigated in an incubated upland soil. The decreasing patterns of GlcNAc and (GlcNAc)2 were similar (). The added GlcNAc and (GlcNAc)2 were not detectable in the samples collected 1 d after the addition of the corresponding amino sugars. GlcNAc, which could be liberated by (GlcNAc)2 hydrolysis, was not detected in the soil treated with (GlcNAc)2 except for the 0.4-d soil, in which 40 μg g−1 GlcNAc was detected. In contrast, GlcNAc was detected (0.2–0.6 μg g−1) in the chitin-treated soil after incubation for 3–12 d. The accumulation of ammonia/ammonium, which arises together with GlcNAc/(GlcNAc)2 consumption, occurred transiently (after 1–2 d incubation) in the GlcNAc/(GlcNAc)2-added soil, whereas ammonia/ammonium accumulation was not observed in the incubated soil treated with chitin powder (Figure S1). We conclude that GlcNAc and (GlcNAc)2 were easily consumed within 1 d and that the consumption profiles were similar to each other.
Figure 2. GlcNAc and (GlcNAc)2 contents in the incubated upland soil supplemented with 0.2% (w/v) GlcNAc (right), (GlcNAc)2 (middle), and chitin (right). GlcNAc contents, circles; (GlcNAc)2, triangles. Soil samples were prepared in duplicate and the average contents are shown. Error bars indicate the maximum and minimum contents of the duplicated soil samples.
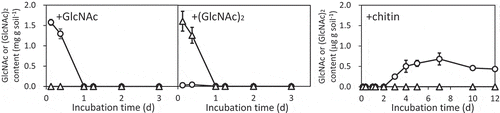
3.3. Effects of GlcNAc and (GlcNAc)2 on chitinase and N-acetylglcosminidase activity
In the presence of chitin, chitinase activity seemed to be transient: activity increased until 7 d, then decreased ()), as we previously observed in upland soil to which chitin was added (Kumeta et al. Citation2018). In contrast, significant chitinase activity was not detected in the GlcNAc-added soil, whereas (GlcNAc)2 induced chitinase activity temporarily after 1 d. N-acetylglucosaminidase (GlcNAcase) activity increased not only with chitin but also GlcNAc and (GlcNAc)2, although the levels and patterns differed from each other ()). In the presence of chitin, GlcNAcase activity gradually increased, peaking at 10 d. The timing of the peak of GlcNAcase activity was later than chitinase activity, as reported previously (Kumeta et al. Citation2018). In contrast, GlcNAcase activity increased earlier and temporarily after 1–4 d in the soil treated with (GlcNAc)2. GlcNAc also increased GlcNAcase activity. The timing of the increment with GlcNAc was similar with that in the (GlcNAc)2-added soil, but the level in the presence of GlcNAc was lower than that induced by (GlcNAc)2.
Figure 3. Chitinase (a) and N-acetylglucosaminidase (GlcNAcase) (b) activity in incubated upland soil supplemented with 0.2% (w/v) N-acetylglucosamine (closed circles), N,N’-diacetylchitobiose (closed triangles), powdered chitin (closed squares), or without supplementation (open circles). GlcNAcase and chitinase activities were measured using 4-methylumbelliferyl-N-acetylglucosaminide and 4-methylumbelliferyl-N,N’-diacetylchitobioside, respectively. Soil samples were prepared in duplicate and the average enzymatic activities are shown. Error bars indicate the maximum and minimum activities of the duplicated soil samples.
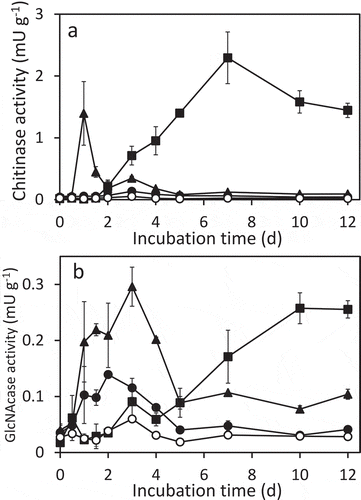
3.4. Effects of GlcNAc and (GlcNAc)2 on bacterial community structure
Amplicon sequencing analysis targeting 16S rRNA genes indicated that GlcNAc and (GlcNAc)2 significantly increased the rates of the order Bacillales (p < 0.01), which increased to 77–89% and 69%, respectively, after 1-d of incubation, whereas the population rate was 8–10% in the control soil samples without supplementation (, red parts). The family Planococcus of Bacillales accounted for 42–50% and 25% of the Prokaryotes after 1 d in GlcNAc- and (GlcNAc)2-supplemented soil samples, respectively (, yellow parts). The genus Bacillus significantly increased by GlcNAc (p < 0.01). Among the 1-d samples, the genus Paenibacillus belonging to Bacillales occupied 30–33% in the (GlcNAc)2-treated soil, but 2% in the GlcNAc-supplemented soil (, orange parts). The abundance of Paenibacillus was significantly dominated more in the (GlcNAc)2-added soil than in soils without supplementation and with chitin (p < 0.01), but was not significantly than by GlcNAc (p = 0.059). Although the abundance of the order Actinomycetales was not affected by the addition of any of the sugars (p > 0.2), the family Streptomycetaceae significantly increased by (GlcNAc)2 and chitin (p < 0.05). In the 1-d soil samples, the family Streptomycetaceae including the genus Streptomyces also increased to 8–9% by (GlcNAc)2 (, parts in light green and green). In the chitin-added soil, Streptomycetaceae including Streptomyces increased gradually and reached 19–23% after 7 d. The rate of the genus Arthrobacter significantly decreased by chitin (p < 0.05).
3.5. Growth of Paenibacillus and Bacillus type strains in the presence of GlcNAc or (GlcNAc)2
Six and five type strains of the genera Paenibacillus and Bacillus, respectively, were selected and cultured to test their ability to utilize GlcNAc and (GlcNAc)2. Five among the six tested Paenibacillus species had an ability to utilize both GlcNAc and (GlcNAc)2, while all six Paenibacillus species were able to utilize glucose (). This suggests that most Paenibacillus species can utilize both GlcNAc and (GlcNAc)2 as a carbon source. Bacillus subtilis and B. amyloliquefaciens, in contrast, did not indicate an ability to utilize (GlcNAc)2, but utilized GlcNAc and glucose. The other Bacillus strains (B. licheniformis, B. circulans, and B. megaterium) utilized both GlcNAc and (GlcNAc)2, but their growths in the presence of (GlcNAc)2 were slower than in GlcNAc (data not shown). These data imply that Bacillus species prefer GlcNAc to (GlcNAc)2 as carbon source. Three among the six Paenibacillus species and one among the five Bacillus strains utilized chitin as the carbon source (). Chitinolytic properties might be more common in Paenibacillus than in Bacillus.
Table 2. Carbon source utilization by type strains of Paenibacillus and Bacillus.
4. Discussion
In this report, we investigated the effects of GlcNAc and (GlcNAc)2 on soil chitinolytic enzyme activities and bacterial community structure in comparison with those of chitin. As we presumed, chitinase activity was induced by (GlcNAc)2, but not by GlcNAc ()). As far as we know, this is the first experimental data indicating that (GlcNAc)2 induced soil chitinase activity. Addition of (GlcNAc)2 increased the populations of Paenibacillus and Streptomycetaeae, which are known as chitinolytic bacteria. Paenibacillus dominated more than Streptomycetaceae in the soil samples supplemented with (GlcNAc)2, whereas Streptomycetaceae increased more than Paenibacillus in the chitin-added soil (). (GlcNAc)2 and chitin powder should be different in their distribution in soil aggregates, because they are soluble and insoluble, respectively. This might be a reason for distinctive patterns of increase of Paenibacillus and Streptomycetaceae.
Both of GlcNAc and (GlcNAc)2 were readily consumed in the incubated soil within 1 d (). In an oxic agricultural soil slurry, in which soil and water were mixed at a 1:2.5 ratio, the supplemented GlcNAc (0.25 mM = 55 mg L−1) and (GlcNAc)2 (0.10 mM = 42 mg L−1) were similarly consumed within 22 and 20 h, respectively, at 20°C (Wieczorek, Hetz, and Kolb Citation2014). The consumption profiles of GlcNAc and (GlcNAc)2 observed in this study thus seem consistent with those in the slurry experiment.
In the chitin-added soil, low level of GlcNAc (around 0.5 μg g−1, which corresponds to 2 μmol kg−1 and 0.03 mg N kg−1) was detected during the incubation period form 4–12 d, whereas GlcNAc was not detected after 1 d and later in the soils treated with GlcNAc or (GlcNAc)2 (). It is unclear why the low-level GlcNAc was not consumed even after 12 d in the chitin-added soil. Such level of GlcNAc is able to be consumed by soil bacteria because it is indicated that GlcNAc at 15 μmol L−1 was consumed in Streptomyces coelicolor (Iinuma et al. Citation2018), which is a member of the family Streptomycetaceae having increased in the chitin-added soil samples (). The number of culturable bacteria was increasing during the incubation period in the chitin-added soil (data not shown). Microorganisms in the chitin-treated soil might prefer chitin which is assumed to still remain even after 12 days, to the coexistent GlcNAc. The remaining GlcNAc might have been consumed after complete chitin-consumption.
Figure 4. Abundance ratio of Prokaryote orders (a), the order Bacillliales (b), and the order Actinomycetales in the upland soil incubated with GlcNAc, (GlcNAc)2, or powdered chitin. Bacterial orders accounting for less than 0.01 (1%) were compiled as ‘other bacteria’, which is indicated by stripes in A. (a) Red parts represent the order Bacillales; sky blue, order Actinomycetales. (b) Yellow, family Planococcaceae; orange, genus Paenibacillus; brown, genus Bacillus; black, other Bacillales. (c) Light-green, genus Streptomyces; green, family Streptomycetaceae; sky blue, family Nocaudioidaceae; blue, genus Catellatospora; purple, genus Arthrobacter; gray, genus Phycicoccus; black, family Intrasporangiaceae.
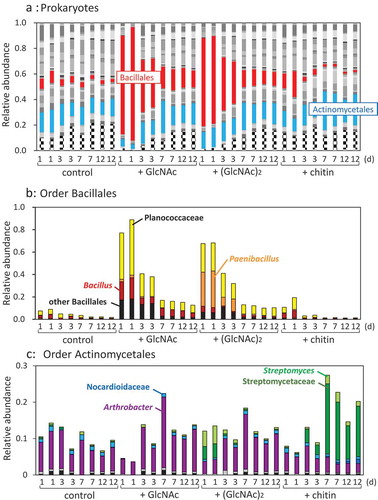
The contents of ammonia/ammonium in the GlcNAc/(GlcNAc)2-treated soil peaked around 100 mg N kg−1 (Figure S1), which approximately coincided with those of added nitrogen as GlcNAc and (GlcNAc)2 (about 130 mg N kg−1). The released ammonia/ammonium may have been assimilated by microbes in the corresponding soil samples. In the chitin-added soil, it is assumed that the mineralization rate of chitin (release rate of ammonia/ammonium) and the assimilation rate of mineralized nitrogen (ammonia/ammonium) may have been similar with each other because the consumption rate of chitin is supposed to be slower than those of GlcNAc and (GlcNAc)2.
These responses of the soil to chitin and (GlcNAc)2 in chitinase production were similar to those of Streptomyces coelicolor A3(2), although the timing of the responses was earlier in S. coelicolor A3(2) (Saito et al. Citation2000) than in the soil observed in this study ()). In S. coelicolor, it has been reported that (GlcNAc)2 induces transcription of chitinase genes after 1 h and chitinase activity after 2 h in resting cell experiments (Saito et al. Citation2007). However, it took one day to observe chitinase activity in the soil treated with (GlcNAc)2 (). Enough growth of chitinolytic bacteria would first be required to express detectable chitinase activity. In resting cell experiments of S. coelicolor using chitin, the transcription of chitinase genes is detected after 2 h and the induction of chitinase activity was observed after 2 d (Saito et al. Citation2000, Citation2013). The produced chitinases could bind chitin and are assumed undetectable in the culture supernatant until chitin was hydrolyzed enough (Saito et al. Citation2000). In this study, the induction timing and the level of chitinase activity in the presence of (GlcNAc)2 were faster and lower, respectively, than those by chitin powder (). It is presumed that (GlcNAc)2 was utilized as carbon and nitrogen sources and simultaneously induced the production of chitinase, which could be extracted with water. The produced chitinase might have degraded later.
The populations of Paenibacillus and Streptomycetaceae increased in the (GlcNAc)2-added soil (). A number of strains of Paenibacillus and Streptomycetaceae have been reported to produce chitinases (Saito, Fujii, and Miyashita Citation1999; Veliz, Martínez-Hidalgo, and Hirsh Citation2017; Itoh and Kimoto Citation2019). (GlcNAc)2 is the main chitin-degradation product which is assimilated as carbon source in the chitinolytic bacteria. (GlcNAc)2 induces the expression of chitinase genes in chitinolytic Paenibacillus strains and Streptomyces strains belonging to Streptomycetaceae, but GlcNAc does not (Miyashita, Fujii, and Saito Citation2000; Saito et al. Citation2000; Itoh and Kimoto Citation2019). Paenibacillus and Streptomycetaceae increased in the (GlcNAc)2-treated soil by utilizing (GlcNAc)2 as carbon source and are presumed to have produced chitinase in response to (GlcNAc)2.
In the soil samples supplemented with GlcNAc or (GlcNAc)2, GlcNAcase activity was detected longer than chitinase induced by (GlcNAc)2 (). The data suggest that GlcNAcase activity detected at after 1 d was directly induced by (GlcNAc)2 as have been reported in chitinolytic Streptomyces and Vibrio strains (Li and Roseman Citation2004; Saito et al. Citation2013) and partly by GlcNAc as in a chitinolytic Serratia strain (Toratani et al. Citation2008). The continuous GlcNAcase activity observed after 2–3 d implies the involvement of GlcNAcase in the turnover of soil biomass, especially in the degradation of murein, a component of bacterial cell wall. The turnover of soil biomass was also implied from the changes of the ammonia/ammonium contents during the corresponding duration in the GlcNAc/(GlcNAc)2-treated soil (Figure S1). It has been reported that a high correlation was found between the GlcNAcase activity and the amount of nitrogen mineralized (r = 0.708) (Shindo Citation1992).
Bacillus and Paenibacillus were distinctively increased in the soils supplemented with GlcNAc and (GlcNAc)2, respectively ()). We could not get simple reasons why such distinctive increase occurred, based on the growth experiment using several type strains of Bacillus and Paenibacillus (). We may have to investigate the reproducibility of the modulation of Bacillus and Paenibacillus by GlcNAc and (GlcNAc)2 in soils with different textures, which would possess other bacterial community structures. However, we obtained data suggesting that Bacillus species prefer GlcNAc to (GlcNAc)2 as carbon source. Significant increase of the family Bacillaceae by GlcNAc amendment was reported by Hui et al. (Citation2020), in which some members of Bacillus were speculated to be opportunistic species rather than chitinolytic species. The chitinolytic ability of only one Bacillus strain among the five strains tested in this study () may support this speculation. Paenibacillus sp. FPU-7, which is a potent chitin degrader, grows faster on a minimal medium supplemented with (GlcNAc)2 than on media with glucose or GlcNAc (Kimoto et al. Citation2014). In this report, five among the six tested Paenibacillus species utilized both GlcNAc and (GlcNAc)2 () and they did not show significant difference in the growth rates (data not shown). There may be other Paenibacillus species like FPU-7, that prefer (GlcNAc)2 to GlcNAc. Such Paenibacillus species might have increased in the (GlcNAc)2-treated soil. We have isolated bacterial strains from the soil samples treated with GlcNAc and (GlcNAc)2 to obtain Bacillus and Paenibacillus strains. We hope characterization of GlcNAc/(GlcNAc)2-utilization profiles of the isolates will provide hints to elucidate why Bacillus and Paenibacillus were increased in the soil supplemented with GlcNAc and (GlcNAc)2, respectively.
5. Concluding remarks
(GlcNAc)2, which has been commonly identified as the smallest unit of chitin degradation product that induces chitinase production in chitinolytic bacteria, was consumed in an incubated upland soil within 1 d as GlcNAc was. (GlcNAc)2 induced chitinase activity and significantly increased the populations of the genus Paenibacillus and the family Streptomycetaceae, which are known as potent chitinolytic bacteria. In contrast, GlcNAc did not induce chitinase activity and enlarged the size of the population of the genus Bacillus.
Supplementary_materials_after_poof_reading_200313.docx
Download MS Word (162.2 KB)Acknowledgments
The authors appreciate the technical support provided by Kazuo Hayakawa in the GC/MS analysis. The authors thank Yaizu Suisankagaku Industry for providing N,N’-diacetylchitobiose.
Disclosure statement
No potential conflict of interest was reported by the authors.
Supplementary material
Supplementary data for this article can be accessed here.
Additional information
Funding
References
- Bolyen, E., J. R. Rideout, M. R. Dillon, N. A. Bokulich, C. C. Abnet, G. A. Al-Ghalith, H. Alexander, et al. 2019. “Reproducible, Interactive, Scalable and Extensible Microbiome Data Science Using QIIME 2.” Nature Biotechnology 37 (8): 852–857. doi:10.1038/s41587-019-0209-9.
- Buxton, E. W., O. Khalifa, and V. Ward. 1965. “Effect of Soil Amendment with Chitin on Pea Wilt Caused by Fusarium oxysporum F. Pisi.” Annals of Applied Biology 55 (1): 83–88. doi:10.1111/j.1744-7348.1965.tb07870.x.
- Caporaso, J. G., C. L. Lauber, W. A. Walters, D. Berg-lyons, C. A. Lozupone, P. J. Turnbaugh, N. Fierer, and R. Knight. 2011. “Global Patterns of 16S rRNA Diversity at a Depth of Millions of Sequences per Sample.” Proceedings of the National Academy of Sciences of the United States of America 108: 4516–4522. doi:10.1073/pnas.1000080107.
- Cretoiu, M. S., G. W. Korthals, J. H. M. Visser, and J. D. van Elsas. 2013. “Chitin Amendment Increases Soil Suppressiveness toward Plant Pathogens and Modulates the Actinobacterial and Oxalobacteraceal Communities in an Experimental Agricultural Field.” Applied and Environmental Microbiology 79: 5291–5301. doi:10.1128/AEM.01361-13.
- Deshpande, M. V. 1986. “Enzymatic Degradation of Chitin and Its Biological Applications.” Journal of Scientific & Industrial Research (India) 45: 273–281.
- Gooday, G. W. 1991. “Chitinases.” In Enzymes in Biomass Conversion, edited by G. F. Latham and M. E. Himmel, 478–485. Washington DC: American Chemical Society.
- Henis, Y., B. Sneh, and J. Katan. 1967. “Effect of Organic Amendments on Rhizoctonia and Accompanying Microflora in Soil.” Canadian Journal of Microbiology 13: 643–649. doi:10.1139/m67-085.
- Hui, C., H. Jiang, B. Liu, R. Wei, Y. Zhang, Q. Zhang, Y. Liang, and Y. Zhao. 2020. “Chitin Degradation and the Temporary Response of Bacterial Chitinolytic Communities to Chitin Amendment in Soil under Different Fertilization Regimes.” Science of the Total Environment 705: 136003. doi:10.1016/j.scitotenv.2019.136003.
- Iinuma, C., A. Saito, T. Ohnuma, E. Tnconi, A. Rosu, S. Colson, Y. Mizutani, et al. 2018. “NgcESco Acts as a Lower-Affinity Binding Protein of an ABC Transporter for the Uptake of N,N′-Diacetylchitobiose in Streptomyces coelicolor A3(2).” Microbes and Environments 33 :272–281. doi:10.1264/jsme2.ME17172.
- Itoh, T., and H. Kimoto. 2019. “Bacterial Chitinase System as a Model of Chitin Biodegradation.” Chap. 7 In Targeting Chitin-containing Organisms. In Advances in Experimental Medicine and Biology, edited by Q. Yang and T. Fukamizo, Vol. 1142. 131–151. Singapore: Springer. doi:10.1007/978-981-13-7318-3_7.
- Itoh, T., T. Hibi, Y. Fujii, I. Sugimoto, A. Fujiwara, F. Suzuki, Y. Iwasaki, J. Kim, A. Taketo, and H. Kimoto. 2013. “Cooperative Degradation of Chitin by Extracellular and Cell Surface-expressed Chitinases from Paenibacillus sp. Strain FPU-7.” Applied and Environmental Microbiology 79: 7482–7490. doi:10.1128/AEM.02483-13.
- Iwasaki, Y., T. Ichino, and A. Saito. 2020. “Transition of the Bacterial Community and Culturable Chitinolytic Bacteria in Chitin-treated Upland Soil: From Streptomyces to Methionine-auxotrophic Lysobacter and Other Genera.” Microbes and Environments 35. doi:10.1264/jsme2.ME19070.
- Jacquiod, S., L. Franqueville, S. Cécillon, T. M. Vogel, P. Simonet, and E. Ben-Jacob. 2013. “Soil Bacterial Community Shifts after Chitin Enrichment: An Integrative Metagenomic Approach.” PloS One 8 (11): e79699. doi:10.1371/journal.pone.0079699.
- Khalifa, O. 1965. “Biological Control of Fusarium Wilt of Peas by Organic Soil Amendments.” Annals of Applied Biology 56: 129–137. doi:10.1111/j.1744-7348.1965.tb01222.x.
- Kimoto, H., T. Itoh, T. Hibi, Y. Fujii, and H. Kusaoke. 2014. “Chitin Degradation by Paenibacillus (In Japanese).” Bulletin of Applied Glycoscience 4: 113–120. doi:10.5458/bag.4.2_113.
- Kumeta, Y., K. Inami, K. Ishimaru, Y. Yamazaki, R. Sameshima-Saito, and A. Saito. 2018. “Thermogravimetric Evaluation of Chitin Degradation in Soil: Implication for the Enhancement of Ammonification of Native Organic Nitrogen by Chitin Addition.” Soil Science and Plant Nutrition 64: 512–519. doi:10.1080/00380768.2018.1457408.
- Li, X., and S. Roseman. 2004. “The Chitinolytic Cascade in Vibrios Is Regulated by Chitin Oligosaccharides and a Two Component Chitin Catabolic Sensor/kinase.” Proceedings of the National Academy of Sciences of the United States of America 101: 627–631. doi:10.1073/pnas.0307645100.
- Ludwig, W., O. Strunk, R. Westram, L. Richter, H. Meier, B. A. Yadhukumar, Lai T, Steppi S, Jobb G, Förster W. 2004. “ARB: A Software Environment for Sequence Data.” Nucleic Acids Research 32 :1363–1371. doi:10.1093/nar/gkh293.
- McDonald, D., M. N. Price, J. Goodrich, E. P. Nawrocki, T. Z. DeSantis, A. Probst, G. L. Andersen, R. Knight, and P. Hugenholtz. 2012. “An Improved Greengenes Taxonomy with Explicit Ranks for Ecological and Evolutionary Analyses of Bacteria and Archaea.” The ISME Journal 6: 610–618. doi:10.1038/ismej.2011.139.
- Mendes, R., M. Kruijt, I. Bruijn, E. Dekkers, M. van der Voort, H. H. M. Schneider, Y. M. Piceno, T. Z. DeSantis, G. L. Andersen, and P. A. H. M. Bakker. 2011. “Deciphering the Rhizosphere Microbiome for Disease-suppressive Bacteria.” Science 332: 1097–1100. doi:10.1126/science.1203980.
- Mian, J. H., G. Godoy, R. A. Shelby, R. Rodriguez-Kabana, and G. Morgan-Jones. 1982. “Chitin Amendments for Control of Meloidogyne arenaria in Infested Soil.” Nematropica 12: 71–84.
- Mitchell, R., and M. Alexander. 1962. “Microbiological Processes Associated with the Use of Chitin for Biological Control.” Soil Science Society of America Journal 26: 556–558. doi:10.2136/sssaj1962.03615995002600060013x.
- Miyashita, K., T. Fujii, and A. Saito. 2000. “Induction and Repression of a Streptomyces lividans Chitinase Gene Promoter in Response to Various Carbon Sources.” Bioscience, Biotechnology, and Biochemistry 64: 39–43. doi:10.1271/bbb.64.39.
- Miyashita, K., T. Fujii, and Y. Sawada. 1991. “Cloning and Characterization of Chitinase Genes from Streptomyces lividans 66”. Journal of General Microbiology 137: 2065–2074. doi:10.1099/00221287-137-9-2065.
- Murakami, H., Y. Kuroyanagi, S. Tsushima, and Y. Shishido. 2009. “Effects of Application of Organic Materials on the Development of Clubroot Disease Caused by Plasmodiophora brassicae (In Japanese).” Soil Microorganisms 63: 3–8.
- Muzzarelli, R. A. 1977. Chitin. New York: Pergamon Press.
- Saito, A., H. Ebise, Y. Orihara, S. Murakami, Y. Sano, A. Kimura, Y. Sugiyama, A. Ando, T. Fujii, and K. Miyashita. 2013. “Enzymatic and Genetic Characterization of the DasD Protein Possessing N-acetyl-β-D-glucosaminidase Activity in Streptomyces coelicolor A3(2).” FEMS Microbiology Letters 340: 33–40. doi:10.1111/1574-6968.12069.
- Saito, A., M. Ishizaka, P. B. Francisco Jr., T. Fujii, and K. Miyashita. 2000. “Transcriptional Co-regulation of Five Chitinase Genes Scattered on the Streptomyces coelicolor A3(2) Chromosome.” Microbiology 146: 2937–2946. doi:10.1099/00221287-146-11-2937.
- Saito, A., T. Fujii, and K. Miyashita. 1999. “Chitinase System in Streptomyces.” Actinomycetologica 13: 1–10. doi:10.3209/saj.13_1.
- Saito, A., T. Shinya, K. Miyamoto, T. Yokoyama, H. Kaku, E. Minami, N. Shibuya, et al. 2007. “The dasABC Gene Cluster, Adjacent to dasR, Encodes a Novel ABC Transporter for the Uptake of N, N’-diacetylchitobiose in Streptomyces coelicolor A3(2).” Applied and Environmental Microbiology 73: 3000–3008. doi:10.1128/AEM.02612-06.
- Sarathchandra, S. U., R. N. Watson, N. R. Cox, M. E. Di Menna, J. A. Brown, G. Burch, and F. J. Neville. 1996. “Effects of Chitin Amendment of Soil on Microorganisms, Nematodes, and Growth of White Clover (Trifolium repens L.) And Perennial Ryegrass (Lolium Perenne L.).” Biology and Fertility of Soils 22: 221–226. doi:10.1007/BF00382516.
- Shindo, H. 1992. “Effect of Continuous Compost Application on the Activities of Protease, β-acetylglucosaminidase, and Adenosine Deaminase in Soils of Upland Fields and Relationships between the Enzyme Activities and the Mineralization of Organic Nitrogen.” Japan Journal of Soil Science and Plant Nutrition 63: 190–195. in Japanese. doi:10.20710/dojo.63.2_190.
- Sneh, B., J. Katan, and Y. Henis. 1971. “Mode of Inhibition of Rhizoctonia solani in Chitin Amended Soil.” Phytopathology 61: 1113–1117. doi:10.1094/Phyto-61-1113.
- Sneh, B., and Y. Henis. 1972. “Production of Antifungal Substances Active against Rhizoctonia solani in Chitin-amended Soil.” Phytopathology 62: 595–600. doi:10.1094/Phyto-62-595.
- Takada-Hoshino, Y., and N. Matsumoto. 2004. “An Improved DNA Extraction Method Using Skim Milk from Soils that Strongly Adsorb DNA.” Microbes and Environments 19: 13–19. doi:10.1264/jsme2.19.13.
- Toratani, T., T. Shoji, T. Ikehara, K. Suzuki, and T. Watanabe. 2008. “The Importance of Chitobiase and N-acetylglucosamine (GlcNAc) Uptake in N,N’-diacetylchitobiose [(GlcNAc)2] Utilization by Settatia marcescens 2170.” Microbiology 154: 1326–1332. doi:10.1099/mic.0.2007/016246-0.
- Uchiyama, T., R. Kaneko, J. Yamaguchi, A. Inoue, T. Yanagida, N. Nikaidou, M. Regue, and T. Watanabe. 2003. “Uptake of N,N’-diacetylchitobiose [(GlcNAc)2] via the Phosphotransferase System Is Essential for Chitinase Production by Serratia marcescens 2170.” Journal of Bacteriology 185: 1776–1782. doi:10.1128/jb.185.6.1776-1782.2003.
- Van Eck, W. H. 1978. “Autolysis of Chlamydospores of Fusarium solani F. Sp. Cucurbitae in Chitin and Laminarin Amended Soils.” Soil Biology and Biochemistry 10: 89–92. doi:10.1016/0038-0717(78)90076-7.
- Veliz, E. A., P. M. Martínez-Hidalgo, and A. M. Hirsh. 2017. “Chitinase-producing Bacteria and Their Role in Biocontrol.” AIMS Microbiology 3: 689–705. doi:10.3934/microbiol.2017.3.689.
- Weller, D. M., J. M. Raaijmakers, B. B. McSpadden, and L. S. Thomashow. 2002. “Microbial Populations Responsible for Specific Soil Suppressiveness to Plant Pathogen.” Annual Review of Phytopathology 40: 309–348. doi:10.1146/annurev.phyto.40.030402.110010.
- Wieczorek, A. S., S. A. Hetz, and S. Kolb. 2014. “Microbial Responses to Chitin and Chitosan in Oxic and Anoxic Agricultural Soil Slurries.” Biogeosciences 11: 3339–3352. doi:10.5194/bg-11-3339-2014.