ABSTRACT
The soil map of Madagascar has not been substantially updated since the 1960s. The number of reported soil profiles that meet the criteria of the Reference Pedon Description – the highest level of data reliability used to ascertain FAO soil unit classification – is also limited for a country-scale soil map. Therefore, we conducted a soil survey in the east coast and the central highlands, the most populated and major food-producing areas in Madagascar, to assess need for reconsideration of the current soil map. As compared to the latest soil map, our results indicate that Ferralsols cover less area on the flat terrains from the east coast to the central highlands and that Geric Ferralsol is more frequently observed soil type rather than Haplic Ferralsol on acidic rocks. The soil type of paddy fields in the southwestern plain of Lake Alaotra – the country’s largest rice-producing region – was Vertisol with a hydragric horizon rather than Histosol. This is attributed to long-term paddy cultivation in the region or the difference of the definition of peat soils between the current and old soil classification systems. The volcanic-origin soils that sporadically exist in the central highlands can be classified as Eutric Andosol rather than Eutric Cambisol. These results show the need for updating the current soil map based on the quantitative data and also have important implications regarding the land history and for appropriate land management in agriculturally intensive areas in the east coast and central highlands of Madagascar.
1. Introduction
Soil maps based on quality-assessed soil data and interpreted soil information provide fundamental information for appropriate agricultural management. The latest soil map of Madagascar – the Soil Atlas of Africa (EU Citation2013) – was developed based on the Soil Map of the World (FAO-UNESCO Citation1977) with little modification (). In addition, the Madagascar part of the Soil Map of the World was created from information in an atlas (Roederer and Bourgeat Citation1969) and a pedological map (Riquier Citation1968) of Madagascar. Therefore, the latest soil map of Madagascar basically relies on an old French soil classification system used prior to the Commission de Pédologie et de Cartographie des Sols (CPCS) (Citation1967), and there has been no significant update since the 1960s. In addition, Batjes (Citation2008) reported that only 20 of 54 soil profiles in Madagascar in Version 3.1 of the ISRIC-WISE database meet the Reference Pedon Description criteria, which is the highest data reliability level used to ascertain FAO soil unit classification. This amount is clearly insufficient to update a country-scale soil map of Madagascar, particularly with its diverse environment. Thus, collecting new soil data and classifying the soils according to the latest soil classification system based on those data would be an important first step in updating the soil map in this country.
Figure 1. Changes in the soil map of Madagascar: (a) EU (Citation2013), (b) FAO-UNESCO (Citation1977) digitalized in FAO (Citation1991), and (c) Riquier (Citation1968). Only soils mentioned in the main text are shown. A rectangle in (a) represents the area shown in
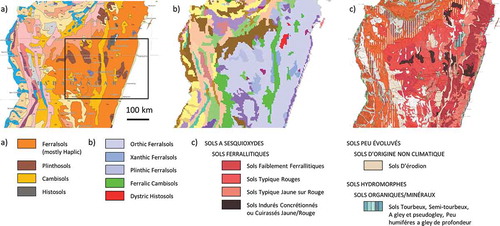
The east coast and the central highlands of Madagascar are characterized as the most populated and major food-producing area of the country. According to the Köppen climate classification system, the climate greatly varies from tropical with high temperatures and precipitation (Af) to temperate with a dry winter and hot-warm summer (Cwa, Cwb) (). Regardless of the various climatic conditions, Haplic Ferralsols (FR-ha) are reported to be predominant on acidic rocks widely distributed from the east coast to the central highlands (EU Citation2013). Haplic Ferralsols are characterized as soils rich in kaolinite and oxides and are generally considered unfertile. Because the classification of soils as FR-ha is mainly based on information in the old atlas (Roederer and Bourgeat Citation1969) and pedological map (Riquier Citation1968), there is a considerable doubt whether these classifications are currently applicable.
Figure 2. Mean annual rainfall (a), mean annual temperature (b) and topographic cross section along the line AB (c). Maps were created using the WorldClim 2 dataset (Fick and Hijmans Citation2017). Topographic data were derived from GTOPO30 (U.S. Geological Survey EROS Data Center Citation1997).
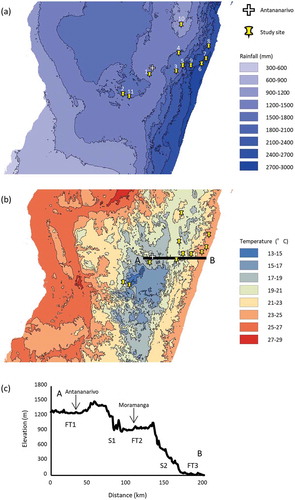
Lake Alaotra and its surrounding wetlands are the most intensive rice-producing area in Madagascar. The fertile sedimentary soils in the vast southwestern plain of Lake Alaotra have been utilized as paddy fields with irrigation canals and a constant water supply from Lake Alaotra. According to the latest soil map (EU Citation2013), the lake’s surrounding wetlands are covered by Dystric Histosols (HS-dy), soils with thick organic layers and a low pH. Although this soil type may be correct for the marsh and reed bed, it is doubtful that HS-dy is the correct soil type for the paddy fields that have been cultivated for many years considering the inadequate characteristics of HS-dy for agricultural practices.
The high mountains that sporadically appear in the central highlands of Madagascar are mostly of volcanic origin, whereas the rest of the highlands are essentially formed by schists, migmatites, and gneiss of the basement complex. The Quaternary volcanic fields overlie Precambrian basement rocks in the central highlands of Madagascar (Rufer et al. Citation2014), and the soils of volcanic origin can have substantially different properties and higher crop productivity as compared with the strongly weathered unfertile soils surrounding them (Nishigaki et al. Citation2018). Therefore, the areas with productive soils should be clearly characterized. Currently, however, volcanic soils in the central highlands are classified as Eutric Cambisols (CA-eu) (EU Citation2013), which offers little information for appropriate land management, particularly for volcanic soils with a high phosphate fixation.
Given the aforementioned obsolete and incomplete nature of the soil database in Madagascar, we established the following objectives in this study: (1) to increase the number of soil profiles meeting the criteria of Reference Pedon Description in Madagascar, (2) to validate the distribution of FR-ha from the east coast to the central highlands, and (3) to obtain representative soil profile information in areas with intensive agriculture in the central highlands to assess need for reconsideration of the current soil types (HS-dy in Lake Alaotra and CA-eu in volcanic soils) in terms of agricultural management.
2. Materials and methods
2.1. General description of the east coast and central highlands
The east coast and central highlands of Madagascar consist of three flat terrains and two slopes (). The upper flat terrain (FT1) is a constituent of the central highlands and has an altitude between 1200 and 1500 m a.s.l. (data from U.S. Geological Survey EROS Data Center (Citation1997)), a mean annual temperature of 13–19ºC, and a mean annual rainfall of 1200–1800 mm (data from Fick and Hijmans (Citation2017)). On the east, FT1 ends in an escarpment (S1) connecting FT1 and the middle flat terrain (FT2). The FT2 is also a constituent of the central highlands and has an altitude between 600 and 900 m a.s.l., a mean annual temperature of 19–21ºC, and a mean annual rainfall of 1500–2400 mm. Slope 2 (S2) is relatively gentle and connects FT2 and the lower flat terrain (FT3). The FT3 is a coastal plain and a constituent of the east coast. It is rarely more than 30 km wide and has an altitude of less than 100 m a.s.l., a mean annual temperature of 23–25ºC, and a mean annual rainfall of 2400–3000 mm. According to the current soil map (EU Citation2013) and geological map (Schlüter Citation2008), a predominant soil type on acidic rocks on FT1–3 is FR-ha (), and the dominant soils on basic rocks (i.e., the greenstone belt) in S2 are Plinthosols, while Cambisols are also found on S1 and S2 between flat terrains.
To estimate the slope distribution within a rectangle of the surveyed area in (top, 17°45’S; bottom, 20°05’S; left, 46°48ʹE; right, 49°57ʹE; totally 75,610 km2), ALOS World 3D-30 m images (JAXA Citation2018) were used. The slope for each pixel having a resolution of 32 m was calculated by slope function of a GIS software (ArcGIS version 10.4, ESRI).
2.2. Soil survey and physicochemical analysis
Ten soil pits were made to obtain a detailed description of the soil profile and to collect soil samples (, and A, excluding ID 4). Each pit was 1 m × 1 m in area and generally up to about 1.2 m deep. The locations were carefully determined from the viewpoint of regional representativeness using geological maps (Basairie Citation1968; Riquier Citation1968; Schlüter Citation2008), soil maps (Riquier Citation1968; EU Citation2013), and satellite images (e.g., Google Earth) (). The specific location was selected based on field observation such that each pit was on a summit or ridge to the extent possible to avoid the effect of recent colluvial deposits. Eight pits (; IDs 1–8) were targeted in areas with FR-ha on FT1–3, which is the predominant soil type in this area based on the latest soil map (EU Citation2013). Due to bad road conditions caused by a landslide, we could not reach the selected location for one soil pit (ID 4), and therefore no sample was obtained for ID 4. A soil pit (ID 9) was targeted on the greenstone belt on S2 where Plinthosols were expected according to EU (Citation2013). One pit (ID 10) was made in the intensive paddy fields in the south-western plain of Lake Alaotra on FT2, and another (ID 11) was made in a cropland in Betafo where volcanic soils are distributed on FT1. In EU (Citation2013), soil types in these agricultural areas were HS-dy and CA-eu, respectively. Soil profiles in the pits were described according to terms in FAO (Citation2006). Soil samples were taken from each horizon using a trowel, air-dried, passed through a 2-mm sieve, and stored at a room temperature until subsequent physical and chemical analyses in the laboratory. Cylinder core samples were also taken for measuring bulk density.
Table 1. List of candidate sites for soil survey.
Figure 3. Location of soil pits on (a) soil map (EU Citation2013) and (b) slope map.
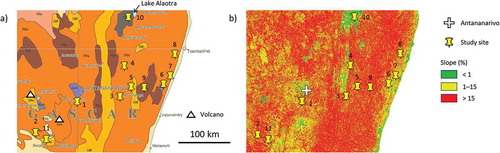
Soil samples were mixed in a soil to solution (H2O and 1 M KCl) ratio of 1:5, and the pH was measured with a pH electrode (LAQUA F-72, HORIBA) after being shaken for 2 h. Total carbon and total nitrogen were determined using the dry combustion method with an elemental analyzer (SUMIGRAPH NC-220F, Sumika Chemical Analysis Service). Exchangeable bases, exchangeable acidity, and cation exchangeable capacity (CEC) were generally measured according to van Reeuwijk (Citation2002), but inductively coupled plasma atomic emission spectroscopy (ICP-AES) with a spectrometer (ICPE-9000, SHIMADZU) was used in this study. Effective base saturation (EBS), as defined by the IUSS Working Group WRB (Citation2015), was calculated by dividing exchangeable (Ca2+ + Mg2+ + K+ + Na+) by exchangeable (Ca2+ + Mg2+ + K+ + Na+ + Al3+). Available phosphorus was determined by the Bray-I method (Bray and Kurtz Citation1945; van Reeuwijk Citation2002) using a Shimadzu UV-1800 spectrophotometer. Particle size distribution was measured as described by Gee and Bauder (Citation1986). After chemical degradation of organic matter by H2O2 and sample dispersion with sodium hexametaphosphate, coarse- and fine-sand fractions (0.2–2 and 0.02–0.2 mm, respectively) were measured by sieving, and silt (0.002–0.02 mm) and clay (<0.002 mm) fractions were measured with the pipette method. Bulk density was determined by measuring the masses of 100-ml core samples after drying at 105°C for 48 h. To test the diagnostic criteria of Andic properties, phosphorus retention (Soil Survey Staff Citation2014a) and oxalate-extractable Al and Fe (Courchesne and Turmel Citation2008) were measured for soils from ID 11.
Based on soil profile descriptions and soil physical and chemical data, soil profiles were classified to the level of principal qualifiers using the current World Reference Base (WRB) system (IUSS Working Group WRB Citation2015) as well as to the level of subgroups using Keys to Soil Taxonomy (Soil Survey Staff Citation2014b).
3. Results and discussion
3.1. Distribution of Ferralsols and Plinthosols
Among IDs 1–8 targeted in areas with FR-ha on FT1–3, Ferralsols were not found on steep land (> 15% slope) (; IDs 3, 7). Rather, soils in steep land on FT1–3 (IDs 3, 7) were classified as Cambisols due to the lack of clay accumulation and a ferralic horizon ( and ), which is similar to the distribution of Cambisols on S1 and a part of S2 (EU Citation2013). Since the area with steep slope (> 15%) was estimated to cover 62% of the whole surveyed area (; the average slope was 21%), it was suggested that Ferralsols cover less area than reported by EU (Citation2013). Even on flat to sloping land (< 15% slope) on FT1–3 (), we found both Ferralsols (IDs 1, 6, 8) and Lixisols (IDs 2, 5), the latter of which has an argic horizon but not a ferralic horizon. According to the legacy soil data of Africa Soil Profiles Database (Leenaars, van Oostrum, and Ruiperez Gonzalez Citation2014), the 20 soil profiles with reliability level 1 in Madagascar (same as 20 profiles in Version 3.1 of the ISRIC-WISE database meeting the Reference Pedon Description criteria) were located within the area of 1,130 km2 covered mainly by steep slope (74% of total area) on FT2. Although those 20 soil profiles were expected to be FR-ha (EU Citation2013), only three profiles were classified as Ferralsols, while the most of others were classified as Cambisols or Fluvisols. This together with our results indicates that, due to the extensive steep land, Ferralsols cover less area on FT1–3 than reported in EU (Citation2013); Cambisols could be more common than Ferralsols.
Table 2. Soil classification and physical properties.
Table 3. Soil chemical properties.
The area covered by FR-ha in EU (Citation2013) basically corresponds to the range of ‘Sols Ferrallitiques’ in Roederer and Bourgeat (Citation1969) and Riquier (Citation1968), both of which followed the old French classification system (Aubert Citation1965). It is worth noting that neither Aubert (Citation1965) nor CPCS (Citation1967) used the concept of a diagnostic horizon, which has been adopted in many current soil classification systems (e.g., Obara et al. Citation2011; Soil Survey Staff Citation2014b; IUSS Working Group WRB Citation2015). Moreover, the criteria for ‘Sols Ferrallitiques’ have no critical value for thickness and soil texture (Aubert Citation1965). Latham (Citation1981) noted the absence of precise diagnostic features as a problem of the old French classification systems, and that sometimes too much was left open to personal interpretation. In contrast, the ferralic horizon is used as a diagnostic horizon for Ferralsols and has more specific criteria, for example, certain values must be met for soil texture class, CEC, and exchangeable cations (Ca2+ + Mg2+ + K+ + Na+ + Al3+) (IUSS Working Group WRB Citation2015). Our results indicate that relying on the old classification system resulted in an overestimation of FR-ha in the targeted region in EU (Citation2013).
In addition, Ferralsols (IDs 1, 6, 8) generally have geric properties, i.e., low effective CEC (sum of exchangeable cations) and/or high anion exchangeable capacity (pHKCl -pHwater ≧ +0.1) (), and thus Geric Ferralsols (FR-gr) should be predominant in the targeted region rather than FR-ha. It is difficult to interpret the reason of this mismatch because we have no means of knowing how ‘Sols Faiblement Ferrallitiques,’ ‘Sols Typique Rouges,’ and ‘Sols Typique Jaune sur Rouge’ in ‘Sols Ferrallitiques’ were translated into Orthic Ferralsols in FAO-UNESCO (Citation1977) and then FR-ha in EU (Citation2013). It could be due to the lack of enough soil data. Note that Geric properties were not used in FAO-UNESCO (Citation1977) and were firstly introduced in Revised Legends (FAO Citation1988), which could have influenced the mismatch.
Translation of a soil order in WRB system to a similar soil order in Soil Taxonomy and vice versa is a simple method often applied to make a soil map. Ferralsols are considered to overlap with Oxisols – highly weathered tropical soils with low natural fertility in Soil Taxonomy classification system (Soil Survey Staff Citation2014b). It’s worth noting that, however, Oxisols were rarely found in the targeted region. This is attributed to the stricter condition of the oxic horizon than the ferralic horizon: an oxic horizon requires < 5% of rock structure and a clay increase with increasing depth, whereas a ferralic horizon accepts < 80% coarse fragment and a clay decrease with increasing depth like IDs 1 and 8. This difference has caused the mismatch of Ferralsols and Oxisols in the targeted region.
In the former version of the WRB system (IUSS Working Group WRB Citation2006), Lixisols for IDs 2 and 5 were classified as Acrisols, but the diagnostic criteria to key out Acrisols was changed in the latest WRB system (IUSS Working Group WRB Citation2015). Base saturation defined as exchangeable (Ca2+ + Mg2+ + K+ + Na+) divided by CEC (by 1 M NH4OAc) was used in the former system, whereas effective base saturation defined as exchangeable (Ca2+ + Mg2+ + K+ + Na+) divided by exchangeable (Ca2+ + Mg2+ + K+ + Na+ + Al3+) is used in the latest system. Owing to this modification, the soil profiles with low exchangeable Al3+ tend to be classified as Lixisols. This is in contrast to that Ferralsols used to be considered to occur alongside of Acrisols on acidic rocks (FAO Citation2001) because the principal difference between those two soil types was just the presence or absence of clay accumulation. Therefore, the introduction of effective base saturation needs special attention when we describe soil maps of tropical area.
A soil profile (ID 9) located on basic rocks (amphibole migmatite) was classified as Geric Plinthosol (PT-gr); the profile had a plinthic horizon, but not a pisoplinthic horizon. This is basically in agreement with the soil type previously reported as ‘Sols induré’ (indurated soils) (Riquier Citation1968) and Pisoplinthic Plinthosol (PT-px) in EU (Citation2013), although the principal qualifier was different (). The cover range of PT-gr is expected to follow that of PT-px in the latest soil map.
It is worth noting that, because of the large gap in soil texture, color, or content of rock fragment between a pair of adjacent layers within a soil profile, some pedons (IDs 1, 5, 7) can be considered as not being evolved from a base parent material but developed from different parent materials; that is, there are base materials and overlying redeposited materials. This can lead to classifying these pedons as Cambisols due to the absence of a ferralic horizon in overlying soils (IDs 1, 5, 7; –). Consequently, Ferralsols were rarely found in the central highlands, and Cambisols were the predominant soil type. Roederer and Bourgeat (Citation1969) noted that the pronounced relief of Madagascar has led to intense soil erosion and stripping of old soil formations. Therefore, there might be various soils that recently developed from the base parent materials or from the overlying redeposited materials. More work is required to reveal the extent to which soils developed from secondary materials existing in the east coast and central highlands of Madagascar.
Table 4. Description of soil profiles.
3.2. Paddy soils around Lake Alaotra and sporadic volcanic soils in the central highlands
Riquier (Citation1968) classified the soil type of southwestern plain of Lake Alaotra as ‘Sols Hydromorphes, Sols Organiques/Minéraux’ (hydromorphic, organic/mineral soils), and this was translated into Histosols in the latest soil map (EU Citation2013). Irrigated rice cultivation in this area doubled between 1959 and 1989, and about 820 km2 of irrigated paddy fields currently cover the Alaotra topographic basin, or about 45% of the total basin (Mietton et al. Citation2018). The soil profile observed in the southwestern plain of Lake Alaotra (ID 10) was classified as Hydragric Vertisol (VR-hg), which had high nutritional status in the top three layers presumably due to constant sedimentation through irrigation water ( and ). In addition, there were redoximorphic features in the top two layers and Fe and Mn accumulation in the underlying horizon, suggesting the effect of irrigated rice cultivation ( and ). The combined thickness of anthraquic horizon and hydragric horizon can vary depending on the distance from canals and farmers’ cultivation practice. Therefore, other places within the paddy fields in southwestern plain of Lake Alaotra could be classified as Anthrosols which can be keyed out prior to Vertisols if the combined thickness of anthraquic horizon and an underlying hydragric horizon is > 50 cm.
Table 5. Description of soil profiles.
It is indicated that long-term paddy-rice cultivation (> 50 years) associated with a drainage system generated redoximorphic features within soil profiles ( and ) and accelerated the oxidation of the organic layer of Histosols, thereby changing Histosols into Vertisols. The previous studies also reported the changes in soil order by the rapid oxidation of the organic layer. Veenstra and Burras (Citation2012) showed that 32% of the pedons originally classified as ‘Black soils’ (Phaeozem and Chernozem) (IUSS Working Group WRB Citation2006) in Iowa, United States, have changed enough to result in reclassification at the WRB Reference Group level, mainly because of degradation of organic mollic horizons after more than 50 years of cultivation. Everett (Citation1983) suggested that a few decades of oxidation after the drainage can make the thickness or organic matter content in the organic layer insufficient for the soil to meet the requirements for the order of Histosols. Kempen et al. (Citation2009) reported that accelerated oxidation due to the intensive tillage and lowering of groundwater levels largely changed peat soils into other soil groups in North-East Netherlands. We should also mention that the old soil classification system (Aubert Citation1965) can classify the soils having much less than 20% of soil organic carbon, which are not classified as Histosols in the current WRB system, as “Sols Hydromorphes.“ The difference of the definition of peat soils between old and current soil classification systems could also be attributed to the change of soil type.
One soil profile (ID 11) was classified as Andosol, and it had a layer with andic properties (). This result is in contrast to the latest soil map (EU Citation2013), which reported CA-eu in this area, but was similar to Riquier (Citation1968), who classified the soils in this area as ‘Sols bruns eutrophes peu évolués cendres volcanique’ (little-evolved eutrophic brown soils on volcanic ash). Therefore, future soil survey should focus on investigating to what extent the area of the ‘Sols bruns eutrophes peu évolués cendres volcanique’ in Betafo can be translated as Andosols in the current classification system. This update at the WRB Reference Group level would lead us to expect that the soils in this area have favorable properties for cultivation, such as a high water-holding capacity and organic matter content, but also unfavorable properties, such as a high phosphorus fixing capacity caused by active Al and Fe. These findings on the paddy soils around Lake Alaotra and sporadic volcanic soils in the central highlands have important implications about the land history and for appropriate land management in the agriculturally intensive areas in the central highlands of Madagascar.
Table 6. Oxalate-extractable Al and Fe and P retention at ID 11.
4. Conclusions
There was a considerable gap between our results and the latest country-scale soil map of Madagascar, which was put together from an atlas and pedological map published more than 50 years ago. The current soil classification systems have more distinct diagnostic criteria, and intensive cultivation has changed soil conditions in some areas. Clearly, there is a need to reconsider the country-scale soil map of Madagascar. Further soil survey toward updating soil map in Madagascar should focus on how to link the topographical information and distribution of Ferralsols and accumulation of soil profile information of overlooked soil types in the current soil map to determine their occurrence.
Supplemental Material
Download Zip (5.3 MB)Acknowledgments
The authors would like to thank the Ministry of Mines and Strategic Resources of Madagascar, regional officers, and farmers for their kind support for our soil survey. The authors would also like to thank Dr. Makoto Shibata for his valuable comments on data of the soil profiles. The soil samples were legally imported to Japan for soil analysis with permission of 30Y1175.
Disclosure statement
The authors report no conflicts of interest.
Supplemental Material
Supplemental data for this article can be accessed here.
Additional information
Funding
References
- Aubert, G. 1965. “La classification pedologique utilisée en France.” Pédologie (Grand.) Symposium International, 3. Classification des Sols, 25–26. http://horizon.documentation.ird.fr/exl-doc/pleins_textes/pleins_textes_5/b_fdi_10-11/12533.pdf
- Basairie, H. 1968. “Carte géologique 1/500000.” Madagascar: Service Geologique.
- Batjes, N. H. 2008. “ISRIC-WISE Harmonized Global Soil Profile Dataset (Ver. 3.1).” Report 2008/02. Wageningen: ISRIC – World Soil Information.
- Bray, R. H., and L. T. Kurtz. 1945. “Determination of Total, Organic, and Available Forms of Phosphorus in Soils.” Soil Science 59: 39–45. doi:10.1097/00010694-194501000-00006.
- Courchesne, F., and M. C. Turmel. 2008. “Extractable Al, Fe, Mn, and Si.” In Soil Sampling and Methods of Analysis, edited by M. R. Carter and E. G. Gregorich, 307–315. 2nd ed. Boca Raton, FL: Canadian Society of Soil Science, CRC Press.
- CPCS. 1967. “Classification des Sols.” Ecole Nationale Supérieure d’Agronomie, Grignon. http://library.wur.nl/isric/fulltext/isricu_i33696_001.pdf
- EU. 2013. Soil Atlas of Africa. Edited byA. Jones, H. Breuning-Madsen, M. Brossard, A. Dampha, J. Deckers, O. Dewitte, T. Gallali et al. Luxembourg: European Commission, Publications Office of the European Union.
- Everett, K. R. 1983. “Histosols.” In Pedogenesis and Soil Taxonomy: II. The Soil Orders, edited by L. P. Wilding, N. E. Smeck, and G. F. Hall, 1–53. Amsterdam: Elsevier Science Publishers.
- FAO. 1988. “FAO-UNESCO Soil Map of the World Revised Legend.” Rome: FAO.
- FAO. 1991. “Digital Soil Map of the World, VI Africa.” http://www.fao.org/fileadmin/user_upload/soils/docs/Soil_map_FAOUNESCO/acrobat/Africa_VI.pdf
- FAO. 2001. “Lecture Notes on the Major Soil.” http://www.fao.org/3/a-y1899e.pdf
- FAO. 2006. Guidelines for Soil Description. 4th ed. Rome: FAO and UN.
- FAO-UNESCO. 1977. “Soil Map of the World, 1:5000000.” Volume VI Africa. Paris: Unesco.
- Fick, S. E., and R. J. Hijmans. 2017. “Worldclim 2: New 1-km Spatial Resolution Climate Surfaces for Global Land Areas.” International Journal of Climatology 37: 4302–4315. doi:10.1002/joc.5086.
- Gee, G. W., and J. W. Bauder. 1986. “Particle-Size Analysis.” In Methods of Soil Analysis: Part 1. Physical and Mineralogical Methods, edited by A. Klute, 383–411. Madison, WI: ASA-SSSA publisher.
- IUSS Working Group WRB. 2006. “World Reference Base for Soil Resources 2006.” World Soil Resources Reports No. 103. Rome: FAO.
- IUSS Working Group WRB. 2015. “World Reference Base for Soil Resources 2014, Update 2015, International Soil Classification System for Naming Soils and Creating Legends for Soil Maps.” World Soil Resources Reports No. 106. Rome: FAO.
- JAXA. 2018. “ALOS Global Digital Surface Model “ALOS World 3D - 30m (AW3D30)” Version 2.1.” Accessed 8 January 2020. https://www.eorc.jaxa.jp/ALOS/en/aw3d30/index.htm
- Kempen, B., D. J. Brus, G. B. M. Heuvelink, and J. J. Stoorvogel. 2009. “Updating the 1:50,000 Dutch Soil Map Using Legacy Soil Data: A Multinomial Logistic Regression Approach.” Geoderma 151: 311–326. doi:10.1016/j.geoderma.2009.04.023.
- Latham, M. 1981. “French Soil Classifications and Their Application in the South Pacific Islands.” In Proceedings of the South Pacific Regional Forum on Soil Taxonomy, edited by R. J. Morrison and D. M. Leslie, 185–199. Suva, Fiji: Institute of Natural Resources, The university of the South Pacific.
- Leenaars, J. G. B., A. J. M. van Oostrum, and M. Ruiperez Gonzalez. 2014. “Africa Soil Profiles Database, Version 1.2. A Compilation of Georeferenced and Standardised Legacy Soil Profile Data for Sub-Saharan Africa (With Dataset).” ISRIC Report 2014/01. Wageningen: Africa Soil Information Service (AfSIS) project and ISRIC - World Soil Information.
- Mietton, M., Y. Gunnell, G. Nicoud, L. Ferry, R. Razafimahefa, and P. Grandjean. 2018. “‘Lake’ Alaotra, Madagascar: A Late Quanternary Wetland Regulated by the Tectonic Regime.” Catena 165: 22–41. doi:10.1016/j.catena.2018.01.021.
- Nishigaki, T., S. Sugihara, K. Kobayashi, Y. Hashimoto, M. Kilasara, H. Tanaka, T. Watanabe, and S. Funakawa. 2018. “Fractionation of Phosphorus in Soils with Different Geological and Soil Physicochemical Properties in Southern Tanzania.” Soil Science and Plant Nutrition 64: 291–299. doi:10.1080/00380768.2018.1436406.
- Obara, H., T. Ohkura, Y. Takata, K. Kohyama, Y. Maejima, and T. Hamazaki. 2011. “Comprehensive Soil Classification System of Japan-First Approximation.” Bulletin of National Institute for Agro-Environmental Sciences 29: 1–73.
- Riquier, J. 1968. “Carte pédologique de Madagascar, 1/1000000.” Tananarive: ORSTOM.
- Roederer, P., and F. Bourgeat. 1969. “Planche 16 Pedologie.” Atlas de Madagascar. Tananarive: ORSTOM.
- Rufer, D., F. Preusser, G. Schreurs, E. Gnos, and A. Berger. 2014. “Late Quaternary History of the Vakinankaratra Volcanic Field (Central Madagascar): Insights from Luminescence Dating of Phreatomagmatic Eruption Deposits.” Bulletin of Volcanology 76: 817. doi:10.1007/s00445-014-0817-7.
- Schlüter, T. 2008. Geological Atlas of Africa. With Notes on Stratigraphy, Tectonics, Economic Geology, Geohazards, Geosites and Geoscientific Education of Each Country. 2nd four-coloured revised and enlarged edition. Berlin Heidelberg: Springer Verlag.
- Soil Survey Staff. 2014a. “Kellogg Soil Survey Laboratory Methods Manual.” Soil Survey Investigations Report No. 42, Version 5.0, Edited by Burt R. and Soil Survey Staff, U.S. Department of Agriculture, Natural Resources Conservation Service.
- Soil Survey Staff. 2014b. Keys to Soil Taxonomy. 12th ed. U.S. Department of Agriculture, Natural Resources Conservation Service, Washington, DC.
- U.S. Geological Survey EROS Data Center. 1997. “USGS 30 ARC-second Global Elevation Data, GTOPO30.” Research Data Archive at the National Center for Atmospheric Research, Computational and Information Systems Laboratory. doi:10.5065/A1Z4-EE71.
- van Reeuwijk, L. P. 2002. “Procedures for Soil Analysis.” Technical Paper, No. 9. Wgeningen: ISRIC. http://www.isric.org/sites/default/files/ISRIC_TechPap09.pdf
- Veenstra, J. J., and C. L. Burras. 2012. “Effects of Agriculture on the Classification of Black Soils in the Midwestern United States.” Canadian Journal of Soil Science 92: 403–411. doi:10.4141/CJSS2010-018.