ABSTRACT
The 2030 Agenda was promulgated for the Sustainable Develop Goals (SDGs), which consist of 17 goals created to eradicate poverty and realize a more sustainable world. Among the goals, soil science can contribute significantly toward achieving zero hunger (#2), implementing climate action (#13), bettering life on land (#15), among others. Paddy fields are important sustainable agro-ecosystems for rice (Oryza sativa) production, and rice is the staple food for more than 2 billion people worldwide. Paddy rice is produced mainly in East, South, and Southeast Asia, in region with a monsoon climate. Paddy soils are unique, as they are mostly managed under waterlogged conditions during the rice-growing season. Nitrogen (N) is one of the most important nutrients for many crops, including rice. Some potential sustainable soil management methods are suggested to meet the SDGs, especially in paddy fields and wetlands in East, South, and Southeast Asia, based on current achievements, including N mineralization analysis, soil microbial analysis, and greenhouse gas measurements, to predict soil fertility and effective environmental management practices in the future. One such example of sustainable soil management practice is the amendment of silicate fertilizer produced from steel-making sludge that reduced methane emission from paddy fields (3%–50%) and increased the rice yield in Vietnam.
The United Nations Sustainable Development Summit was held in September 2015 to promulgate the 2030 Agenda for Sustainable Development (the 2030 Agenda), a set of international development goals from 2016 to 2030 (‘Sustainable Development Goals’; SDGs). The 2030 Agenda listed the SDGs, which consist of 17 goals and 169 targets that need to be met to eradicate poverty and realize a more sustainable world (UN Citation2015). Across several goals and targets, soil science can contribute widely to achieving many of them, including achieving zero hunger (#2), implementing climate action (#13), bettering life on land (#15), among others. In this review, potential sustainable soil management methods to achieve SDGs, especially in the paddy fields and wetlands of East, South, and Southeast Asia, are suggested based on current knowledge and future prospects.
1. Nitrogen mineralization and soil fertility
Paddy fields are important sustainable agro-ecosystems used for rice production, and rice is the staple food for more than 2 billion people worldwide. Paddy rice production is practiced mainly in East, South, and Southeast Asia in regions with a monsoon climate. Paddy soils are unique in that they are mostly managed under waterlogged conditions during the rice-growing season. Nitrogen (N) is one of the most important bio-elements for many crops, including rice. N mineralization from soil organic nitrogen is fundamentally important, and works in harmony with an adequate application of nitrogen fertilizer and environmental soil, water, and air quality. For this reason, it has been studied for many decades, but further integrations of quantitative and qualitative research is still needed to improve the basic understanding and inform practical soil managements. For example, some of the traditional indices used for paddy soil fertility in Japan include mineralizable N, mineralized N after waterlogged incubation of air-dried soil, and rate of N mineralization (percentage of mineralizable N in total N), which all vary across different soils and fertilization management practices. However, in several long-term experiments on paddy soils in Japan that had a continuous application of manure, the ratio of the increase in mineralizable N to the total N increased by manure application was nearly constant, indicating that soil N fertility shows a shared an upward trend when manure is applied in the long-term. The extent of these increases was proportional to the amount of total manure applied over the long-term experiments until they reach a certain level. Interestingly, when a standard level of manure was applied, it took approximately 50 years to plateau in Japan, while in the UK, the level of soil organic matter took about 100 years to plateau after the long-term application of manure. This indicates that there is a difference in organic matter stabilization under different climate conditions (Wada et al. Citation1981; Jenkinson and Ladd Citation1981). Later, the dynamics of soil organic matter accumulation was described in a mathematical model for soil in the UK (Roth C model; Coleman and Jenkinson Citation1996), and then modified to fit paddy soil with some modifications to the decomposition rate and other parameters (Shirato and Yokozawa Citation2005).
N mineralization in the paddy soils of Japan and the Philippines were first investigated using mathematical first-order kinetics models (Inubushi, Wada, and Takai Citation1985). It was discovered that N mineralization was regulated by two parameters: namely, the total organic nitrogen content and the clay content. The former parameter regulates mineralization potentials, and the second parameter regulates the mineralization rate constant. Mineralization potentials were positively correlated with the total amount of soil N, while mineralization rate constants were negatively correlated with clay content. Interestingly, the slope of the linear regression showing a negative correlation between mineralization rate and clay content was different for Japanese and Philippine soil, the former slope being about half of the later slope. N mineralization is affected by in situ soil temperature, but it has been suggested that, even under the same incubation temperature, the rate constants remain some influences of the soil origins, either temperate or tropical conditions.
2. Quantification of microbial biomass in Japanese and Philippine paddy soils
The soil microbial biomass is the active nutrient pools of nitrogen and phosphorus among other elements, and it is also an engine for nutrient turnover (Jenkinson and Ladd Citation1981). It was first examined via the chloroform-fumigation incubation method that was originally developed for aerobic upland soils, and later adapted for paddy soil under water-logged conditions in Japan and the Philippines after some modifications (Inubushi, Wada, and Takai Citation1984; Inubushi and Watanabe Citation1986). In the incubation experiment, 15N from both labeled ammonium sulfate and 15N2 gas was incorporated into microbial biomass for a short period (−1 week), and then re-mineralized at the end of the incubation period (−4 weeks) (Inubushi and Watanabe Citation1987). In field experiments using the 15N tracer technique, it was discovered that the soil microbial biomass supplied nitrogen to rice plants, especially during the active rice growth stage. N supplied by the microbial biomass was proportional to the N uptake by rice plant. However, the ratio of N uptake to biomass N varied depending on soil type, and decreased with the application of organic matter (Inubushi et al. Citation1997a). The turn-over rate of nitrogen in microbial biomass in paddy soil was much faster in Japan and the Philippines (Inubushi and Watanabe Citation1986; Shibahara, Yamamuro, and Inubushi Citation1998) than it was in upland soils in Japan (Sakamoto and Hodono Citation2000) and the UK (Jenkinson and Ladd Citation1981) during the growing season.
The chloroform-fumigation extraction method was also modified to suit submerged paddy soil. It was used to compare the microbial biomass in a long-term paddy experimental station in Kounosu, Japan, with the microbial biomass in upland soil at the Rothamsted Experimental Station in Harpenden, UK. The results showed that the amount of microbial biomass decreased rapidly during anaerobic incubation, more rapidly in the Harpenden soils, but it recovered quickly after a short aeration period. This was especially so in the Konosu soils, as was confirmed by ATP measurements, all of which indicate that the unique characteristics of the soil microbial biomass in paddy soil are adapted to regular flooding-drying cycles (Inubushi, Brookes, and Jenkinson Citation1989a, Citation1991). Microbial biomass C and N contents were closely related each other, but the ratio of C to N in microbial biomass depended on paddy soil type and drainage condition during the winter fallow season in Japan (Shibahara and Inubushi Citation1995) and in the Philippines (Witt et al. Citation2000). In long-term rice-wheat experiments in Kyushu, Japan; Ludhiana, India; and Bhairahawa, Nepal, organic amendments affected soil parameters including microbial biomass (Tirol-Padre et al. Citation2005, Citation2007). Even in Uganda, located in sub-Saharan Africa, fertilizer managements in the long-term experiment affected the biochemical and microbiological properties of the soil, including its microbial biomass, in NERICA (New Rice for Africa) cultivated upland (Inubushi et al. Citation2020), where available P was another important factor. On the basis of these studies, organic manure has been recognized as essential for sustainably maintaining soil quality. Furthermore, the amount of organic manure needed to supplement inorganic fertilizers must be optimized to increase C, N and P accumulation in the soil without negatively affecting the crop yield.
3. Dynamics of elements in soil microbial biomass and its community
In Japan, heavy metal (such as cadmium, mercury and arsenicum) contamination of paddy soils became a serious problem starting from the 1880s, mostly due to mining activities (Arao et al. Citation2010). However, some soil parent materials in some area already contained heavy metals substantially. When heavy metal-contaminated paddy fields were reclaimed by covering with uncontaminated low-fertile mountain soil, the microbial biomass proved to be a good indicator for evaluating how soil fertility recovered in the reclaimed fields and how it was stabilized with the amendment of organic matter (Yoshikawa and Inubushi Citation1995).
As significant linear relationship was observed between the microbial biomass C content and the total amount of phospholipid fatty acid (PFLA) in the surface (0–1 cm) and sub-surface (1–10 cm) soils of paddy fields (Acquaye et al. Citation2000; Inubushi et al. Citation2002a; Acquaye and Inubushi Citation2004a, Citation2004b). The effect of different fertilizer types on the microbial biomass N content was small, even with a 20% reduction in coated urea application compared to uncoated urea (Inubushi and Acquaye Citation2004). The difference was also small across different soil types, but was clearer between different soil layers (surface or subsurface). A similar result was also obtained for microbial diversity; fertilizer type and soil type did not have much of an effect on PFLA, but soil layers and timing (flooding or pre-flooding) significantly affected diversity, indicating that these factors are more determinant than soil types or fertilizer type (Inubushi and Nagano Citation2017). This is likely because subsurface soil is more anaerobic than surface soil during rice cultivation, and the oxygenated surface soil is more hospitable for phototrophic microorganisms. These features of soil biodiversity may be common characteristics in various paddy soil ecosystems, although much more diversity was found in flooded water, plant debris and compost (Kimura and Asakawa Citation2006). The microbial diversity in paddy soil has also been studied using DNA and RNA techniques, with work done on denitrifiers (Yoshida et al. Citation2009), methanogens (Watanabe, Kimura, and Asakawa Citation2006) and microbial biomass K in paddy soil (Yamashita et al. Citation2014). The results suggested that better soil management is required to reduce N loss via denitrification and C loss via methane emission, and also that better K fertilizer management is important for the sustainability of the environment. The diversity of methanogens in paddy soil was also reviewed (Dubey Citation2005), and examined in Indian paddy soils with Japanese group to find some similarities with Japanese paddy soils (Dubey et al. Citation2014).
4. Organic matter decomposition and methane production in paddy soil
Organic matter decomposition in paddy soil occurs anaerobically under submerged conditions via a series of reduction reactions, until methane (CH4), a greenhouse gas, is produced as the end product in the final stage of the reduction process. Based on a quantitative analysis of these reactions (Inubushi, Wada, and Takai Citation1984), the rapid decomposition of organic matter in the early stage of submergence is mainly associated with Fe (II) formation, while the gradual decomposition of organic matter in the later stages of submergence is associated with the formation of volatile fatty acids (VFA) and CH4. Paddy fields are regarded as one of the most important sources of CH4, a gas that has about 30 times stronger global warming potential (GWP) than CO2 in molar basis that could enhance climate change (IPCC Citation2013). The total amounts of CH4, CO2 and accumulated VFA produced were set into a mathematical model based on first-order kinetics, similar to the nitrogen mineralization model (Inubushi et al. Citation1997b), which showed that organic matter decomposition is parallelly proceeding in carbon and nitrogen. Their decomposition rates were influenced by temperature and other soil condition, such as air-drying and amendment, among others. In these studies, it was also found that CH4 production decreased when Fe and fertilizers containing S were added (Inubushi, Umebayashi, and Wada Citation1990a; Inubushi et al. Citation1997b; Furukawa, Tsuji, and Inubushi Citation2001). The balance and relationship between oxidizing agents, especially soil Fe, and reducing agents, namely soil organic matter, on methane production was investigated in Southeast Asian paddy, and results showed a similar relationship between oxidizing and reducing agents as has been observed in Japanese paddy soils (Inubushi et al. Citation2018).
5. Methane emission from paddy field and mitigation strategies
The handy potable chamber method was established to measure the methane gas flux of paddy soil, and results confirmed that the rice plant itself is a major passage of methane flux from soil to the atmosphere, while gas buddle is a minor passage (Inubushi et al. Citation1989b). It has also been demonstrated that mid-summer draining of the fields significantly reduced the methane flux in sites in Japan (Inubushi et al. Citation1990b; Yagi et al. Citation1996) and Indonesia (Hadi, Inubushi, and Yagi Citation2010), and this practice has been widely disseminated in Japan through regional and governmental supports. The alternate wetting and drying (AWD) management system has also been tested in Indonesia, the Philippines, Thailand, Vietnam (Setyanto et al. Citation2018; Sibayan et al. Citation2018; Tirol-Padre et al. Citation2018; Chidthaisong et al. Citation2018; Tran et al. Citation2018) and India (Oo et al. Citation2017). Another strategy to reduce methane emission from paddy fields is the amendment of silicate fertilizer with iron sourced from steel slag, which has been shown to reduce methane emission in Japan (Furukawa and Inubushi Citation2002; Singla and Inubushi Citation2015) as well as in Vietnam (a reduction ratio of 3%₋50%; ), while also increasing rice yield (), as seen in other Asian countries. Steel-making slag began being used as a silicate fertilizer in Japan in 1956 because steel-making slag contains a significant amount of available silica, which is an important element for rice growth (Ma and Takahashi Citation2002; Darmawani et al. Citation2006). The effect of steel-making slag on rice yield was seen in some field experiments in Vietnam, especially in soils with low available silicate, measured using the incubation method (JSSSPN Citation1986) (). Silicon elution from steel-making slag fertilizers into a paddy field has also been visualized using an electron probe micro-analyzer (EPMA) (Ito, Shiratori, and Inubushi Citation2015). To mitigate methane emissions from paddy fields, measuring the rate of methane oxidation and methanotrophs activity are also important, particularly in rice plant-soil systems that have different rice cultivars and growth stages (Inubushi et al. Citation2002b). Another mitigation strategy to reduce CH4 emission from paddy fields is via organic farming using aquatic weeds, especially those that have a high methane-oxidation capability (Inubushi et al. Citation2001), as well as microbes such as photosynthetic bacteria (Kato, Iwaishi, and Inubushi Citation2008). Further investigations are needed to test these strategies, such as root-associated soil methanotrophic bacteria as shown by Bao et al. (Citation2014).
Figure 1. Effect of steel making slag on methane emissions in paddy fields in Vietnam. The yield scale total CH4 emission (amount of total CH4 emission divided by rice yield) was calculated for each treatment, and the ratio (the yield scale CH4 emission from steel slag treated plot)/(the yield scale CH4 emission from control) was plotted in the Y-axis. Open bar □ indicates summer season, closed bar ■ indicates spring season
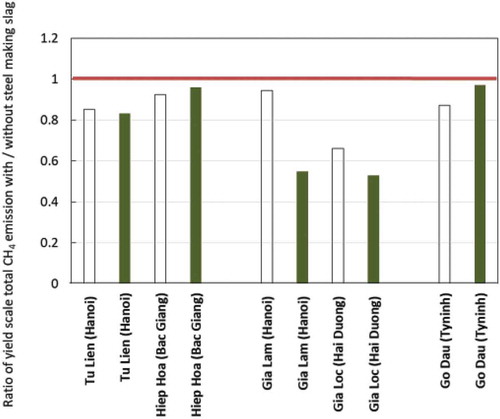
6. Effect of elevated CO2 on methane and soil microbial biomass in paddy soil
To plan a sustainable agricultural management system, the effect of elevated atmospheric CO2 on agricultural ecosystem should be investigated. At first, effects of elevated CO2 levels on soil biological functions were investigated in microcosm experiments without rice plants. It was found that the soil microbial biomass was increased but CH4 emissions were decreased by elevated CO2 due to the higher O2 content produced by higher photosynthesis rates in flooded water, as well as due to methane oxidation (Inubushi, Cheng, and Chander Citation1999; Cheng, Chander, and Inubushi Citation2000). However, in a paddy field under free-air CO2 enrichment (FACE) conditions in Shizukuishi, Iwate, an elevated CO2 also increased CH4 emission, due more to enhanced rice plant growth rather than CH4 oxidation (Inubushi et al. Citation2003a), which indicates positive feedback loop exacerbating global warming. Under normal N fertilizer application levels, available N decreased for the rice plants, soil microbial biomass N content also decreased, while biological N2 fixation activity increased (Hoque et al. Citation2001). However, under a higher application rate of N fertilizer, research in China showed that CH4 emissions increased with an increase in N application rate under elevated CO2 conditions (Zheng et al. Citation2006). These are important insights to inform and manage future agroecosystems that will live under elevated atmospheric CO2 conditions. Further investigations in rice FACE in Tsukubamirai, Ibaraki, are continuing on elevated soil temperature, with results being evaluated using carbon isotope signature changes (as seen in Hayashi et al. Citation2014; Matsushima et al. Citation2018)
7. Tropical peat soil, wetland, and permafrost forest soil
Similar to paddy soil, peat soil is found in wetlands under waterlogged conditions. Although wetlands occupy only 4%–6% of the land surface area of the world (Aselmann and Crutzen Citation1989), it holds about 20%–25% of the terrestrial carbon and nitrogen (Batjes Citation1996). Approximately 29 million ha of peat is in the tropical region, and a large proportion of that is on Borneo Island (Driesen Citation1978; Takai Citation1997). Tropical peatlands can become a source of greenhouse gas emissions because they store large amounts of soil carbon and nitrogen. However, these emissions are strongly influenced by soil moisture conditions. The tropical monsoon climate is characterized typically by its wet and dry seasons. Seasonal changes in the emission of CO2, CH4 and nitrous oxide (N2O) were measured using the closed chamber method for over a year at three sites (secondary forest, paddy field and upland field) in the tropical peatland in South Kalimantan, Indonesia (Inubushi et al. Citation2003b; Hadi et al. Citation2000, Citation2005). The quantity of gases emitted from the fields varied tremendously according to the seasonal pattern of precipitation; methane emission rates, in particular, were strongly correlated with precipitation. Converting secondary forest peatland into paddy fields tended to increase the annual emissions of CO2 and CH4 to the atmosphere, while changing land cover from secondary forest to upland field tended to decrease these emissions. However, no clear trend was observed for N2O, which had both positive and negative values at all three sites. Similarly, monthly measurements of CO2, CH4, and N2O fluxes in peat soils were recorded and compared with the groundwater level for over a year at four sites (drained forest, upland cassava (Manihot esculenta) plantation, upland paddy fields, lowland paddy fields) located in Jambi province, Indonesia (Furukawa et al. Citation2005). Land-use change from drained forest to lowland paddy field significantly decreased CO2 and N2O fluxes but increased the CH4 flux in the soils. Changing a drained forest into a cassava field significantly increased the N2O flux, but had no significant influence on CO2 and CH4 fluxes in the soil. Groundwater levels of the drained forest and the upland crop fields had gone down due to drainage ditches getting dug out, while swamp forest and lowland paddy fields were flooded. Groundwater levels were also affected by precipitation. Groundwater levels were found to be negatively correlated to CO2 flux but positively related to CH4 flux at all investigation sites. The greatest N2O flux was observed a groundwater level of −20 cm. The effects of land-use changes factors such as intensity of drainage, forest fires, and agricultural land use conversion, on the control of gas emission factors were also investigated by looking at the characteristics of the microbes in tropical peat soils in Central Kalimantan, Indonesia (Arai et al. Citation2014a). The influences of water depth and soil amelioration on greenhouse gas emissions from peat soil columns has also been investigated, which were similar in tendency as in the field and were easier method to monitor over a short period but not over a long period (Susilawati et al. Citation2016). Another factors controlling CH4 fluxes in peat soil is the water-filled pore space (WFPS), which showed a significantly positive relationship with CH4 fluxes and a negative relationship with the of methanotrophs populations. However, the methanotrophic community was unaffected by drainage and forest fires (Arai et al. Citation2014b). In oil palm plantations, even with well-managed drainage, tropical peat soil can become significant sources of CO2 and N2O (Sakata et al. Citation2015). On the other hand, natural mangrove ecosystems in Vietnam and India showed limited amounts of CH4 flux, that was influenced by the chemical properties, natural vegetation, and tidal water movement of this unique ecosystem with its salt-tolerant methanogenic community (Arai et al. Citation2016). The methanogenic and methanotrophic activity and community structure and how they relate to CO2 and CH4 emissions in the frozen soil core samples taken from Alaskan permafrost forest have also been investigated (Nagano et al. Citation2018), with results indicating that global warming is affecting CH4 release from the thawing of frozen soil.
8. Andosols
In Japan, about half of all arable lands are paddy fields, while the other half are upland fields (MAFF (Ministry of Agriculture, Fishery and Forestry) Citation1991). A quarter of the total of arable lands is covered by Andosols or Andisols that are derived from volcanic parent material, and similar conditions are found in other Asian countries with volcanos, such as the Philippines and Indonesia (Parfitt and Clayden Citation1991). The microbial biomass and greenhouse gas dynamics in Andosol and non-Andosol soils were also compared. The ratio of microbial biomass C to total C in Andosol was found to be much smaller than in non-Andosol soils (Inubushi, Sakamoto, and Sawamoto Citation2005), which indicates a much slower soil organic matter turnover and stability in Andosols. The effect of organic fertilizer on microbial biomass was also examined in Andosols in vegetable fields, in the context of crop growth and greenhouse gas emissions (Goyal et al. Citation1999; Goyal, Sakamoto, and Inubushi Citation2000, Citation2006a, b; Inubushi et al. Citation2000; Nagano et al. Citation2012a). Several organic treatments, even over a short period (<2 years) increased both microbial biomass and crop growth to some extent. The environmental impact of recycling organic residues in grassland was also investigated (Ushiwata, Sasa, and Inubushi Citation2007). The effects can be seen not only in the surface soil but also in the subsurface soils (Zaman et al. Citation2002a, b, Citation2004; Matsushima, Choi, and Inubushi Citation2009). The ratio of soil microbial biomass C (MBC) and soil organic C (SOC) was compared in an Andosol and non-Andosol (sand-dune Regosol) soils from Japan and at Rothamsted Experimental Station, UK (Inubushi and Kong Citation2014). A significant linear correlation was found between MBC and SOC in Japanese Andosols, but the slope of the linear regression (MBC/SOC) was significantly less steep for Andosols than for non-Andosols. This indicates that MBC was relatively lower at the same SOC level in the Andosol than non-Andosol. Subsurface Andosol buried in paleosol had a higher MBC than the upper subsoil layer, indicating that the accumulated soil C may become a substrate for microorganisms even thousands of years after eruption and surface horizon formation (Inubushi and Kong Citation2014). These findings highlight the unique characteristics of microbial biomass and C turnover in Andosols. Even when Andosols naturally contain large amounts of soil organic matter due to acidic soil conditions, microbial biomass and activity are sensitive indicators that can show how soil organic matter changes.
Microbial biomass and greenhouse gas production and consumption potential of Andosols and non-Andosols under different land-use types were compared in central Japan and eastern Hungary, respectively, showing that aside from climatic conditions, also soil type and land-use type were principal factors influencing soil microbial properties and greenhouse gas dynamics (Kong et al. Citation2013a; Citation2013b). Another field investigation into land-use history was conducted in semi-dry Eurasian steppe soil. The effects of land-use change history (cultivated land changing back to preserved grassland after 18 years) on microbial biomass and activity were examined by taking soil and gas samples from nearby sites with a similar soil type but with different land-use history. Microbial biomass C was significantly larger in former pastures than in former crop land, both having been converted and currently under preserved grassland. However, there was no significant difference in CO2 efflux between the two sites, indicating that the microbial biomass was affected not only by the current land-use type but also by prior land-use (Nagano et al. Citation2012b). Soil microbial biomass can therefore be a useful environmental index, especially under such critical or extreme conditions.
Research has been done on the influence of vegetation types and soil properties on microbial biomass carbon and metabolic quotients in temperate volcanic and tropical forest soils (Xu, Inubushi, and Sakamoto Citation2006. Xu et al. Citation2007; Nagano et al. Citation2012a). An investigation using multiple timescale variations and controls of soil/root respiration and soil microbial biomass in a tropical dry Dipterocarp forest in western Thailand found that soil microbial biomass played a crucial role in the magnitude and temporal variation of soil respiration. In this forest ecosystem, large seasonal variations in soil respiration were observed and were mainly attributed to the response of soil microbial biomass to soil moisture (Hanpattanakit et al. Citation2015).
9. Future research challenges
In paddy soil ecosystems, modern technology such as heavy machinery and low-cost soil management are used not only in Japan but also in many other rice-growing countries. However, their effects on soil microbes, organic matter, soil fertility, and climate change is still not clearly understood. Consequently, the effectiveness of these technologies in sustainable agriculture and land management is not yet proven. Similarly, human impact is also increasing in wetland ecosystems, via interventions such as drainage, wild-fire, and the thawing of the permafrost. Other global issues such as soil C sequestration (Lal Citation2020), greenhouse gas emission and consumption, climate change, land use and land cover changes, desertification, and deforestation have become more and more critical issues in many countries and regions. Soil has many characteristics and features, such as productivity, soil fertility, water holding capacity, ability to moderate climate change, biodiversity, soil health for environmental and human health, in relation to bio-elements’ (C, N, P, K, Fe, Si, etc.) cycling and air and water dynamics, which interact with each other (). Further research with a multi-disciplinary approach to soil science is urgently needed to assess current sustainable practices and to provide long-term prospects for more sustainable agriculture and nature.
Supplemental Material
Download MS Word (65.5 KB)Acknowledgments
This research was initiated under the guidance of Professor Emeritus Yasuo Takai and the late Dr. Hidenori Wada of the University of Tokyo.
Disclosure statement
No potential conflict of interest was reported by the author.
Supplementary material
Supplemental data for this article can be accessed here.
References
- Acquaye, S., A. Hadi, W. Cheng, and K. Inubushi. 2000. “Effect of Fertilizers on Soil Microbial Biomass in Two Types of Paddy Fields.” Technical Bulletin of Faculty of Horticulture, Chiba University 54: 1–11. https://ci.nii.ac.jp/naid/10012935594.
- Acquaye, S., and K. Inubushi. 2004a. “Comparative Effects of Application of Coated and Non-coated Urea in Clayey and Sandy Paddy Soil Microcosms Examined by the 15N Tracer Technique, I. Effects on Growth, N Uptake and Yield of Rice Crop.” Soil Science and Plant Nutrition 50: 205–213. doi:10.1080/00380768.2004.10408469.
- Acquaye, S., and K. Inubushi. 2004b. “Comparative Effects of Application of Coated and Non-coated Urea in Clayey and Sandy Paddy Soil Microcosms Examined by the 15N Tracer Technique, II. Effects on Soil Microbial Biomass N and Microbial 15N Immobilization.” Soil Science and Plant Nutrition 50: 215–223. doi:10.1080/00380768.2004.10408470.
- Arai, H., A. Hadi, U. Darung, S. H. Limin, H. Takahashi, R. Hatano, and K. Inubushi. 2014a. “Land Use Change Affects Microbial Biomass and Fluxes of Carbon Dioxide and Nitrous Oxide in Tropical Peatlands.” Soil Science and Plant Nutrition 60 (3): 423–434. doi:10.1080/00380768.2014.903576.
- Arai, H., A. Hadi, U. Darung, S. H. Limin, R. Hatano, and K. Inubushi. 2014b. “A Methanotrophic Community in A Tropical Peatland Is Unaffected by Drainage and Forest Fires in A Tropical Peat Soil.” Soil Science and Plant Nutrition 60 (4): 577–585. doi:10.1080/00380768.2014.922034.
- Arai, H., R. Yoshioka, S. Hanazawa, V. Q. Minh, V. Q. Tuan, T. K. Tinh, T. Q. Phu, et al. 2016. “Function of the Methanogenic Community in Mangrove Soils as Influenced by the Chemical Properties of the Hydrosphere.” Soil Science and Plant Nutrition 62 (2): 150–163. doi:10.1080/00380768.2016.1165598.
- Arao, T., S. Ishikawa, M. Murakami, K. Abe, Y. Maejima, and T. Makino. 2010. “Heavy Metal Contamination of Agricultural Soil and Countermeasures in Japan Paddy and Water Environment.” Paddy and Water Environment 8 (3): 247–257.
- Aselmann, I., and P. J. Crutzen. 1989. “Global Distribution of Natural Freshwater Wetlands and Rice Paddies, Their Net Primary Productivity, Seasonality, and Possible Methane Emissions.” Journal of Atmospheric Chemistry 8: 307–358. doi:10.1007/BF00052709.
- Bao, Z., T. Okubo, K. Kubota, Y. Kasahara, H. Tsurumaru, M. Anda, S. Ikeda, and K. Minamisawa. 2014. “Metaproteomic Identification of Diazotrophic Methanotrophs and Their Localization in Root Tissues of Field-grown Rice Plants.” Applied and Environmental Microbiology 80 (16): 5043–5052. doi:10.1128/AEM.00969-14.
- Batjes, N. H. 1996. “Total Carbon and Nitrogen in the Soils of the World.” European Journal of Soil Science 47: 151–163. doi:10.1111/j.1365-2389.1996.tb01386.x.
- Cheng, W., K. Chander, and K. Inubushi. 2000. “Effect of Elevated CO2 and Temperature on Methane Production and Emission from Submerged Soil Microcosms.” Nutrient Cycling in Agroecosystems 58: 339–347.
- Chidthaisong, A., N. Cha-un, B. Rossopa, C. Buddaboon, C. Kunuthai, P. Sriphirom, S. Towprayoon, T. Tokida, A. Tirol, and K. Minamikawa. 2018. “Evaluating the Effects of Alternate Wetting and Drying (AWD) on Methane and Nitrous Oxide Emissions from a Paddy Field in Thailand.” Soil Science and Plant Nutrition 64 (1): 31–38. doi:10.1080/00380768.2017.1399044.
- Coleman, K., and D. S. Jenkinson. 1996. “RothC-26.3-A Model for the Turnover of Carbon in Soil.” In Evaluation of Soil Organic Matter Models: Using Existing Long-Term Datasets, edited by D. S. Powlson, P. Smith, and J. U. Smith, 237–246. Berlin: Springer.
- Darmawani, K. K., A. Saleh, H. Subagjo, T. Masunaga, and T. Wakatsuki. 2006. “Effect of Long-term Intensive Rice Cultivation on the Available Silica Content of Sawah Soils: Java Island, Indonesia.” Soil Science and Plant Nutrition 52: 745–753. doi:10.1080/00380768.2017.1401906.
- Driesen, P. M. 1978. “Peat Soil.” In Soils and Rice, edited by IRRI, 763–779. Los Baños, Philippines: IRRI.
- Dubey, S. K. 2005. “Microbial Ecology of Methane Emission in Rice Agroecosystem.” Applied Ecology and Environmental Research 3 (2): 1–27. http://www.aloki.hu/pdf/0302_001027.pdf.
- Dubey, S. K., A. Singh, T. Watanabe, S. Asakawa, A. Singla, H. Arai, and K. Inubushi. 2014. “Methane Production Potential and Methanogenic Archaeal Community Structure in Tropical Dryland Irrigated Indian Paddy Soils.” Biology and Fertility of Soils 50: 369–379. doi:10.1007/s00374-013-0858-7.
- Furukawa, Y., and K. Inubushi. 2002. “Feasible Suppression Technique of Methane Emission from Paddy Soil by Iron Amendment.” Nutrient Cycling in Agroecosystems 64 (1–2): 193–201.
- Furukawa, Y., K. Inubushi, M. Ali, A. M. Itang, and H. Tsuruta. 2005. “Effect of Changing Groundwater Levels Caused by Land-use Changes on Greenhouse Gas Emissions from Tropical Peatlands.” Nutrient Cycling in Agroecosystems 71: 81–91. doi:10.1007/s10705-004-5286-5.
- Furukawa, Y., T. Tsuji, and K. Inubushi. 2001. “Suppression of Methane Emission from Paddy Soil by Application of Iron Materials.” Japanese Journal of Soil Science and Plant Nutrition 72 (2): 257–264. (in Japanese with English summary). https://ci.nii.ac.jp/naid/10015011966/
- Goyal, S., K. Inubushi, S. Kato, H. L. Xu, and H. Umemura. 1999. “Effect of Anaerobically Fermented Manure on the Soil Organic Matter, Microbial Properties and Growth of Spinach under Greenhouse Conditions.” Indian Journal of Microbiology 39 (4): 211–216.
- Goyal, S., K. Sakamoto, and K. Inubushi. 2000. “Microbial Biomass and Activities along an Andosol Profile in Relation to Soil Organic Matter Level.” Microbes and Environment 15 (3): 143–150. doi:10.1264/jsme2.2000.143.
- Goyal, S., K. Sakamoto, and K. Inubushi. 2006a. “Decomposition of Sewage Sludge Compost and Its Effect on Soil Microbial Biomass and Growth of Spinach.” Research on Crops 7 (2): 517–521.
- Goyal, S., K. Sakamoto, K. Inubushi, and K. Kamewada. 2006b. “Long-term Effects of Inorganic Fertilization and Organic Amendments on Soil Organic Matter and Soil Microbial Properties in Andisols.” Archives of Agronomy and Soil Science 52 (6): 617–625. doi:10.1080/03650340601048595.
- Hadi, A., K. Inubushi, E. Purnomo, F. Razie, K. Yamakawa, and H. Tsuruta. 2000. “Effect of Land-use Changes on Nitrous Oxide (N2O) Emission from Tropical Peatlands.” Chemosphere-Global Change Sciences 2: 347–358. doi:10.1016/S1465-9972(00)00030-1.
- Hadi, A., K. Inubushi, and K. Yagi. 2010. “Effect of Water Management on Greenhouse Gas Emissions and Microbial Properties of Paddy Soils in Japan and Indonesia.” Paddy and Water Environment 8: 319–324. doi:10.1007/s10333-010-0210-x.
- Hadi, A., K. Inubushi, Y. Furukawa, E. Purunomo, M. Rasmadi, and H. Tsuruta. 2005. “Greenhouse Gas Emissions from Tropical Peatlands of Kalimantan, Indonesia.” Nutrient Cycling in Agroecosystems 71: 73–80. doi:10.1007/s10705-004-5286-5.
- Hanpattanakit, P., M. Y. Leclerc, A. M. S. Mcmillan, P. Limtong, J. L. Maeght, S. Panuthai, K. Inubushi, and A. Chidthaisong. 2015. “Multiple Timescale Variations and Controls of Soil Respiration in a Tropical Dry Dipterocarp Forest, Western Thailand.” Plant and Soil 390 (1): 167–181. doi:10.1007/s11104-015-2386-8.
- Hayashi, K., T. Tokida, M. Yashima Matsushima, K. Ono, H. Nakamura, and T. Hasegawa. 2014. “Free-air CO2 Enrichment (FACE) Net Nitrogen fixation Experiment at a Paddy Soil Surface under Submerged Conditions.” Nutrient Cycling in Agroecosystems 98: 57–69. doi:10.1007/s10705-013-9595-4.
- Hoque, M. M., K. Inubushi, S. Miura, K. Kobayashi, H.-Y. Kim, M. Okada, and S. Yabashi. 2001. “Biological Dinitrogen Fixation and Soil Microbial Biomass Carbon as Influenced by Free-air Carbon Dioxide Enrichment (FACE) at Three Levels of Nitrogen Fertilization in a Paddy Field.” Biology and Fertility of Soils 34: 453–459. doi:10.1007/s00374-001-0430-8.
- Inubushi, K., and Y. Kong. 2014. “Soil Microbial Biomass and C Storage of an Andosol.” In Soil Carbon, Progress in Soil Science, edited by A. E. Hartemink and K. McSweeney, 173–178. Switzerland: Springer.
- Inubushi, K., and H. Nagano. 2017. “Microbial Biomass and Functions in Paddy Soil.” In Microbial Biomass – A Paradigm Shift in Terrestrial Biogeochemistry, edited by K. Tate, 103–117. Singapore: World Scientific.
- Inubushi, K., F. Shibahara, K. Hasegawa, and S. Yamamuro. 1997a. “Effects of Added Organic Matter on Microbial Biomass Nitrogen Dynamics and Plant Uptake in Paddy Soil.” Developments in Plant and Soil Sciences 78: 777-778. doi:10.1007/978-94-009-0047-9_252.
- Inubushi, K., H. Saito, H. Arai, K. Ito, K. Endoh, and M. M. Yashima. 2018. “Effect of Oxidizing and Reducing Agents in Soil on Methane Production in Southeast Asian Paddies.” Soil Science and Plant Nutrition 64 (1): 84–89. doi:10.1080/00380768.2017.1401907.
- Inubushi, K., H. Sugii, I. Watanabe, and R. Wassmann. 2002b. “Evaluation of Methane Oxidation in Rice Plant-soil System.” Nutrient Cycling in Agroecosystems 64 (1–2): 71–77. doi:10.1023/A:1021136431176.
- Inubushi, K., H. Sugii, S. Nishino, and E. Nishino. 2001. “Effect of Aquatic Weeds on Methane Emission from Submerged Paddy Soil.” American Journal of Botany 88 (6): 975–979. doi:10.2307/2657078.
- Inubushi, K., H. Wada, and Y. Takai. 1984. “Easily Decomposable Organic Matter in Paddy Soil, (IV) Relationship between Reduction Process and Organic Matter Decomposition.” Soil Science and Plant Nutrition 30 (2): 189-198. doi:10.1080/00380768.1984.10434682.
- Inubushi, K., H. Wada, and Y. Takai. 1985. “Easily Decomposable Organic Matter in Paddy Soil (VI), Kinetics of Nitrogen Mineralization in Submerged Soils.” Soil Science and Plant Nutrition 31 (4): 563-572. doi:10.1080/00380768.1985.10557464.
- Inubushi, K., and I. Watanabe. 1986. “Dynamics of Available Nitrogen in Paddy Soils (II) Mineralized N of Chloroform-fumigated Soil as a Nutrient Source for Rice.” Soil Science and Plant Nutrition 32 (4): 561-577. doi:10.1080/00380768.1986.10557538.
- Inubushi, K., and I. Watanabe. 1987. “Microbial Biomass Nitrogen in Anaerobic Soil as Affected by N-immobilization and N2-fixation.” Soil Science and Plant Nutrition 33 (2): 213-224. doi:10.1080/00380768.1987.10557567.
- Inubushi, K., K. Hori, S. Matsumoto, and H. Wada. 1997b. “Anaerobic Decomposition of Organic Carbon in Paddy Soil in Relation to Methane Emission to the Atmosphere.” Water Science and Technology 36 (6–7): 523-530. https://search.proquest.com/docview/1943332040?accountid=26282.
- Inubushi, K., K. Hori, S. Matsumoto, M. Umebayashi, and H. Wada. 1989b. “Methane Emission through Rice Plant to the Atmosphere.” Japanese Journal of Soil Science and Plant Nutrition 60: 318–324. (in Japanese with English summary). https://ci.nii.ac.jp/naid/10008749332/
- Inubushi, K., K. Sakamoto, and T. Sawamoto. 2005. “Properties of Microbial Biomass in Acid Soils and Their Turnover.” Soil Science and Plant Nutrition 51 (5): 605–608. doi:10.1111/j.1747-0765.2005.tb00073.x.
- Inubushi, K., M. Umebayashi, and H. Wada, 1990a. “Methane Emission from Paddy Fields.” 14th International Congress of Soil Science, Transactions, II-249-254. https://www.cabdirect.org/cabdirect/abstract/19911951840
- Inubushi, K., M. Yashima, S. Hanazawa, A. Goto, K. Miyamoto, T. Tsuboi, and G. Asea. 2020. “Long-term Fertilizer Management in NERICA Cultivated Upland Affects on Soil Bio-chemical Properties.” Soil Science and Plant Nutrition 66: 247–253. doi:10.1080/00380768.2019.1705738.
- Inubushi, K., P. C. Brookes, and D. S. Jenkinson. 1989a. “Adenosine 5ʹ-triphosphate and Adenylate Energy Charge in Waterlogged Soil.” Soil Biology & Biochemistry 21 (5): 733-739. doi:10.1016/0038-0717(89)90072-2.
- Inubushi, K., P. C. Brookes, and D. S. Jenkinson. 1991. “Soil Microbial Biomass C, N and ninhydrin-N in Aerobic and Anaerobic Soils Measured by the Fumigation-extraction Method.” Soil Biology & Biochemistry 23 (8): 737-741. doi:10.1016/0038-0717(91)90143-8.
- Inubushi, K., and S. Acquaye. 2004. “Role of Microbial Biomass in Biogeochemical Processes in Paddy Soil Environments.” Soil Science and Plant Nutrition 50 (6): 793–805. doi:10.1080/00380768.2004.10408539.
- Inubushi, K., S. Acquaye, S. Tsukagoshi, F. Shibahara, and S. Komatsu. 2002a. “Effects of Controlled-release Coated Urea (CRCU) on Soil Microbial Biomass N in Paddy Fields Examined by the 15N Tracer Technique.” Nutrient Cycling in Agroecosystems 63 (2–3): 291–300. doi:10.1023/A:1021111126976.
- Inubushi, K., S. Goyal, K. Sakamoto, Y. Wada, K. Yamakawa, and T. Arai. 2000. “Influence of Application of Sewage Sludge Compost on N2O Production in Soils.” Chemosphere-Global Change Sciences 2: 329–334. doi:10.1016/S1465-9972(00)00026-X.
- Inubushi, K., W. Cheng, and K. Chander. 1999. “Carbon Dynamics in Submerged Soil Microcosms as Influenced by Elevated CO2 and Temperature.” Soil Science and Plant Nutrition 45 (4): 863–872. doi:10.1080/00380768.1999.10414335.
- Inubushi, K., W. Cheng, S. Aonuma, M. M. Hoque, K. Kobayashi, S. Miura, H. Y. Kim, and M. Okada. 2003a. “Effects of Free-air CO2 Enrichment (FACE) on CH4 Emission from a Rice Paddy Field.” Global Change Biology 9 (10): 1458–1464. doi:10.1046/j.1365-2486.2003.00665.x.
- Inubushi, K., Y. Furukawa, A. Hadi, E. Purnomo, and H. Tsuruta. 2003b. “Seasonal Changes of CO2, CH4 and N2O Fluxes in Relation to Land-use Change in Tropical Peatlands Located in Coastal Area of South Kalimantan.” Chemosphere 52 (3): 603–608. doi:10.1016/S0045-6535(03)00242-X.
- Inubushi, K., Y. Muramatsu, D. Taja, and M. Umebayashi. 1990b. “Control of Methane Emission from Paddy Soil.” 14th International Congress of Soil Science, Transactions, II–431–432. Kyoto, Japan
- IPCC. 2013. “The Physical Science Basis. Contribution of Working Group I to the Fifth Assessment Report of the Intergovernmental Panel on Climate Change.” The Physical Science Basis. Contribution of Working Group I to the Fifth Assessment Report of the Intergovernmental Panel on Climate Change. Cambridge, UK: Cambridge University Press.
- Ito, K. E., Y. Shiratori, and K. Inubushi. 2015. “Silicon Elution from Three Types of Steel Slag Fertilizers in a Paddy Field Analyzed by Electron Probe Micro-analyzer (EPMA).” Soil Science and Plant Nutrition 61 (5): 835–845. doi:10.1080/00380768.2015.1064326.
- Jenkinson, D. S., and J. N. Ladd. 1981. “Microbial Biomass in Soil: Measurement and Turnover.” In Soil Biochemistry, edited by E. A. Paul, and J. N. Ladd, 415–471. Vol. 5. New York: Marcel Dekker.
- JSSSPN. 1986. Methods of Soil Nutrient Analysis. Tokyo, Japan: Yokendo.
- Kato, S., S. Iwaishi, and K. Inubushi. 2008. “Effect of Microbial Material Solution on Methane Emission from Paddy Soil.” HortResearch 62: 39–44. http://agris.fao.org/agris-search/search.do?recordID=JP2008007137.
- Kimura, M., and S. Asakawa. 2006. “Comparison of Community Structures of Microbiota at Main Habitats in Rice Field Ecosystems Based on Phospholipid Fatty Acid Analysis.” Biology and Fertility of Soils 43: 20–29. doi:10.1007/s00374-005-0057-2.
- Kong, Y., H. Nagano, J. Kátai, I. Vágó, Á. Z. Oláh, M. Yashima, and K. Inubushi. 2013a. “CO2, N2O and CH4 Production/consumption Potentials of Soils under Different Land-use Types in Central Japan and Eastern Hungary.” Soil Science and Plant Nutrition 59: 455–462. doi:10.1080/00380768.2013.775005.
- Kong, Y., M. Watanabe, H. Nagano, K. Watanabe, M. Yashima, and K. Inubushi. 2013b. “Effects of Land-use Type and Nitrogen Addition on Nitrous Oxide and Carbon Dioxide Production Potentials in Japanese Andosols.” Soil Science and Plant Nutrition 59: 790–799. doi:10.1080/00380768.2013.817938.
- Lal, R. 2020. “Managing Soils for Negative Feedback to Climate Change and Positive Impact on Food and Nutritional Security.” Soil Science and Plant Nutrition 66 (1): 1–9. doi:10.1080/00380768.2020.1718548.
- Ma, J. F., and E. Takahashi. 2002. Soil. Fertilizer, and Plant Silicon Research in Japan. Tokyo: Elsevier.
- MAFF (Ministry of Agriculture, Fishery and Forestry). 1991. “Current Status and Countermeasures of Arable Soils in Japan.” In Japanese Society of Soil Science and Plant Nutrition, 40–41. Tokyo: Hakuyuusha.
- Matsushima, M., W.-J. Choi, and K. Inubushi. 2009. “Nitrification Inhibitor Reduces N2O Production from Different Soil Profiles of an Andosol.” Communications in Soil Science and Plant Analysis 40: 3194–3206. doi:10.1080/00103620903261692.
- Matsushima, M. Y., W. Cheng, T. Tokida, H. Nakamura, K. Inubushi, M. Okada, and T. Hasegawa. 2018. “Effects of Free-air CO2 Enrichment on Plant-derived Carbon Input to Paddy Andosol and Ultisol as Evaluated by Carbon Isotope Signature Changes.” HortResearch 72: 19-28. https://core.ac.uk/download/pdf/158826718.pdf.
- Nagano, H., S. Kato, S. Ohkubo, and K. Inubushi. 2012a. “Emissions of Carbon Dioxide, Methane, and Nitrous Oxide from Short- and Long-term Organic Farming Andosols in Central Japan.” Soil Science and Plant Nutrition 58 (6): 793–801. doi:10.1080/00380768.2012.739550.
- Nagano, H., S. Sugihara, M. Matsushima, S. Okitsu, V. E. Prikhodko, E. Manakhova, G. B. Zdanovich, et al. 2012b. “Carbon and Nitrogen Contents and Greenhouse Gas Fluxes of the Eurasian Steppe Soils with Different Land-use Histories Located in the Arkaim Museum Reserve of South Ural, Russia.” Soil Science and Plant Nutrition 58 (2): 238–244. doi:10.1080/00380768.2012.661354.
- Nagano, H., Y. Kim, B.-Y. Lee, H. Shigeta, and K. Inubushi. 2018. “Laboratory Examination of Greenhouse Gaseous and Microbial Dynamics during Thawing of Frozen Soil Core Collected from a Black Spruce Forest in Interior Alaska.” Soil Science and Plant Nutrition 64 (5): 793–801. doi:10.1080/00380768.2018.1525267.
- Oo, A. Z., S. Sudo, K. Inubushi, M. Mano, A. Yamamoto, K. Ono, T. Osawa, et al. 2017. “Methane and Nitrous Oxide Emissions from Conventional and Modified Rice Cultivation Systems in South India.” Agriculture, Ecosystems & Environment 252: 148–158. doi:10.1016/j.agee.2017.10.014.
- Parfitt, R. L., and B. Clayden. 1991. “Andisols — The Development of a New Order in Soil Taxonomy.” Geoderma. 49 (3–4): 181–198. doi:10.1016/0016-7061(91)90075-5.
- Sakamoto, K., and N. Hodono. 2000. “Turnover Time of Microbial Biomass Carbon in Japanese Upland Soils with Different Textures.” Soil Science and Plant Nutrition 46 (2): 483–490. doi:10.1080/00380768.2000.10408801.
- Sakata, R., S. Shimada, H. Arai, N. Yoshioka, R. Yoshioka, H. Aoki, N. Kimoto, A. Sakamoto, L. Melling, and K. Inubushi. 2015. “Effect of Soil Types and Nitrogen Fertilizer on Nitrous Oxide and Carbon Dioxide Emissions in Oil Palm Plantations.” Soil Science and Plant Nutrition 61: 48–60. doi:10.1080/00380768.2014.960355.
- Setyanto, P., A. Pramono, T. A. Adriany, H. L. Susilawati, T. Tokida, A. Tirol-Padre, and K. Minamikawa. 2018. “Alternate Wetting and Drying Reduces Methane Emission from a Rice Paddy in Central Java, Indonesia without Yield Loss.” Soil Science and Plant Nutrition 64 (1): 23–30. doi:10.1080/00380768.2017.1409600.
- Shibahara, F., and K. Inubushi. 1995. “Measurements of Microbial Biomass C and N in Paddy Soils by the Fumigation-extraction Method.” Soil Science and Plant Nutrition 41 (4): 681-689. doi:10.1080/00380768.1995.10417018.
- Shibahara, F., S. Yamamuro, and K. Inubushi. 1998. “Dynamics of Microbial Biomass Nitrogen as Influenced by Organic Matter Application in Paddy Fields, I. Fate of Fertilizer and Soil Organic N Determined by 15N Tracer Technique.” Soil Science and Plant Nutrition 44: 167–178. doi:10.1080/00380768.1998.10414437.
- Shirato, Y., and M. Yokozawa. 2005. “Applying the Rothamsted Carbon Model for Long-term Experiments on Japanese Paddy Soils and Modifying It by Simple Tuning of the Decomposition Rate.” Soil Science and Plant Nutrition 51: 405–415. doi:10.1111/j.1747-0765.2005.tb00046.x.
- Sibayan, E. B., K. Samoy-Pascual, F. S. Grospe, M. E. D. Casil, T. Tokida, A. Tirol-Padre, and K. Minamikawa. 2018. “Effects of Alternate Wetting and Drying Technique on Greenhouse Gas Emissions from Irrigated Rice Paddy in Central Luzon, Philippines.” Soil Science and Plant Nutrition 64 (1): 39–46. doi:10.1080/00380768.2017.1401906.
- Singla, A., and K. Inubushi. 2015. “Effect of Biochar on CH4 and N2O Emission from Soils Vegetated with Paddy.” Paddy and Water Environment 12: 239–243. doi:10.1007/s10333-013-0405-z.
- Susilawati, H. L., P. Setyanto, M. Ariani, A. Hervani, and K. Inubushi. 2016. “Influence of Water Depth and Soil Amelioration on Greenhouse Gas Emissions from Peat Soil Columns.” Soil Science and Plant Nutrition 62: 57–68. doi:10.1080/00380768.2015.1107459.
- Takai, Y., 1997. “Environmental Characteristics and Management in Peat/Acid Sulfate Soils of Southeast Asia.” MAB Report, Japan, pp. 31–49.
- Tirol-Padre, A., J. K. Ladha, A. P. Regmi, A. L. Bhandari, and K. Inubushi. 2007. “Organic Amendments Affect Soil Parameters in Two Long-term Rice-wheat Experiments.” Soil Science Society of America, Journal 71: 442–452. doi:10.2136/sssaj2006.0141.
- Tirol-Padre, A., K. Minamikawa, T. Tokida, R. Wassmann, and K. Yagi. 2018. “Site-specific Feasibility of Alternate Wetting and Drying as a Greenhouse Gas Mitigation Option in Irrigated Rice Fields in Southeast Asia: A Synthesis.” Soil Science and Plant Nutrition 64 (1): 2–13. doi:10.1080/00380768.2017.1409602.
- Tirol-Padre, A., K. Tsuchiya, K. Inubushi, and J. K. Ladha. 2005. “Enhancing Soil Quality through Residue Management in a Rice-wheat System in Fukuoka, Japan.” Soil Science and Plant Nutrition 51 (6): 849–860. doi:10.1111/j.1747-0765.2005.tb00120.x.
- Tran, D. H., T. N. Hoang, T. Tokida, A. Tirol-Padre, and K. Minamikawa. 2018. “Impacts of Alternate Wetting and Drying on Greenhouse Gas Emission from Paddy Field in Central Vietnam.” Soil Science and Plant Nutrition 64 (1): 14–22. doi:10.1080/00380768.2017.1409601.
- UN. 2015. Transforming Our World: The 2030 Agenda for Sustainable Development. New York, USA: United Nations.
- Ushiwata, S., H. Sasa, and K. Inubushi. 2007. “Influence of Steam-treated Grass Clipping on Grass Growth, Drainage Water Quality and Soil Microbial Properties in a Simulation of Green Course.” Soil Science and Plant Nutrition 53 (4): 489–498. doi:10.1111/j.1747-0765.2007.00149.x.
- Wada, H., K. Inubushi, Y. Uehara, and Y. Takai. 1981. “Easily Decomposable Organic Matter in Paddy Soil (I), Relationship between Nitrogen Mineralization and Total Soil Nitrogen.” Japanese Journal of Soil Science and Plant Nutrition 52 (3): 246-252. (in Japanese with English summary). http://agris.fao.org/agris-search/search.do?recordID=JP19820731461
- Watanabe, T., M. Kimura, and S. Asakawa. 2006. “Community Structure of Methanogenic Archaea in Paddy Field Soil under Double Cropping (Rice–wheat).” Soil Biology & Biochemistry 38: 1264–1274. doi:10.1016/j.soilbio.2005.09.020.
- Witt, C., J. L. Gaunt, C. C. Galicia, J. C. G. Ottow, and H.-U. Neue. 2000. “A Rapid Choloroform-fumigation Extraction Method Foe Measuring Soil Microbial Biomass Carbon and Nitrogen in Flooded Rice Soils.” Biology and Fertility of Soils 30: 510–519. doi:10.1007/s003740050030.
- Xu, X., K. Inubushi, and K. Sakamoto. 2006. “Effect of Vegetations and Temperature on Microbial Biomass Carbon and Metabolic Quotients of Temperate Volcanic Forest Soils.” Geoderma 136: 310–319. doi:10.1016/j.geoderma.2006.03.045.
- Xu, X., L. Han, Y. Wang, and K. Inubushi. 2007. “Influence of Vegetation Types and Soil Properties on Microbial Biomass Carbon and Metabolic Quotients in Temperate Volcanic and Tropical Forest Soils.” Soil Science and Plant Nutrition 53 (4): 430–440. doi:10.1111/j.1747-0765.2007.00146.x.
- Yagi, K., H. Tsuruta, K. Kanda, and K. Minami. 1996. “Effect of Water Management on Methane Emission from a Japanese Rice Paddy Field: Automated Methane Monitoring.” Global Biogeochemical Cycles 10 (2): 255–267. doi:10.1029/96GB00517.
- Yamashita, K., H. Honjo, M. Nishida, M. Kimura, and S. Asakawa. 2014. “Estimation of Microbial Biomass Potassium in Paddy Field Soil.” Soil Science and Plant Nutrition 60: 512–519. doi:10.1080/00380768.2014.919237.
- Yoshida, M., S. Ishii, S. Otsuka, and K. Senoo. 2009. “Temporal Shifts in Diversity and Quantity of nirS and nirK in a Rice Paddy Field Soil.” Soil Biology & Biochemistry 41: 2044–2051. doi:10.1016/j.soilbio.2009.07.012.
- Yoshikawa, S., and K. Inubushi. 1995. “Characteristics of Microbial Biomass and Soil Organic Matter in Newly Reclaimed Wetland Rice Soils.” Biology and Fertility of Soils 19 (4): 292-296. doi:10.1007/BF00336097.
- Zaman, M., H. J. Di, K. Sakamoto, S. Goto, H. Hayashi, and K. Inubushi. 2002b. “Effect of Sewage Sludge Compost and Chemical Fertilizer Applications on Microbial Biomass and N Mineralization Rates.” Soil Science and Plant Nutrition 48: 195–201. doi:10.1080/00380768.2002.10409191.
- Zaman, M., K. C. Cameron, H. J. Di, and K. Inubushi. 2002a. “Changes in Mineral N, Microbial Biomass and Enzyme Activities in Different Soil Depths after Surface Applications of Dairy Shed Effluent and Chemical Fertilizer.” Nutrient Cycling in Agroecosystems 63 (2–3): 275–290.
- Zaman, M., M. Matsushima, S. X. Chang, K. Inubushi, L. Nguyen, S. Goto, F. Kaneko, and T. Yoneyama. 2004. “Nitrogen Mineralization, N2O Production and Soil Microbiological Properties as Affected by Long-term Applications of Sewage Sludge Composts.” Biology and Fertility of Soils 40: 101–109. doi:10.1007/s00374-004-0746-2.
- Zheng, X., Z. Zhou, Y. Wang, J. Zhu, Y. Wang, J. Yue, Y. Shi, et al. 2006. “Nitrogen-regulated Effects of Free-air CO2 Enrichment on Methane Emissions from Paddy Rice Fields.” Global Change Biology 12: 1717–1732. doi:10.1111/j.1365-2486.2006.01199.x.