ABSTRACT
The objective of this study was to investigate the current soil properties in lowland paddy fields in Peninsular Malaysia and to assess the long-term changes in the soil fertility status during 50 years after the Green Revolution. Forty paddy fields were selected close to the study sites surveyed in 1965 and grouped based on six physiographic environments: the brackish swamp (including acid sulfate soils), the brackish alluvium, the freshwater swampand the riverine alluvium in the west coast, and the riverine alluvium and the beach ridges interspersed with swales in the east coast. Soil samples from the depth of 0–15 cm were analyzed for the physicochemical properties. Despite similar fertilizer application rates over Peninsular Malaysia, several differences were found in the current soil properties between different physiographic environments, such as 1) higher levels of clay fraction, 1.4 nm minerals, CEC, exchangeable bases and available Si as well as Mg- and Na-rich status in the brackish environments, 2) higher levels of available N in the riverine alluvium environment in the east coast , and 3) the excessive P accumulation in the acid sulfate soils. The long-term changes well appeared in the dramatic increase of P availability and the alleviation of soil acidity. In addition, the composition of exchangeable bases changed toward Ca-rich and Mg-low status. Large reduction in soil organic matter was found in the swamp environments while those in the riverine alluvium environment in the east coast were increased. It could be concluded that despite the successful increase in rice yield after the Green Revolution, the long-term changes in the paddy soil fertility showed positive and negative aspects depending on physiographic environments. Appropriate fertilizer application schemes taking into account different soil characteristics in different physiographic environments should be required to achieve both efficient, sustainable rice production and environmental conservation.
1. Introduction
In the tropical Asian countries, the Green Revolution, the development of a package of new technologies such as high yielding varieties, abundant agrochemical inputs, irrigation systems and mechanization, initiated in 1960s and has induced the dramatic increase in rice production to sustain increasing population. As described later, in Peninsular Malaysia (formerly called as Malaya or West Malaysia), the Green Revolution started before the independence of Malaysia in 1963, relatively earlier among the countries (Kuchiba Citation1978; Hill Citation2012), and nowadays the farming system seems the most developed and intensive because of the generous support policy of the government (DOA Citation2016).
Kawaguchi and Kyuma (Citation1969; Citation1977) surveyed lowland paddy soils in Peninsular Malaysia in 1965 and then assessed soil fertility of lowland paddy fields among 10 tropical Asian countries including Peninsular Malaysia. Their factor analysis ranked the soils in Peninsular Malaysia in 1965 the most superior in the organic matter factor among the countries. This was due to the accumulation of soil organic matter (SOM) under rather swampy conditions in the west coast. The phosphorus availability factor was intermediate among the countries where the levels of available P and HCl-extractable P were absolutely low. The soils were the most inferior in the inherent potentiality factor related to the nature and amount of clay and base status. Within Peninsular Malaysia, the soils in the west coast were more fertile than those in the west coast.
We can assume that the soils in the lowland paddy fields in 1960s reported by Kawaguchi and Kyuma (Citation1977) were unaffected yet, or least affected if any, by the Green Revolution and, therefore, that the soil fertility status of today must reflect the changes during 50 years after the Green Revolution. Limited studies have been conducted so far from a long-term viewpoint (for example, Ali et al. Citation1997 in Bangladesh; Darmawan et al. Citation2006 in Indonesia). In 2010s, we carried out a series of follow-up studies to assess soil fertility status in Thailand (Yanai et al. 2020) and Peninsular Malaysia (the present study) as well as the Philippines and Bangladesh (forthcoming). The results of these countries will be compared elsewhere. Thus, the objective of this study was to investigate the current soil properties in lowland paddy fields in Peninsular Malaysia and to assess the long-term changes in soil fertility status after the Green Revolution.
2. Materials and methods
2.1. Natural setting in Peninsular Malaysia
Because natural setting of Peninsular Malaysia is described in detail elsewhere (Kawaguchi and Kyuma Citation1969; Asharaf, Othman, and Ishak Citation2018), brief information is given here on the west and east coasts where most of the study sites were located (). The tropical rainforest climate (Af) of the Köppen’s system dominates in both coasts except the tropical monsoon climate (Am) in the northern region of the west coast. The mean annual temperature is around 27 to 28 °C. Because of the influences of southwest and northeast monsoons, the annual precipitation are different between the coasts: the precipitation in the west coast is about 2000 mm with two peaks during October to November and during April to May, while that in the east coast exceeds 3000 mm with a single peak between November and January.
Figure 1. Map of study sites. Although the sites were named as M1 to M43, ‘M’ is omitted in this figure. Three sites were not surveyed: M25 was converted to an urban area in 2017, while M26 and M37 had been listed but not investigated in Kawaguchi and Kyuma (Citation1969). M9 and M27 were acid sulfate soils. BRIS is the local name used for the landform of sandy beach ridges interspersed with swales
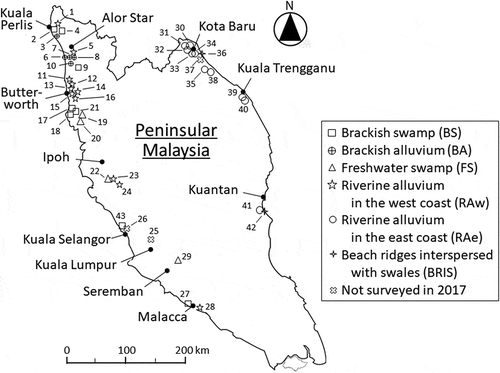
Extensive parts of the west coast are originally freshwater or brackish swamps with peat accumulation, formed by the mid-Holocene high see-level stand and the subsequent regression processes. Therefore, lowland paddy soils in the west coast are derived mainly from swamp sediments as well as recent brackish (or marine) alluvial deposits. Otherwise, the soils develop from riverine alluvium which is originated from Paleozoic metasedimentary rocks or Triassic granitoids in upper mountainous or hilly areas. Meanwhile, along the east coast, sandy beach ridges interspersed with swales (locally called as BRIS) are well-developed due to strong wave action of the South China Sea. However, riverine alluviums distributing around several river deltas such as Kelantan and Pahang Rivers are more commonly used for rice production than BRIS. Acid sulfate soils occasionally exist along both west and east coasts (Shamshuddin Citation2006).
2.2. Rice cultivation in Peninsular Malaysia
The history of lowland rice cultivation of Peninsular Malaysia is relatively short with substantial expansion after the 18th to 19th century (Hill Citation2012). Rice self-sufficiency rates were less than 30% during the colonial era. The Green Revolution with new technologies such as a non-photoperiodic rice variety (Mahsuri) started before the independence of Malaysia in 1963 (Kuchiba Citation1978; Hill Citation2012). However, during the late 1950s to early 1960s, the technologies had not yet spread over farmers. Rainfed single cropping during the rainy season was still common (Moritani Citation1965; Takaya, Fukui, and Yamada Citation1978). Moritani (Citation1965) reported that despite the recommendation rate around 30, 35–70 and 15 kg ha−1 for N, P2O5 and K2O, the actual fertilizer application was far low and, occasionally, only Christmas Island rock phosphate and bat guano were used because of their cheap prices. Manure was not applied. Harvest residues were burned away to control rice stem borers. Under swamp environments in the west coast, slashing vegetation with Tajak (a heavy metal blade) and transplanting with Kuku Kanbin (a metal stick with a bifurcated tip to transplant rice seedlings) were adopted specifically to work amid entangled plant debris and peat materials (Takaya, Fukui, and Yamada Citation1978).
Nowadays, the farming practices and techniques are rather homogenized over Peninsular Malaysia (DOA (Department of Agriculture, Peninsular Malaysia) Citation2016; Kari Citation2018). A series of irrigation schemes has been carried out since the implementation of the Muda and Kemubu Irrigation Schemes in the late 1960s, leading to rapid expansion of double-cropping systems. Based on these schemes, 10 granary areas are designated with the aim at consolidating and intensifying rice production. About 90% of paddy fields are irrigated during the off-season, the season which is administratively defined for rice planting commencing between 1st March and 31st July which depend on irrigation while the main-season is between 1st August and 28/29th February without depending on irrigation. Land preparation and harvesting are completely mechanized while the direct-broadcasting method for sowing is adopted in 90% of the fields. The subsidy scheme provides gratis fertilizers and agrochemicals, starting with partially subsidizing in 1960’s, followed by completely subsidizing since the 1979/1980 main season. According to the statistics (DOA (Department of Agriculture, Peninsular Malaysia) Citation2016), the average input of N, P and K in the granary areas in the 2014/2015 main season amount to 122, 67 and 66 kg ha−1 (N, P2O5 and K2O) with the standard application of compound fertilizer (17.5:15.5:10 or 17:20:10 as N, P2O5 and K2O) and urea as well as the additional input of compound fertilizer (17:3:20 as N, P2O5 and K2O with 2 MgO, 1 B, 0.5 Cu and 0.2 Zn) and fortified organic fertilizer (5:5:5). Liming materials (mainly, ground magnesium limestone, GML) are also applied extensively. The rice yield in Peninsular Malaysia increases from about 2 t ha−1 during 1960s to 4.56 t ha−1 in 2015 with the production of 2.78 million ton and the harvested area of 5.21 × 105 ha (DOA (Department of Agriculture, Peninsular Malaysia) Citation2016).
2.3. Study sites and soils
For the present study, 40 paddy fields were selected close to the study sites in 1965 (Kawaguchi and Kyuma Citation1969) and the same names of M1 to M43 were given to the corresponding sites (). Three sites were excluded: M25 has been converted to an urban area in 2017, while M26 and M37 had been listed but not investigated in their study. The locations of the sites are given in , roughly overlapping with the granary areas designated by the government, except for M27 and M28 in Malacca, M35 in Kelantan and M39 and M40 in Terengganu. Following Kawaguchi and Kyuma (Citation1969), the study sites were grouped based on the physiographic environments: the brackish swamp (BS), the brackish alluvium (BA), the freshwater swamp (FS) and the riverine alluvium (RAw) in the west coast, and the riverine alluvium (RAe) in the east coast except for M36 and M42 (). The latter sites were grouped into the new category of BRIS in the present study because of their particular quartz-sandy nature (Roslan et al. Citation2010; Mohd Yusoff et al. Citation2017), although grouped in RA and BS, respectively, in Kawaguchi and Kyuma (Citation1969).
Table 1. Study sites and their physiographic environments
The soil classes had not been sufficiently identified in Kawaguchi and Kyuma (Citation1969) because of the limitation due to undeveloped classification systems for paddy soils at that time. Based on their soil data including morphological description, many of the soils seemed to be Aquepts in the USDA Soil Taxonomy (Soil Survey Staff Citation2010). M9 and M27 in BS were acid sulfate soils (Kawaguchi and Kyuma Citation1969), which could be classified into Sulfaquepts or Tropaquepts (Shamshuddin Citation2006). Meanwhile, two BRIS soils (M36 and M42) had been classified into Rudua and Rusila series in the Malaysian classification system at that time (Kawaguchi and Kyuma Citation1969), which correspond to Arenic Alorthods and Typic Psammaquents, respectively (Roslan et al. Citation2010).
According to our interview to the landowners, the management practices such as fertilizer application were similar to those above-mentioned (DOA (Department of Agriculture, Peninsular Malaysia) Citation2016). The rice varieties such as MR219, 220, 263 and 297 were planted. All fields were double-cropped except for the case of M35 and M40 with rainfed single-cropping. Burning practices of harvest residues were occasionally conducted and the cinders of harvest residues were left in the fields. Otherwise, the residues were left and plowed into soils before the next cropping.
2.4. Soil sampling and analysis
Soil sampling was conducted in September for the west coast and in November for the east coast in 2017. Although rice farming calendars vary between localities depending on the officially set irrigation schedules, most of the fields upon sampling were at the stage immediately before harvest to that after paddling. Meanwhile, the soils in Kawaguchi and Kyuma (Citation1969) were sampled during January and February in 1965 after rice harvest in the single-cropping system.
Soil samples were collected from the depth of 0–15 cm at 5 points within each field, mixed well to make one composite sample and air-dried to pass through a 2 mm mesh sieve. The analytical methodology was based on Kawaguchi and Kyuma (Citation1969) and described in Yanai et al. (2020). pH (H2O) and EC were measured by the grass electrode method. Total C and N were determined by the dry combustion method. Exchangeable bases (Ca, Mg, K and Na) were extracted with 1 M CH3COONH4 solution at pH7.0 and determined by atomic absorption spectrophotometry, and subsequently, the concentration of adsorbed NH4+ was determined spectrophotometrically as cation exchange capacity (CEC). Total P was determined with an Energy-Dispersive X-Ray Fluorescence spectrometer while available P was with the Bray II method. HCl-extractable P and available Si were extracted with 0.2 M HCl and an acetate buffer, respectively, and each determined spectrophotometrically. Available N was determined by an evaluation of NH4+ concentration extracted with 2 M KCl solution by the steam distillation method after two-week incubation at 40 °C under the submerged condition. Soil particle distribution was determined by the sieving and pipette method. Clay mineralogy were determined by the X-ray diffraction (XRD) method and 0.7, 1.0 and 1.4 nm mineral composition was computed based on their relative peak areas of the XRD charts. Other detected minerals such as quartz were not concerned in the present study.
All of the measurements except total C and N from the depth of 0–15 cm in the present study were directly compared with those from the most upper A horizon tabled in Kawaguchi and Kyuma (Citation1969) as the references after unit conversion if necessary. Because their study had adopted the Tyurin method for total C and the Kjeldahl method for total N, these data were multiplied by the factors of 1.2 and 1.1, respectively, for the comparison, which were determined via the dry combustion method based on a preliminary experiment (Yanai et al. 2020). These values were reasonably close to the reported average values of 1.25 for C (Nelson and Sommers Citation1996) and 1.10 for N (Bremner Citation1996).
2.5. Statistical analysis
Data analysis were performed by using a statistical software (Excel Statistics ver. 3.10, BellCurve, Tokyo). Soil properties between physiographic environments were compared by using the Tukey’s multiple comparison at p= 0.05 after one-way analysis of variance (ANOVA) if homogeneity of variance was verified by the Bartlett test, otherwise the data were log-transformed before ANOVA. The soil properties were compared with those in Kawaguchi and Kyuma (Citation1969) by using the paired t-test, or the Wilcoxon signed rank test in case that normality was not verified. A standardized multiple regression analysis with the stepwise selection method was performed to identify the CEC determinants between total C, clay contents and the percentages of 1.0 nm and 1.4 nm minerals. The percentage of 0.7 nm minerals was omitted from the analysis because of a highly negative correlation between 0.7 nm and 1.4 nm minerals (r = −0.94**).
3. Results
3.1. Soil chemical properties in 2017
The overall averages of chemical properties for 40 soil samples are given in the left row in with their coefficient of variation (CV). The soils were fine-textured and acidic with predominant Ca and Mg among exchangeable bases. The contents of total C and total N seemed to be relatively high in terms of tropical farmland soils. Those of available P and HCl-extractable P exceeded 100 mg kg−1 but with the largest CV values. Clay mineralogy was predominated by 0.7 nm minerals followed by 1.4 nm minerals.
Table 2. Averages and the coefficients of variation (CV) for chemical properties of 40 soil samples in 2017 and the comparison between physiographic environments
For physiographic environments, noticeable differences were found between brackish environments (BS and BA) and freshwater environments (FS, RAw and RAe) (). The levels of pH, EC, CEC and available Si were significantly higher in the brackish environments than in the freshwater environments. The contents of exchangeable bases and base saturation were higher in the brackish environments than RAe in the east coast. Those in FS and RAw in the west coast were intermediate. The percentage of Mg to the sum of exchangeable bases (data not given in ) tended to be high in the brackish environments (33 and 43% in BS and BA) compared with the freshwater environments (20 to 26%). The level of available N was the highest in RAe. Clay mineral composition could be characterized by a higher percentage of 1.4 nm minerals in the brackish environments and by the predominance of 0.7 nm minerals in the freshwater environments.
There was no difference in total C and N, three forms of P and particle size distribution between the physiographic environments except for the content of coarse sand. Extremely high values of available P and HCl-extractable P were detected for acid sulfate soils (1394 and 2223 mg P kg−1 in M9 and 572 and 661 mg P kg−1 in M27, respectively) while the other properties were almost within the range of the BS soils. Omitting these extremes, the averages of available P and HCl-extractable P in BS were 99 and 110 mg kg−1, respectively, but significant differences were not found between physiographic environments. Two BRIS soils in the east coast (M36 and M42) were rather sandy and high in exchangeable Mg compared with the other RAe soils. The M36 soil showed relatively high base and P status with base saturation exceeding 150%.
3.2. Comparison of soil properties between 1965 and 2017
compares soil properties between 1965 and 2017. As a whole, the levels of pH, CEC, total P, available P, HCl-extractable P and available Si significantly increased from 1965 to 2017, while those of total C and the C/N ratio decreased. Although the sum of exchangeable bases did not change, the levels of Ca and K significantly increased while those of Mg and Na decreased. The percentages of Ca, Mg and Na to the sum of exchangeable bases also showed similar trends of 40 to 62%, 44 to 28% and 11 to 5%, respectively (p< 0.01; data not given in ). Clay mineral composition slightly but significantly increased in 0.7 nm minerals and decreased in 1.0 nm and 1.4 nm minerals.
Table 3. Trend of soil properties over 50 years after Green Revolution
Regarding the physiographic environments, the trends are described here irrespective of statistical context because of large variations in some properties within each environment as well as the limited sample numbers (FS and BA). The trends of pH, the C/N ratio, exchangeable Ca, Mg and Na, available P, HCl-extractable P, available Si and 0.7 nm and 1.4 nm minerals in each physiographic environment were mostly similar to the whole trend. Meanwhile, the soils in the swamp environments (BS and FS) showed decreasing trends in total C and N from 1965 to 2017. The decreasing ratios of total C and N were 41 and 39% in BS and 64 and 64% in FS, respectively. In these swamp environments, available N tended to decrease while an increasing tendency in both coarse and fine sand fractions was found. Meanwhile, in the alluvial environments (BA, RAw and RAe), there were no appreciable changes in total C and N. However, regarding RAe in the east coast, it should be noted that the contents of total C and N in M41 were extremely high in 1965 (136.8 and 8.6 g kg−1) and decreased to 35.2 and 3.37 g kg−1 in 2017, respectively. Omitting these extremes, the averages in RAe significantly increased from 18.0 to 30.0 g kg−1 and from 1.7 to 2.7 g kg−1, respectively (p< 0.05 and 0.01). Regardless of M41, the soils in RAe showed significant increases in CEC, exchangeable K and available N.
Acid sulfate soils (M9 and M27) showed dramatic increase in pH from 3.6 to 6.7 and 3.4 to 6.1, respectively, from 1965 to 2017. Available P and HCl-extractable P also considerably increased from 26 to 1394 mg P kg−1 and from 47 to 2223 mg P kg−1 in M9 and from 8 to 572 mg P kg−1 and 6 to 661 mg P kg−1 in M27, respectively. The other properties of these soils were changed similarly to those of the other BS soils. Meanwhile, the BRIS soils (M36 and M42) showed double levels of available N (117 and 134 mg N kg−1 in ), compared with the values of 51 and 66 mg N kg−1 in 1965, respectively. The opposite trend was found in the other properties: SOM and nutrients increased in M36 and decreased in M42 from 1965 to 2017 (for example, 7.2 to 16.2 and 92.6 to 24.5 g kg−1 of total C and 2.76 to 5.76 and 12.7 to 3.33 cmolc kg−1 of the sum of exchangeable bases). Soil texture considerably changed in M42 with 12 to 61% of sand and 53 to 19% of clay contents.
4. Discussion
4.1. Differences in soil properties in 2017 between physiographic environments
Despite rather homogenized rice farming practices, the current soil properties could be distinguished between the brackish environments (BS and BA) and the freshwater environments (FS, RAw and RAe) (). The latter could be sub-divided in the west coast (FS and RAw) and the east coast (RAe).
The soils in the brackish environments (BS and BA) were high in CEC and 1.4 nm clay minerals. Similar mineralogy of recent sediments was reported in the Bangkok plain of Thailand (Hattori Citation1972). A standardized multiple regression analysis for all soils produced an equation: CEC = 0.52 x clay** + 0.37 × 1.4 nm minerals** (adjusted R2 = 0.62**; ** indicates p< 0.01). illustrates the relationship between the percentages of clay and 1.4 nm minerals in terms of different CEC levels. The higher CEC in the brackish environments could be ascribed to the combined effect of clay contents mostly exceeding 40% and higher percentages of 1.4 nm minerals. Although SOM is generally regarded as the key factor for the amount of negative charges, the regression analysis did not select total C as the explanatory variable. Furthermore, a Pearson’s correlation of total C with CEC was significant for all soils but with a low coefficient (r= 0.36, p< 0.05), and was insignificant for the soils in each physiographic environment. The charge characteristics of SOM might vary depending on the combined effect of the qualities of organic matter added as manure and the cinders of harvest residues as well as the alteration processes of SOM in different physiographic environments.
Figure 2. Relationship between clay content and percentage of 1.4 nm clay minerals with different CEC under different physiographic environments. BS: Brackish swamp, BA: Brackish alluvium, FS: Fresh-water swamp, and RAw: Riverine alluvium, RAe: Riverine alluvium, BRIS: Beach ridges interspersed with swales
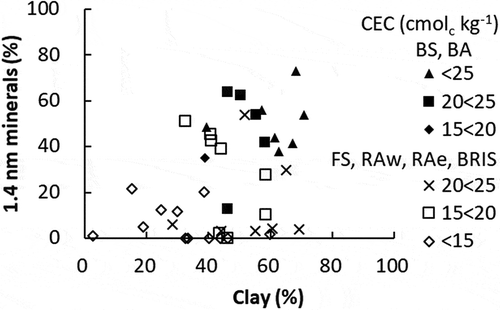
A negative correlation was found between the percentages of Mg and Ca to the sum of exchangeable bases (); r= −0.93 for all soils, p< 0.01) with the 80 to 90% share by these elements in the sum of exchangeable bases, indicating the strong competition between Mg and Ca for negative charge sites. As discussed below, the trend of these elements over the 50 years seemed to be the result of the replacement of soil Mg with Ca contained in the P fertilizers and/or GML. Meanwhile, the soils in the brackish environments (BS and BA) showed less acidic nature with higher contents of exchangeable bases and base saturation than the other soils. Several soils in the brackish environments were high in both exchangeable Mg and Na ()), suggesting the influence of saline water. For Malaysian paddy fields, despite well-designed irrigation systems, drainage facilities are not so effective because their main purpose is not complete but sufficient drainage to make heavy-machinery works possible (Ezaki, Yashima, and Thavaraj Citation1976; Fukui and Takaya Citation1978). Therefore, because of intrinsically brackish environments of BS and BA, the influences of saline water entering to soils upon flooding or the intrusion of saline groundwater might remain due to poor internal drainage.
Figure 3. Relationships (a) between the percentages of Mg and Ca to the sum of exchangeable bases and (b) between exchangeable Mg and Na. BS: Brackish swamp, BA: Brackish alluvium, **: p < 0.01
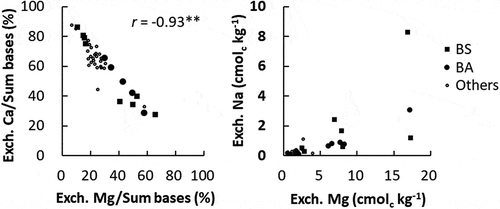
The higher levels of available Si in the brackish environments might be ascribable to the presence of readily weatherable minerals as the source of Si in the tropical climate, judging from appreciable amount of 1.4 nm clay minerals and relatively high contents of silt fraction (Kawaguchi and Kyuma Citation1977). Regarding acid sulfate soils (M9 and M27) of BS, remarkably high levels of available P and HCl-extractable P were due to the cumulative effect of applied P fertilizer, which was provided as a subsidy to improve this problematic soil with a recommendation rate of 125 kg P2O5 ha−1 (Asharaf, Othman, and Ishak Citation2018).
In the freshwater environments (FS, RAw and RAe), clay mineral composition dominated by 0.7 nm minerals seemed to be largely influenced by alluvial sediments loaded through river systems from the upland regions where highly weathered ultisols and oxisols distributed extensively. Compared with the other soils, the RAe soils in the east coast were remarkably higher in available N despite the similar levels of total C and N and C/N. Although this phenomenon had also been reported in Kawaguchi and Kyuma (Citation1969), no proper reason could be found.
4.2. Changes in lowland paddy soils in Peninsular Malaysia after the Green Revolution
The long-term changes after the Green Revolution were detected on the lowland paddy soils (). The most prominent was the increased P availability compared with P-deficient status in 1965, which could be ascribed to plentiful P fertilizer application as well as the alleviation of soil acidity by using the liming materials and P fertilizers. The similar result was obtained in Thailand (Yanai et al. 2020).
Reduction in total C and N and available N was obvious in the swamp environments (BS and FS), indicating that plant debris and peat materials accumulated under anaerobic condition had been progressively decomposed due to repeating wet and dry cycles due to the establishment of irrigation and drainage systems. In contrast, in RAe in the east coast except M41, the levels of total C and N and available N increased, indicating the improvement of SOM status probably due to manure application as well as increasing input of harvest residues. Thus, while the difference in SOM between physiographic environments found in 1965 was reduced in 2017 (), the current intensive rice farming system had the contrast effects on the SOM level depending on physiographic environments. Meanwhile, the alleviation of soil acidity might be ascribable to the reduction in acidic SOM constituents, especially for the swamp environments (BS and FS), as well as continuous application of liming materials. In case of acid sulfate soils, another possible reason is a washout effect of produced acid materials by irrigation water.
The significant increase in the overall average of CEC could be ascribed mainly to its increase in RAe in the east coast. The trend of CEC in the other physiographic environments in the west coast were not clear although the reduction of SOM was found, especially in the swamp environments (BS and FS). This might be explainable partially by less significance of SOM to CEC as discussed above. Despite heavy fertilizer application over 50 years, the sum of exchangeable bases was not appreciably increased probably due to the limited development of the negative charges under acidic condition in many of the soils with little changes in CEC level. The composition of exchangeable bases significantly altered toward Ca-rich and Mg- and Na-low status, indicating the replacement of soil Mg and Na with Ca added as the P fertilizers or GML. The reduction of Mg was in accordance with the case in Thailand (Yanai et al. 2020). It is noteworthy that the soils in the brackish environments (BS and BA) persisted Mg- and Na-rich in nature to some extent. Despite plentiful fertilizer application, the increase in exchangeable K was not obvious, found only for all soils and the RAe soils in the east coast where the level of the K had been the lowest in 1965. This result for the K was different from the case in Thailand where the significant increase in exchangeable K was observed (Yanai et al. 2020). Due to more humid condition in Peninsular Malaysia than Thailand, the substantial loss of fertilized K might occur, although the harvest residues were returned to soils with or without burning practices as mentioned above. The level of available Si significantly increased in BS and RAe, for which the increase in pH might be one of the reasons. The increasing tendencies of 0.7 nm minerals might be the result of the outflow of original 1.4 nm clay minerals and the recruitment of 0.7 nm minerals from the weathered soils in upland regions. The trend of coarse-texturization in the swamp environments (BS and FS) could be explained by changes in sedimentation mode through repeated heavy-machinery works and irrigation. The coarse-texturization was also observed in Thailand (Yanai et al. 2020).
Regarding the BRIS soils, the Rudua series distributing on the ridge are characterized by the feature of so-called tropical podzols with extremely quarts-sandy texture and the concomitant extremely poor fertility (Roslan et al. Citation2010; Mohd Yusoff Citation2017). Increasing tendency of SOM and nutrients in M36 suggested that such inferior soil fertility was improved through repeated fertilizer and manure application. Meanwhile, the Rusila series distribute at back-swamps between the ridges, showing peat characteristics (Roslan et al. Citation2010). Because of this nature, M42 had been grouped in BS in Kawaguchi and Kyuma (Citation1969). While the reducing trend of SOM was similar to the other BS soils, the M42 soil showed considerable coarse-texturization. One possible reason for this is the inflow of sand from the surrounding sandy ridges to the low-lying swamp area due to the disturbance such as the conversion from forest to farmland and residential patches.
5. Conclusion
The present study assessed the long-term changes in soil fertility status in lowland paddy fields in Peninsular Malaysia during 50 years after the Green Revolution. The most prominent change was the increase in P availability. Soil acidity was also alleviated. The composition of exchangeable bases changed toward Ca-rich and Mg-low status. Large reduction in SOM was found in the swamp environments in the west coast while those in the east coast were increased. Meanwhile, several differences were found between the current soil properties in different physiographic environments such as 1) higher levels of clay fraction, 1.4 nm minerals, CEC, exchangeable bases and available Si as well as Mg- and Na-rich status in brackish environments, 2) higher levels of available N in the riverine alluvium environment in the east coast, and 3) excessive accumulation of P in the acid sulfate soils. The rice yield in Peninsular Malaysia has been successfully increased owing to the most developed and intensive farming systems among the tropical Asia, generously supported by the government. However, based on the results of the present study, soil fertility status in the paddy fields showed positive and negative aspects in the long-term changes during the 50 years, depending on physiographic environments. Instead of the current rather homogenized system over Peninsular Malaysia, appropriate fertilizer application schemes taking into account different soil characteristics in different physiographic environments should be required to achieve both efficient, sustainable rice production and environmental conservation.
Acknowledgments
The authors would like to express their deep gratitude to Dr. Kazutake Kyuma, Emeritus Professor of Kyoto University, and Dr. Toshiyuki Wakatsuki, Emeritus Professor of Shimane University, for their constructive suggestions on this research and their offer of the detail information on the sampling sites, analytical methods and analytical data of the soil samples investigated. This research was partly funded by the Japan Society for the Promotion of Science through a Grant-in-Aid for Scientific Research (B) (overseas academic) (No. 15H05247).
Disclosure statement
No potential conflict of interest was reported by the authors.
References
- Ali, M. M., S. M. Saheed, D. Kubota, T. Masunaga, and T. Wakatsuki. 1997. “Soil Degradation during the Period 1967–1995 in Bangladesh.” Soil Science and Plant Nutrition 43 (4): 863–878. doi:10.1080/00380768.1997.10414653.
- Asharaf, M. A., R. Othman, and C. F. Ishak, eds. 2018. Soils of Malaysia. Boca Raton: CEC Press.
- Bremner, J. M. 1996. “Nitrogen-Total.” In Methods of Soil Analysis Part 3 – Chemical Methods, edited by D. L. Sparks, 1085–1121. Wisconsin: SSSA Book series: 5. Soil Science Society of America and American Society of Agronomy.
- Darmawan, K. Kyuma, A. Saleh, H. Subagjo, T. Subagjo, and T. Wakatsuki. 2006. “Effect of green revolution technology from 1970 to 2003 on sawah soil properties in Java, Indonesia: I. Carbon and nitrogen distribution under different land management and soil types.” Soil Science and Plant Nutrition 52 (5): 634–644.
- DOA (Department of Agriculture, Peninsular Malaysia). 2016. “Paddy Production Survey Report Malaysia. Main Season 2104/2015”. Putrajaya.
- Ezaki, K., S. Yashima, and S. H. Thavaraj. 1976. “Need for the Drainage of Paddy Fields to Enable Farm Mechanization for Double-cropping of Rice in the Muda Irrigation Project - A Joint Study by the Muda Agricultural Development Authority, Malaysia, and the Tropical Agriculture Research Center, Japan.” JARQ 10 (3): 119–124.
- Fukui, H., and Y. Takaya. 1978. “Some Ecological Observations on Rice-growing in Malaysia.” South East Asian Studies 16 (2): 189–197.
- Hattori, T. 1972. “Some Properties of Recent Sediments in the Bangkok Plain of Thailand.” South East Asian Studies 10 (2): 321–334.
- Hill, R. D. 2012. Rice in Malaya. A Study in Historical Geography. Singapore: NUS Press.
- Kari, F. 2018. “Evaluation of Agricultural Subsidies and the Welfare of Rice Farmers.” Malaysia Agricultural Subsidies Report 2018. Kuala Lumpur: IDEAS.
- Kawaguchi, K., and K. Kyuma. 1969. Lowland Rice Soils in Malaya. Kyoto: Center for Southeast Asian Studies.
- Kawaguchi, K., and K. Kyuma. 1977. Paddy Soils in Tropical Asia. Their Material Nature and Fertility. Honolulu: University Press of Hawaii.
- Kuchiba, M. 1978. “Socio-economic Changes in a Malay-growing Community (Padang Lalang) in Kudah.” Southeast Asian Studies 16 (2): 22–39.
- Mohd Yusoff, K. H., A. Abdu, K. Sakurai, S. Tanaka, and Y. Kang. 2017. “Influence of Agricultural Activity on Soil Morphological and Physicochemical Properties on Sandy Beach Ridges along the East Coast of Peninsular Malaysia.” Soil Science and Plant Nutrition 63 (1): 55–66. doi:10.1080/00380768.2016.1255127.
- Moritani, M. 1965. “Rice Culture Techniques.” South East Asian Studies 2 (3): 30–37. in Japanese.
- Nelson, D. W., and L. E. Sommers. 1996. “Total Carbon, Organic Carbon, and Organic Matter.” In Methods of Soil Analysis Part 3 – Chemical Methods, edited by D. L. Sparks, 961–1010. Wisconsin: SSSA Book series: 5. Soil Science Society of America and American Society of Agronomy.
- Roslan, I., J. Shamshuddin, C. I. Fauziah, and A. R. Anuar. 2010. “Occurrence and Properties of Soils on Sandy Beach Ridges in the Kelantan–Terengganu Plains, Peninsular Malaysia.” Catena 83 (1): 55–63. doi:10.1016/j.catena.2010.07.004.
- Shamshuddin, J. 2006. Acid Sulfate Soils in Malaysia. Serdang: UPM Press.
- Soil Survey Staff. 2010. Keys to Soil Taxonomy (11th Ed.). Washington DC: USDA Natural Resources Conservation Service.
- Takaya, Y., H. Fukui, and I. Yamada. 1978. “Ecology of Traditional Padi Fanning in West Malaysia.” South East Asian Studies 16 (2): 133–158.
- Yanai, J., M. Hirose, S. Tanaka, K. Sakamoto, A. Nakao, K. Dejbhimon, A. Sriprachote, P. Kanyawongha, T. Lattirasuvan, and S. Abe. 2020. “Changes in Paddy Soil Fertility in Thailand Due to the Green Revolution during the Last 50 Years.” Soil Science and Plant Nutrition, in press.