ABSTRACT
Silicon (Si) is a beneficial element for plants, which helps to mitigate various biotic and abiotic stresses. Since the last review on Si published in this journal in 2004, great progress has been made in understanding transport system of Si in different plant species. The discovery of two different transporters for Si (Lsi1 and Lsi2) in rice led to intensive investigation of Si transporters in other plant species. Lsi1 belongs to the Nodulin 26-like intrinsic proteins (NIPs) subfamily in the aquaporin (AQP) family and functions as an influx transporter for Si. By contrast, Lsi2 belongs to the anion transporter superfamily and functions as an efflux transporter of Si. They are polarly localized at the distal and proximal sides, respectively, of both exodermis and endodermis of rice roots and are required for efficient uptake of Si. So far, homologs of Lsi1 and Lsi2 are identified not only in monocots, but also in dicots, which greatly differ in Si accumulation in the aboveground parts. However, the expression pattern, cell-type-specific expression, and polar localization of these transporters differ with plant species. In this review, we focus on recent progress in Si transporters identified in different plant species. We link these transporters with an accumulation of Si in different plant species in terms of expression pattern, cell-type-specific expression, polar localization of these transporters and propose three uptake systems of Si in different plant species. We also provide the perspectives toward a better understanding of Si transport system in different plant species and discuss its essentiality for plant growth.
1. Introduction
Silicon (Si) is the most abundant mineral element in the earth’s crust (Marschner Citation2012), therefore, all plants rooting in soil contain a significant amount of Si in their bodies (Ma and Takahashi Citation2002). However, Si has not been listed as an essential element for plants due to a lack of evidence for the involvement of Si in metabolism (Epstein Citation1999; Marschner Citation2012). Nerveless, the beneficial effects of Si have been observed in several plant species (for review, see Ma Citation2004), which are characterized by alleviating various stresses, including biotic (plant pathogen, and insect pest) and abiotic stresses (drought stress, salt stress, metal toxicity, lodging, nutrient imbalance) (Ma and Takahashi Citation2002). Since our last review paper on the beneficial effect of Si published in this journal in 2004, great progress has been made in understanding transport system of Si in plants, especially in rice. In this paper, we focus on transport and accumulation of Si in different plant species and propose three modes for Si uptake.
2. Beneficial effects of Si revealed by a mutant defective in Si accumulation
It is well known that the beneficial effects of Si are more obvious under stress conditions (Tamai and Ma Citation2008). However, the real beneficial effects of Si might have been underestimated so far. In hydroponic culture, although it is easy to remove Si from the nutrient solution, the experiments are usually performed in a greenhouse with fewer stresses. On the other hand, in the field experiments with various stresses, the beneficial effect of Si is usually compared between plants with and without the application of Si fertilizers. Since Si is relatively rich in soil, the real effect of Si on plant growth and development is difficult to be evaluated. However, isolation of a rice mutant defective in Si uptake and accumulation makes it possible to evaluate the real effect of Si on rice under field conditions. When the low silicon rice 1 (lsi1) mutant and its wild-type (WT) rice were grown in the same field, the growth of the mutant was severely damaged ()). The leaves were attached by insects ()) and the panicles were infected by various pathogens, resulting in the coloration of the panicles and low fertility ()). From four-year field experiments, the grain yield of the mutant was only less than 10% of the wild-type rice (Tamai and Ma Citation2008), indicating the importance of Si for rice growth and productivity. This is the reason why Si is recognized as an ‘Agronomically essential element’ for rice in Japan (Ma and Takahashi Citation2002), and Si fertilizers have been routinely applied into paddy fields for stable and high rice production. For other plant species, when similar low-Si mutants are available in the future, evaluation of real Si effects could also be made in the field as rice.
Figure 1. Beneficial effects of silicon in rice revealed by lsi1, a mutant defective in Si uptake and accumulation. (a) Overview of wild-type rice (WT) and lsi1 grown in a paddy field. (b) Effect of Si on insect damage. (c) Effect of Si on pathogen infection in the panicles. (d) Effect of Si on grain yield of WT and mutant grown in a paddy field in different years. Data are adapted from Tamai and Ma (Citation2008)
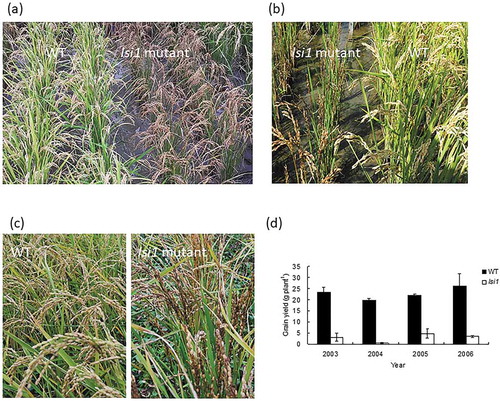
By using the lsi1 mutant, progress has also been made in understanding the mechanisms for Si-improved nutrient imbalance. For example, Si supply alleviated manganese (Mn) toxicity by decreasing both the uptake and root-to-shoot translocation of Mn. Si supply resulted in down-regulation of OsNramp5 in rice roots, a transporter gene for Mn uptake (Che et al. Citation2016). Si supply also down-regulates the expression of inorganic phosphorus (Pi) transporter gene, OsPT6 to suppress Pi uptake under high P condition (Hu et al. Citation2018). Recently, Si supply decreased zinc (Zn) uptake by down-regulating the expression of Zn transporter OsZIP1 required for Zn uptake (Huang and Ma Citation2020). Interestingly, these effects of Si on gene expression were caused by Si accumulated in the shoots, but not in the roots and solution. For the beneficial effects in other plant species, refer to recent review papers (e.g. Coskun et al. Citation2019a; Frew et al. Citation2018).
3. Variation of Si accumulation in different plant species
There is a wide variation of Si accumulation in the shoots among plant species, ranging from 0.1% to 10% Si in the dry weight (Ma and Takahashi Citation2002). Based on the Si concentration in the shoots and Si/Ca ratio, plants have been classified into three types; low-, intermediate- and high-Si accumulators (Ma and Takahashi Citation2002). Low Si accumulators (excluder) are plants having less than 0.5% Si concentration in the shoots and <0.5 of Si/Ca ratio, while intermediate Si accumulators have Si concentration of 0.5%- 1% and 0.5–1.0 of Si/Ca ratio, and high accumulators have Si concentration higher than 1.0% and >1.0 of Si/Ca ratio (Ma and Takahashi Citation2002). The typical Si accumulator is rice (Oryza sativa), which accumulates to up to Si 10% of shoot dry weight, while the typical excluder is tomato with less than 0.2% Si (Takahashi, Ma, and Miyake Citation1990). There is a characteristic distribution of Si accumulation in the plant kingdom. Si is highly accumulated in Bryophyta, as well as Lycopsida and Equisetopsida, but hardly accumulated in Gymnospermae (Ma and Takahashi Citation2002). In Angiospermae, Gramineae and Cyperaceae show particularly high Si accumulation (Ma and Takahashi Citation2002; Hodson et al. Citation2005). Most dicots are unable to accumulate Si and belong to the low accumulator. However, some notable exceptions exist such as Asteraceae, Urticaceae, and Cucurbitaceae (Adatia and Besford Citation1986; Cherif et al. Citation1992; Hodson et al. Citation2005). These wide variations in Si accumulation have been attributed to the ability of the roots to take up Si (Ma Citation2004), which will be discussed later.
4. Transport system of Si in rice
Plants take up Si in the form of silicic acid [Si(OH)4], a non-charged molecule at a pH under 9. Most soil usually contains Si in the solution between 0.1 mM and 0.6 mM (Ma and Takahashi Citation2002). Following uptake, more than 95% of Si is rapidly translocated to the shoots in rice (Ma and Takahashi Citation2002), although this ratio may be low in other plant species. Since Si is present in the form of silicic acid in the xylem sap in rice and wheat (Mitani, Ma, and Iwashita Citation2005; Casey et al. Citation2003), it seems that this form is translocated from the roots to the shoots, followed by unloading from the xylem to the parenchyma cells at the leaf blade and leaf sheath. However, in the leaves, most Si is present in the form of silica, SiO2 (Ma and Takahashi Citation2002). With water loss due to transpiration, silicic acid is gradually concentrated and finally polymerized to silica. Silica is deposited beneath the cuticle of leaves, forming cuticle-silica double layers, and inside of particular cells of leaf epidermis such as motor cells, forming silica bodies in rice (Ma and Takahashi Citation2002). These Si depositions in the aerial part of the plants play a crucial role in protecting the plants from various stresses.
To transport Si from soil to different organs in the ground-above parts, transporters are required at least for the uptake, root-to-shoot translocation, and distribution. These transporters have not been identified until the discovery of the first Si transporter, Lsi1 in rice (Ma et al. Citation2006). According to the textbook, there are three different membrane transport proteins; channel, carrier, and pump (Assmann Citation2015). Since these proteins facilitate the transport of ions and molecules from one side to the other side across the membrane, all of them could be considered as ‘transporters’. The first transporter gene for Si, Lsi1 (OsLsi1), was cloned from a rice mutant defective in Si uptake (Ma et al. Citation2006). OsLsi1 is a channel-type transporter, which belongs to the Nod26-like major intrinsic protein (NIP) subfamily of aquaporin-like proteins (Ma et al. Citation2006). OsLsi1 is a plasma membrane-localized protein and shows bi-directional transport activity for silicic acid. OsLsi1 is polarly localized at the distal side of the exodermis and endodermis of the roots ()) (Ma et al. Citation2006; Yamaji and Ma Citation2007).
Figure 2. Schematic presentation of Si uptake system in different plant species. (a) Active Si uptake system in rice. Si uptake is cooperatively mediated by Lsi1 and Lsi2, which are polarly localized at the distal and proximal side, respectively, of both exodermis and endodermis. (b) Active Si uptake system in other Si-accumulating species. Si uptake in these species such as barley is also cooperatively mediated by Lsi1 and Lsi2, but localized at different cell layers. HvLsi1 shows polar localization at the distal side, but HvLsi2 does not. (c) Passive uptake system. Si uptake in these plant species such as cucumber, is mediated by CsLsi1 expressed at all cell layers without polarity, and CsLsi2 at the endodermis without polarity. (d) Rejective uptake system. This system is employed by low Si-accumulator such as tomato, which has functional Lsi1, but lacks Lsi2. Arrows with different colors indicate transport processes mediated by different transporters, apoplastic flow, and symplastic flow of Si. See the main text for more details
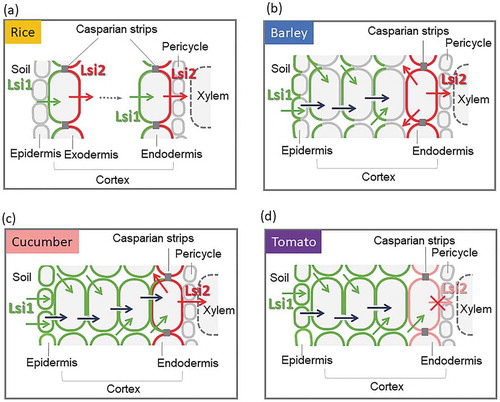
The second Si transporter, OsLsi2 was identified using the same approach as OsLsi1. However, OsLsi2 belongs to a putative anion transporter family without any similarity with OsLsi1 (Ma et al. Citation2007). In contrast to the passive transport of OsLsi1, OsLsi2 shows efflux transport activity for silicic acid, which is driven by the proton gradient (Ma et al. Citation2007). Similar to OsLsi1, OsLsi2 is also localized at the plasma membrane of the exodermis and endodermis of the roots, but shows different polar localization. OsLsi2 is localized at the proximal side ()) (Ma et al. Citation2007). Knockout of either OsLsi1 or OsLsi2 results in a significant decrease of Si uptake by the roots, indicating that both of them are required for high Si uptake (Tamai and Ma Citation2003; Ma et al. Citation2006, Citation2007).
Following the uptake of Si by OsLsi1 and OsLsi2 in the root, Si is translocated to the shoot by transpirational mass flow in the xylem and unloaded by OsLsi6, a sole homolog of OsLsi1 in rice genome (Yamaji, Mitani, and Ma Citation2008). Lsi6 is polarly localized at the adaxial side of the xylem parenchyma cells in the leaf sheaths and leaf blades (Yamaji, Mitani, and Ma Citation2008)(). The knockout of Lsi6 affects the silica deposition pattern in the leaf blades and sheaths (Yamaji, Mitani, and Ma Citation2008). HvLsi6 in barley also shows a similar role as OsLsi6 in Si distribution (Yamaji et al. Citation2012).
Figure 3. Schematic presentation of Si distribution in shoot of rice. In leaves, Si in the xylem sap is unloaded by Lsi6, but transporters for further deposition at specific cells are unidentified. In nodes, Si in the xylem of enlarged vascular bundle is first unloaded by Lsi6 localized at the xylem transfer cells, followed by releasing Si toward diffuse vascular bundles by OsLsi2 polarly localized at the bundle sheath cell layer and OsLsi3 localized in the parenchyma tissues between enlarged vascular bundles and diffuse vascular bundles. Arrows with different colors indicate transport processes mediated by different transporters and symplastic flow of Si
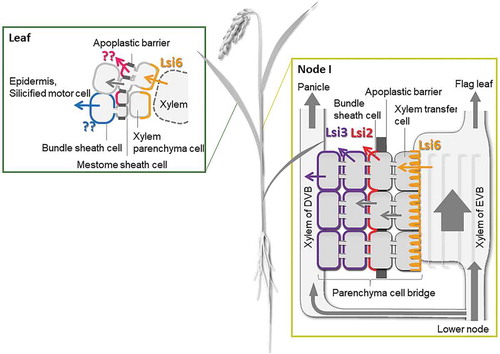
At the reproductive stage, most Si will be distributed to the grains (mainly accumulated in the husk). This distribution is mediated by three different Si transporters; Lsi6, Lsi2, and Lsi3 in rice(), which are localized at different cell layers of node I (Yamaji and Ma Citation2009; Yamaji et al. Citation2015; ). OsLsi6 at the xylem transfer cells of the enlarged vascular bundles (EVB) is responsible for the unloading of Si from the xylem, while OsLsi2 and its homolog OsLsi3 localized at the bundle sheath and parenchyma bridge cells are responsible for further transfer of Si to the diffuse vascular bundles. Knockout of OsLsi6, OsLsi2, or OsLsi3 results in the decreased distribution of Si to the panicles but increased Si to the flag leaf (Yamaji et al. Citation2015).
5. Uptake system of Si in different plant species
Based on physiological studies, three different modes of Si uptake have been proposed for plants having different degrees of Si accumulation, that is, active, passive, and rejective uptake (Takahashi, Ma, and Miyake Citation1990; Mitani and Ma Citation2005). On the other hand, homologs of rice Lsi1 and Lsi2 have been identified in several other plant species such as barley, maize, pumpkin, cucumber, tomato, tobacco, sorghum, and others (). They show different expression patterns, tissue/cell-type-specific expression, and polarity of transporters as summarized in . To link these transporters with uptake modes and accumulation, we propose three uptake systems in different plant species, corresponding to the different uptake modes.
Table 1. Characteristics of Si transporters in different plant species and correlation with their Si accumulation. Dark gray indicates that Si transporters have not been identified, while light gray indicates that data on transport activity are not available
5.1. Active uptake system of Si
This uptake system is represented by Si-accumulating plants such as barley, maize, rice, and wheat. The uptake is mediated by both influx and efflux transporters of Si; Lsi1 and Lsi2. Furthermore, both of them or either of them show polar localization ()). In rice, the roots have distinct anatomy, which is characterized by two Casparian strips at the exodermis and endodermis, and formation of aerenchyma in most of cortex cells ()). Therefore, Si (in soil solution) is first imported into the exodermis by Lsi1 polarly localized at the distal side, followed by exporting Si by Lsi2 polarly localized at the proximal side to the aerenchyma ()). Si in the apoplastical space is further transported into and out of the endodermis by Lsi1 and Lsi2, respectively, toward the xylem ()). Lsi1 is a bidirectional channel that mediates concentration-dependent passive transport of Si, whereas Lsi2 is an energy-dependent active efflux transporter (Ma et al. Citation2006, Citation2007; Mitani, Yamaji, and Ma Citation2008). Therefore, polar localization of Lsi1 and Lsi2 cooperatively forms an efficient directional transport system for Si uptake, resulting in high Si accumulation in the shoots (Ma and Yamaji Citation2015). Mathematical modeling showed that the polar localization of Lsi1 and Lsi2 at the same cell and the presence of Casparian strips are important factors for active Si uptake system (Sakurai et al. Citation2015). In fact, lack of Casparian strip at the endodermis significantly decreases Si uptake (Wang et al. Citation2019).
In barley and maize, Si in the soil solution is taken up by HvLsi1 or ZmLsi1, respectively, which is polarly localized in epidermal, hypodermal, and cortical cells ()). Unlike rice roots, since there is no developed aerenchyma in the roots of barley and maize, Si is transported to the endodermis by the symplastic pathway. Releasing Si to the stele is mediated by HvLsi2 or ZmLsi2, respectively, localized at the endodermis ()). This uptake system also results in high Si accumulation in the shoots but the efficiency is lower compared with rice. The difference in Si accumulation could be attributed to the expression level and polar localization of Lsi1 and Lsi2, Casparian strip, and root structure ()). The expression level of OsLsi1 and OsLsi2 is probably higher than that of HvLsi1/ZmLsi1 and HvLsi2/ZmLsi2 although a detailed comparison remains to be examined. Although HvLsi1 and ZmLsi1 also show polar localization at the distal side ()), HvLsi2 and ZmLsi2 do not show polar localization (Mitani et al. Citation2009b) and they are not localized at the same cells. These differences result in less Si uptake and accumulation in the shoots compared with rice.
TaLsi1 from wheat was also identified (Montpetit et al. Citation2012), but its cell-type-specific expression is unknown. Lsi2-like transporter has not yet been identified in wheat. Based on Si accumulation, its uptake system might be similar to that in barley and maize.
5.2. Passive transport system
This uptake system is employed by plants having intermediate Si accumulation such as cucumber and pumpkin ()). CmLsi1 and CmLsi2 in pumpkin, CsLsi1, and CsLsi2 in cucumber have been partially characterized (; Mitani et al. Citation2011; Mitani-Ueno, Yamaji and Ma Citation2011; Sun et al. Citation2017, Sun et al. Citation2018). CmLsi1 and CsLsi1 are expressed in most root cells (Mitani et al. Citation2011; Sun et al. Citation2017), while CsLsi2 are in endodermal cells (Sun et al. Citation2018). However, different from Lsi1 and Lsi2 in Si-accumulating plants as described above, most of them do not show polar localization at the cortex cells except CsLsi1 (Mitani et al. Citation2011; Sun et al. Citation2017, Citation2018). CsLsi1 shows the polar localization at the endodermis (Sun et al. Citation2017). Furthermore, Lsi1 and Lsi2 in these plant species are not localized at the same cell (Mitani et al. Citation2011; Sun et al. Citation2017, Citation2018). Since Lsi1 is a bi-directional transporter (Mitani, Yamaji, and Ma Citation2008), lack of polar localization and co-existence of Lsi1 and Lsi2 in these species result in low efficiency of Si uptake.
5.3. Rejective uptake system
This uptake system is used by non-Si accumulators such as tomato. A previous study showed that Lsi1 in tomato is not functional due to longer distance between asparagine–proline–alanine (NPA) domains (Deshmukh et al. Citation2015). However, a recent study showed that tomato roots also have a functional Lsi1, SlLsi1 (Sun et al. Citation2019; (d)). SlLsi1 is localized at all root cells but does not show polar localization (Sun et al. Citation2019). The low accumulation in tomato seems to result from the absence of Lsi2-type transporter. This is supported by a recent finding; when a functional Lsi2 from cucumber was expressed in tomato, increased Si accumulation was observed in the transgenic tomato plants (Sun et al. Citation2019). Therefore, the presence of Lsi2 is very important for Si uptake and accumulation by actively pumping out Si toward the xylem.
In addition to Si transporters described above, other homologs of Lsi1 and Lsi2 have also been isolated in date palm (PdNIP2) (Bokor et al. Citation2019), potato (StLsi1 and StLsi2) (Vulavala et al. Citation2016), tobaccos (Nicotiana tabacum (NtLsi1) and Nicotiana sylvestris (NsLsi1)) (Zellner et al. Citation2019; Coskun et al. Citation2019b)), soybean (GmNIP2-1 and GmNIP2-2) (Deshmukh et al. Citation2013), and sorghum (SbLsi1) (Markovich et al. Citation2019). However, since the cell-type-specific expression and polar localization of these transporters have not been investigated, their uptake system remains to be examined in the future.
Also, several corresponding homologs of Lsi1 in Equisetum arvense (horsetail), EaNIP3s have been experimentally demonstrated to be permeable to Si (Grègoire et al. Citation2012). Horsetail is one of the highest Si accumulating primitive plants (Hodson et al. Citation2005), which requires Si to complete its lifecycle (Chen and Lewin Citation1969). Different from the selectivity filter of Lsi1 in higher plants consisting of Gly (G), Ser (S), Gly (G), and Arg (R), EaNIP3s have a distinct one composing of Ser (S), Thr (T), Ala (A), and Arg (R) (Grègoire et al. Citation2012; Ma and Yamaji Citation2015). On the other hand, two putative Si efflux transporters, EaLsi2-1, and EaLsi2-2 have also been identified in horsetail (Vivancos et al. Citation2016). Since their cell-type-specific expression is not clear, it is still unknown which uptake system makes horsetail to accumulate high Si.
6. Future perspectives
Based on previous findings, Si accumulation in the shoots seems to be determined by the ability of the roots to take up Si. Si uptake in different plant species is controlled by several factors including the presence and expression level of Lsi1 and Lsi2, their polar localization, and cell-type-specific expression in addition to the presence of Casparian strip in the roots. Since the discovery of OsLsi1 in 2006 and OsLsi2 in 2007, several homologs have been identified and functionally characterized as described above, however, Si transporters in most plant species have not been identified. With rapid progress in genome sequencing of different plant species, many candidates of both Lsi1- and Lsi2-like Si transporters are found in diverse plant species. Functional characterization of these Si transporters in terms of expression level, cell-type-specific expression, and polar localization in different plant species will help better understanding of the uptake system of Si in different plant species.
The expression level of Lsi1 and/or Lsi2 differs with plant species, which affects the Si uptake and accumulation. Furthermore, the response of their expression to Si differs from plant species. For example, expression of Lsi1 in rice is down-regulated by Si supply (Ma et al. Citation2006), whereas that in barley, maize, pumpkin, and tomato is unaffected (Chiba et al. Citation2009; Mitani, Yamaji, and Ma Citation2009a; Mitani et al. Citation2011; Sun et al. Citation2019). Si reduces the expression of Lsi2 in rice, barley, and maize, but increases that in pumpkin and cucumber, while that in tomato and potato is unaffected (Ma et al. Citation2007; Yamaji and Ma Citation2011; Mitani et al. Citation2009b; Mitani-Ueno, Yamaji, and Ma Citation2011b; Sun et al. Citation2018, Citation2019; Vulavala et al. Citation2016). However, the mechanism responsible for the regulation of expression of Lsi1 and Lsi2 is unknown. Recently, it was reported that Si-induced down-regulation of Lsi1 and Lsi2 in rice roots is triggered by Si deposited in the shoots (Mitani-Ueno, Yamaji, and Ma Citation2016) and the promoter region of Lsi1 between −327 and −292 seems to be involved in this down-regulation, however, the exact mechanism for this remains to be further investigated.
The most characteristic feature of Lsi1 and Lsi2 is the polar localization found in some species such as OsLsi1 and OsLsi2 in rice and HvLsi1 in barley (Ma et al. Citation2006, Citation2007; Chiba et al. Citation2009). Polar localization is important for efficient Si uptake by facilitating directional transport of Si from soil solution to the root stele. However, the mechanism for the polar localization of Lsi1 and Lsi2 is still unknown. Furthermore, some Si transporters such as Lsi1 in tomato, Lsi2 in barley, do not show the polar localization. This means that some specific mechanism is present for polar localization in specific cells of rice and barley although the polarity of most Si transporters reported is unknown.
Lsi1 and Lsi2 in rice are also able to transport arsenite (As(III) due to the similarity of chemical property between silicic acid and arsenite (Ma et al. Citation2008). This is one of the reasons for high As accumulation in rice (Ma et al. Citation2008). Arsenic is a carcinogenic metalloid, therefore it is important to reduce As in rice grain. For this purpose, the mechanisms responsible for the substrate specificity of Si transporters need to be investigated in the future. Although selectivity filter ar/R has been considered to be important for transport substrate specificity of Lsi1 (Mitani-Ueno et al. Citation2011a), it is still exactly unknown how this filter affects the substrate specificity. Analysis of the crystal structure of Si transporters in the future may give some clues for manipulating transport substrates.
The beneficial effects of Si are associated with Si accumulation in the aboveground parts. However, as described above, most plant species are not able to accumulate high Si due to the lack of an efficient transport system, therefore failing to benefit from Si. In the future, introduction of an efficient Si uptake system to non-Si accumulating crops may increase crop productivity through enhancing resistance to multiple stresses.
In addition to transporters for Si uptake in roots, transporters for distribution of Si in different organs and tissues are also required. For example, in rice, Si is deposited in some specific cells such as motor cells and beneath the cuticle (Ma and Takahashi Citation2002; Prychid, Rudall, and Gregory Citation2004). However, only a few transporters for Si distribution have been identified. It is unknown whether homologs of Lsi1 and Lsi2 or novel Si transporters are involved in this process.
Due to the universal existence of Si, its impact on plant growth has not been paid much attention for a long time. However, given that transporters specific for Si is present in a wide variety of plant species and that evidence on beneficial effects of Si is accumulating in different plant species, it is a time to discuss whether Si should be considered as an essential element for plant growth and development. This will challenge the criteria for essential elements proposed by Arnon and Stout in 1939.
Disclosure statement
No potential conflict of interest was reported by the authors.
Additional information
Funding
References
- Adatia, M. H., and R. T. Besford. 1986. “The Effect of Silicon in Cucumber Plants Grown in Recirculating Nutrient Solution.” Annals of Botany 58 (3): 343–351. doi:10.1093/oxfordjournals.aob.a087212.
- Assmann, S. M. 2015. “Solute Transport.” In Plant Physiology and Development, edited by L. Taiz, E. Zeiger, I. M. Moller, and A. Murphy, 143–168. 6th ed. Massachusetts: Sinauer Associates, .
- Bokor, B., M. Soukup, M. Vaculík, P. Vd’ačný, M. Weidinger, I. Lichtscheidl, S. Vávrová, et al. 2019. “Silicon Uptake and Localisation in Date Palm (Phoenix Dactylifera) -a Unique Association with Sclerenchyma.” Frontiers in Plant Science 10: 988. doi:10.3389/fpls.2019.00988.
- Casey, W. H., S. D. Kinrade, C. T. G. Knight, D. W. Rains, and E. Epstein. 2003. “Aqueous Silicate Complexes in Wheat, Triticum Aestivum L.” Plant, Cell & Environment 27 (1): 51–54. doi:10.1046/j.0016-8025.2003.01124.x.
- Che, J., N. Yamaji, J. F. Shao, J. F. Ma, and R. F. Shen. 2016. “Silicon Decreases Both Uptake and Root-to-shoot Translocation of Manganese in Rice.” Journal of Experimental Botany 67 (5): 1535–1544. doi:10.1093/jxb/erv545.
- Chen, C., and J. Lewin. 1969. “Silicon as a Nutrient Element for Equisetum Arvense.” Canadian Journal of Botany 47 (1): 125–131. doi:10.1139/b69-016.
- Cherif, M., N. Benhamou, J. G. Menzies, and R. R. Bélanger. 1992. “Silicon Induced Resistance in Cucumber Plants against Pythium Ultimum.” Physiological and Molecular Plant Pathology 41 (6): 411–425. doi:10.1016/0885-5765(92)90053-X.
- Chiba, Y., N. Mitani, N. Yamaji, and J. F. Ma. 2009. “HvLsi1 Is a Silicon Influx Transporter in Barley.” The Plant Journal 57 (5): 810–818. doi:10.1111/j.1365-313X.2008.03728.x.
- Coskun, D., R. Deshmukh, H. Sonah, J. G. Menzies, O. Reynolds, J. F. Ma, H. J. Kronzucker, and R. R. Bélanger. 2019a. “The Controversies of Silicon’s Role in Plant Biology.” New Phytologist 221 (1): 67–85. doi:10.1111/nph.15343.
- Coskun, D., R. Deshmukh, H. Sonah, S. M. Shivaraj, R. Frenette-Cotton, L. Tremblay, P. Isenring, and R. R. Bélanger. 2019b. “Si Permeability of a Deficient Lsi1 Aquaporin in Tobacco Can Be Enhanced through a Conserved Residue Substitution.” Plant Direct 3 (8): e00163. doi:10.1002/pld3.163.
- Deshmukh, R. K., J. Vivancos, V. Guérin, H. Sonah, C. Labbé, F. Belzile, and R. R. Bélanger. 2013. “Identification and Functional Characterization of Silicon Transporters in Soybean Using Comparative Genomics of Major Intrinsic Proteins in Arabidopsis and Rice.” Plant Molecular Biology 83 (4–5): 303–315. doi:10.1007/s11103-013-0087-3.
- Deshmukh, R. K., J. Vivancos, G. Ramakrishnan, V. Guérin, G. Carpentier, H. Sonah, C. Labbé, P. Isenring, F. Belzile, and R. R. Bélanger. 2015. “A Precise Spacing between the NPA Domains of Aquaporins Is Essential for Silicon Permeability in Plant.” The Plant Journal 83 (3): 489–500. doi:10.1111/tpj.12904.
- Epstein, E. 1999. “Silicon.” Annual Review of Plant Physiology and Plant Molecular Biology 50: 641–664. doi:10.1146/annurev.arplant.50.1.641.
- Frew, A., L. A. Weston, O. L. Reynolds, and G. M. Gurr. 2018. “The Role of Silicon in Plant Biology: A Paradigm Shift in Research Approaches.” Annals of Botany 121 (7–8): 1265–1273. doi:10.1093/aob/mcy009.
- Grègoire, C., W. Rémus-Borel, J. Vivancos, C. Labbé, F. Belzile, and R. R. Bélanger. 2012. “Discovery of a Multigene Family of Aquaporin Silicon Transporters in the Primitive Plants Equisetum Arvense.” The Plant Journal 72 (2): 320–330. doi:10.1111/j.1365-313X.2012.05082.x.
- Hodson, M. J., P. J. White, A. Maed, and M. R. Broadley. 2005. “Phylogenetic Variation in the Silicon Composition of Plants.” Annals of Botany 96 (6): 1027–1046. doi:10.1093/aob/mci255.
- Hu, A. Y., J. Che, J. F. Shao, K. Yokosho, X. Q. Zhao, R. F. Shen, and J. F. Ma. 2018. “Silicon Accumulated in the Shoots Results in Down-regulation of Phosphorus Transporter Gene Expression and Decrease of Phosphorus Uptake in Rice.” Plant and Soil 423: 317–325. doi:10.1007/s11104-017-3512-6.
- Huang, S., and J. F. Ma. 2020. “Silicon Suppresses Zinc Uptake through Down‐regulating Zinc Transporter Gene in Rice.” Physiologia Plantarum. doi:10.1111/ppl.13196.
- Ma, J. F. 2004. “Role of Silicon in Enhancing the Resistance of Plants to Biotic and Abiotic Stresses.” Soil Science and Plant Nutrition 50 (1): 11–18. doi:10.1080/00380768.2004.10408447.
- Ma, J. F., and E. Takahashi. 2002. Soil, Fertilizer, and Plant Silicon Research in Japan. Amsterdam: Elsevier.
- Ma, J. F., K. Tamai, N. Yamaji, N. Mitani, S. Konishi, M. Katsuhara, M. Ishiguro, Y. Murata, and M. Yano. 2006. “A Silicon Transporter in Rice.” Nature 440 (7084): 688–691. doi:10.1038/nature04590.
- Ma, J. F., and N. Yamaji. 2015. “A Cooperative System of Silicon Transport in Plants.” Trends in Plant Science 20 (7): 435–442. doi:10.1016/j.tplants.2015.04.007.
- Ma, J. F., N. Yamaji, N. Mitani, K. Tamai, S. Konishi, T. Fujiwara, M. Katsuhara, and M. Yano. 2007. “An Efflux Transporter of Silicon in Rice.” Nature 448 (7150): 209–212. doi:10.1038/nature05964.
- Ma, J. F., N. Yamaji, N. Mitani, X. Y. Xu, Y. H. Su, S. McGrath, and F. J. Zhao. 2008. “Transporters of Arsenite in Rice and Their Role in Arsenic Accumulation in Rice Grain.” Proceedings of the National Academy of Sciences of the United States of America 105 (29): 9931–9935. doi:10.1073/pnas.0802361105.
- Markovich, O., S. Kumar, D. Cohen, S. Addadi, E. Fridman, and R. Elbaum. 2019. “Silicification in Leaves of Sorghum Mutant with Low Silicon Accumulation.” Silicon 11: 2385–2391. doi:10.1007/s12633-015-9348-x.
- Marschner, P. 2012. Mineral Nutrition of Higher Plants. 3rd ed. London: Academic Press.
- Mitani, M., J. F. Ma, and T. Iwashita. 2005. “Identification of Silicon Form in the Xylem of Rice (Oryza Sativa L.).” Plant & Cell Physiology 46 (2): 279–283. doi:10.1093/pcp/pci018.
- Mitani, N., Y. Chiba, N. Yamaji, and J. F. Ma. 2009b. “Identification and Characterization of Maize and Barley Lsi2-like Silicon Efflux Transporters Reveals a Distinct Silicon Uptake System from that in Rice.” The Plant Cell 21 (7): 2133–2142. doi:10.1105/tpc.109.067884.
- Mitani, N., and J. F. Ma. 2005. “Uptake System of Silicon in Different Plant Species.” Journal of Experimental Botany 56 (414): 1255–1261. doi:10.1093/jxb/eri121.
- Mitani, N., N. Yamaji, Y. Ago, K. Iwasaki, and J. F. Ma. 2011. “Isolation and Functional Characterization of an Influx Silicon Transporter in Two Pumpkin Cultivars Contrasting in Silicon Accumulation.” The Plant Journal 66 (2): 231–240. doi:10.1111/j.1365-313X.2011.04483.x.
- Mitani, N., N. Yamaji, and J. F. Ma. 2008. “Characterization of Substrate Specificity of a Rice Silicon Transporter Lsi1.” Pflügers Archiv - European Journal of Physiology 456 (4): 679–686. doi:10.1007/s00424-007-0408-y.
- Mitani, N., N. Yamaji, and J. F. Ma. 2009a. “Identification of Maize Silicon Influx Transporters.” Plant & Cell Physiology 50 (1): 5–12. doi:10.1093/pcp/pcn110.
- Mitani-Ueno, N., N. Yamaji, and J. F. Ma. 2011b. “Silicon Efflux Transporters Isolated from Two Pumpkin Cultivars Contrasting in Si Uptake.” Plant Signaling & Behavior 6 (7): 991–994. doi:10.4161/psb.6.7.15462.
- Mitani-Ueno, N., N. Yamaji, and J. F. Ma. 2016. “High Silicon Accumulation in the Shoot Is Required for Down-regulating the Expression of Si Transporter Genes in Rice.” Plant & Cell Physiology 57 (12): 2510–2518. doi:10.1093/pcp/pcw163.
- Mitani-Ueno, N., N. Yamaji, F. J. Zhao, and J. F. Ma. 2011a. “The Aromatic/arginine Selectivity Filter of NIP Aquaporins Plays a Critical Role in Substrate Selectivity for Silicon, Boron, and Arsenic.” Journal of Experimental Botany 62 (12): 4391–4398. doi:10.1093/jxb/err158.
- Montpetit, J., J. Vivancos, N. Mitani-Ueno, N. Yamaji, W. Rémus-Borel, F. Belzile, J. F. Ma, and R. R. Bélanger. 2012. “Cloning, Functional Characterization and Heterologous Expression of TaLsi1, a Wheat Silicon Transporter Gene.” Plant Molecular Biology 79 (1–2): 35–46. doi:10.1007/s11103-012-9892-3.
- Prychid, C. J., P. J. Rudall, and M. Gregory. 2004. “Systematics and Biology of Silica Bodies in Monocotyledons.” The Botanical Review 69: 377–440.
- Sakurai, G., A. Satake, N. Yamaji, N. Mitani-Ueno, M. Yokozawa, F. Feugier, and J. F. Ma. 2015. “In Silico Simulation Modeling Reveals the Importance of the Caspian Strip for Efficient Silicon Uptake in Rice Roots.” Plant & Cell Physiology 56 (4): 631–639. doi:10.1093/pcp/pcv017.
- Sun, H., Y. Duan, N. Mitani-Ueno, J. Che, J. Jia, J. Liu, J. Guo, J. F. Ma, and H. Gong. 2019. “Tomato Roots Have a Functional Silicon Influx Transporter but Not a Functional Silicon Efflux Transporter.” Plant and Cell Environment 43 (3): 732–744. doi:10.1111/pce.13679.
- Sun, H., Y. Duan, X. Qi, L. Zhang, H. Huo, and H. Gong. 2018. “Isolation and Functional Characterization of CsLsi2, a Cucumber Silicon Efflux Transporter Gene.” Annals of Botany 122 (4): 641–648. doi:10.1093/aob/mcy103.
- Sun, H., J. Guo, Y. Duan, T. Zhang, H. Huo, and H. Gong. 2017. “Isolation and Functional Characterization of CsLsi1, Silicon Transporter Gene in Cucumis Sativus.” Physiologia Plantarum 159 (2): 201–214. doi:10.1111/ppl.12515.
- Takahashi, E., J. F. Ma, and Y. Miyake. 1990. “The Possibility of Silicon as an Essential Element for Higher Plants.” Comments on Agricultural and Food Chemistry 2 (2): 99–102.
- Tamai, K., and J. F. Ma. 2003. “Characterization of Silicon Uptake by Rice Roots.” New Phytologist 158 (3): 431–436. doi:10.1046/j.1469-8137.2003.00773.x.
- Tamai, K., and J. F. Ma. 2008. “Reexamination of Silicon Effects on Rice Growth and Production under Field Conditions Using a Low Silicon Mutant.” Plant and Soil 307 (1): 21–27. doi:10.1007/s11104-008-9571-y.
- Vivancos, J., R. Deshmukh, C. Grégoire, W. Rémus-Borel, F. Belzile, and R. R. Bélanger. 2016. “Identification and Characterization of Silicon Efflux Transporters in Horsetail (Equisetum Arvense).” Journal of Plant Physiology 200: 82–89. doi:10.1016/j.jplph.2016.06.011.
- Vulavala, V. K., R. Elbaum, U. Yermiyahu, E. Fogelman, A. Kumar, and I. Ginzberg. 2016. “Silicon Fertilization of Potato: Expression of Putative Transporters and Tuber Skin Quality.” Planta 243 (1): 217–229. doi:10.1007/s00425-015-2401-6.
- Wang, Z., N. Yamaji, S. Huang, X. Zhang, M. Shi, S. Fu, G. Yang, J. F. Ma, and J. Xia. 2019. “OsCASP1 Is Required for Casparian Strip Formation at Endodermal Cells of Rice Roots for Selective Uptake of Mineral Elements.” The Plant Cell 31 (11): 2636–2648. doi:10.1105/tpc.19.00296.
- Yamaji, N., Y. Chiba, N. Mitani-Ueno, and J. F. Ma. 2012. “Functional Characterization of a Silicon Transporter Gene Implicated in Silicon Distribution in Barley.” Plant Physiology 160 (3): 1491–1497. doi:10.1104/pp.112.204578.
- Yamaji, N., and J. F. Ma. 2007. “Spatial Distribution and Temporal Variation of the Rice Silicon Transporter Lsi1.” Plant Physiology 143 (3): 1306–1313. doi:10.1104/pp.106.093005.
- Yamaji, N., and J. F. Ma. 2009. “A Transporter at the Node Responsible for Inter-vascular Transfer of Silicon in Rice.” The Plant Cell 21 (9): 2878–2883. doi:10.1105/tpc.109.069831.
- Yamaji, N., and J. F. Ma. 2011. “Further Characterization of a Rice Silicon Efflux Transporter, Lsi2.” Soil Science and Plant Nutrition 57 (2): 259–264. doi:10.1080/00380768.2011.565480.
- Yamaji, N., N. Mitani, and J. F. Ma. 2008. “A Transporter Regulating Silicon Distribution in Rice Shoots.” The Plant Cell 20 (5): 1381–1389. doi:10.1105/tpc.108.059311.
- Yamaji, N., G. Sakurai, N. Mitani-Ueno, and J. F. Ma. 2015. “Orchestration of Three Transporters and Distinct Vascular Structures in Node for Intervascular Transfer of Silicon in Rice.” Proceedings of the National Academy of Sciences of the United States of America 112 (36): 11401–11406. doi:10.1073/pnas.1508987112.
- Zellner, W., L. Lutz, S. Khandekar, and S. Leisner. 2019. “Identification of NtNIP2;1: An Lsi1 Silicon Transporter in Nicotiana Tabacum.” Journal of Plant Nutrition 42 (9): 1028–1035. doi:10.1080/01904167.2019.1589500.