ABSTRACT
Mycorrhizal symbiosis may help plants to compensate the water deficit-induced macronutrients, secondary metabolites and osmotic adjustments as the basis of crop production. The present investigation was undertaken to evaluate the physiological responses of the lavender (Lavandula officinalis L.), rosemary (Rosmarinus officinalis L.) and thyme (Thymus vulgaris) host plants to inoculation with mycorrhizal fungi (Funnelliformis mosseae, Rhizophagus irregularis compared with non-inoculated control treatment) in different irrigation levels (irrigation at 75% field capacity, 50% field capacity and rainfed). The 2-year (2015–2016) factorial experiment was conducted based on a randomized complete block design with three replications at Urmia University. The results showed that the stress treatments promoted the generation of osmotic adjustment (total soluble sugars and proline) in non-inoculated plants more than plants inoculated with fungi species. Nutrient (nitrogen, phosphorous and potassium) concentrations and leaf chlorophyll, reduced by water-limiting irrigation, were enhanced with mycorrhizal inoculation. Colonized plants were better adapted and had greater relative water content under stressful conditions due to higher absorption sites for water and nutrients. Also, stress treatments stimulated the phenylalanine ammonia-lyase, DPPH radical-scavenging activity and, the total phenolic and flavonoids content in non-inoculated plants more than that inoculated with two fungi species.
1. Introduction
Lavender (Lavandula officinalis L.), rosemary (Rosmarinus officinalis L.) and thyme (Thymus vulgaris L.) from Lamiaceae family are used to evaluate the existence of some typical secondary metabolites as phenolic acids and flavonoids. As the demand of lavender, rosemary and thyme increases, improving their cultivation under unfavorable environmental conditions such as drought will enhance their natural conservation and will sustain the pharmaceutical and cosmetic industries (Chrysargyris et al. Citation2016; Singh and Ramesh Citation2000; Tatrai et al. Citation2016). The highest essential oil percentage of lavender, rosemary and thyme were previously reported in rainfed condition compared with well-watered (irrigated at 75% FC (field capacity)) and moderate stress (irrigated at 50% FC). In plants irrigated at 75% FC, the yields of essential oil are reduced by mycorrhization because of the cost of carbohydrate. While, in stressed plants, mycorrhizal symbiosis can exhibit a benefit in terms of essential oil yield (Pirzad and Mohammadzadeh Citation2018).
Water scarcity, a structural condition in arid regions, affects aspects of the physiology of plants. The significant influence of deficit irrigation on the growth, yield, photosynthesis and chlorophyll of rosemary (Hassan, Bazaid, and Ali Citation2013), thyme (Bahreininejad, Razmjoo, and Mirza Citation2013) and lavender (Cordovilla et al. Citation2014) has been reported. With severe drought, irrigation arises as a general solution, but it may not be feasible or even desirable. Among physiological and biochemical adaptations in plants associated with water-stress tolerance, the mechanism of osmotic adjustment helps plants to maintain water uptake by accumulating large quantities of soluble carbohydrates and proline (Chen and Jiang Citation2010). In plants, secondary metabolites are not only involved in the treatment of human diseases (Wink Citation2015) but also another important part of plant defense system against environmental stresses (Yang et al. Citation2018). The limited water conditions decrease the uptake and translocation of water and nutrients that they were higher in mycorrhizal plants as a result of maintaining transpiration and photosynthesis longer during drought (Zhu et al. Citation2012).
Mycorrhizal fungi have positive effect on the quality of soil aggregation, structure of plant and bacteria communities, and environment stability (Diagne et al. Citation2020). Under water deficit conditions, arbuscular mycorrhizal fungal (AMF) symbiosis is known as bio enhancers of plants. These roots colonizers (AMF) influence the growth and productivity of plants (Habibzadeh et al. Citation2013; Begum et al. Citation2019), promote photosynthetic efficiency, enhance the antioxidant system and regulate the osmotic balance of plant (Baslam and Goicoechea Citation2012). Production of different solutes, extensive network of the mycorrhizal plant roots and enhanced nutrient uptake are all among the processes that enable the plant to improve their growth and productivity under stressed condition (Abdel Latef and Miransari Citation2014; Habeeb et al. Citation2020). The responses of plants to mycorrhizal symbiosis based on plant and fungi species may be different (Murphy et al. Citation2015; Zhang et al. Citation2013).
Current study, as field-based experiment is aimed at investigating the effects of two AMF species on the water deficit-induced by physiological responses of lavender, rosemary and thyme plants including nutrient elements, secondary metabolites and osmotic adjustment.
2. Materials and methods
For each plant species (L. officinalis cv. Silver, R. officinalis cv. Boule and T. vulgaris cv. Varico3), a 2-year (2015–2016) factorial experiment was separately conducted based on randomized complete block design with three replications at Agricultural Research Farm of Urmia University (37°33ʹ’ N, 45°05ʹ’ E, 1362 m a.s.l.).
2.1. Soil physic-chemical properties
The soil texture (clay {23% silt, 42% clay and 35% sand}- clay-Loam {29% silt, 40% clay and 31% sand}), organic matter (1.35–1.04%), total neutralizing value (17.15–17.42), electrical conductivity (0.56–0.51 dS m−1), pH (7.95–7.82), nitrogen (0.13–0.08%), available phosphorus (10.52–10.68 mg kg−1), available potassium (488–446 mg kg−1) were respectively determined for two separate depths 0–30 and 30–60 cm.
2.2. Experimental treatments
The three levels of irrigation including irrigation at 75% field capacity (I1), 50% field capacity (I2) and rainfed (I3) were applied after the last rainfall as the first factor. Mycorrhizal inoculation included Funnelliformis mosseae, Rhizophagus irregularis and a non-AMF inoculated treatment, were considered as the second factor.
2.3. Field preparation and plot experiment
The two-year age seedlings of lavender, rosemary and thyme (obtained from the Research Institute of Forests and Rangelands) were transplanted on the 22nd of April 2015. The size of plots were 500 × 250 cm that the three species of plants were transplanted in nine rows (50 cm inter and 45 cm intra row spaces). The mycorrhizal inocula, a mixture of sterile sand, mycorrhizal hyphae and spores (20 spores g−1 inoculum) and colonized root fragments were provided by Dr. Y. Rezaee Danesh (Department of Plant Protection, Urmia University). The inoculum was placed in the holes (20 g per hole) through the plant seedlings roots and lightly covered with soil on the day of planting (only in the first year). For non-AMF inoculated plants, seedlings were transplanted with no inoculation.
The percentage of plant root colonization was determined in 10plants per experimental unit. To this end, fresh roots were cleared in10% potassium hydroxide (KOH) for 10 min at 90°C, and then stainedin 0.05% lactic acid-glycerol-Trypan Blue (Phillips and Hayman Citation1970). Root colonization was assessed using the gridline intersect method of Liu and Luo (Citation1994). Mycorrhizal plants had higher colonization than control plants.
2.4. Irrigation water calculation
The irrigation water supply at each plot was measured with a water counter. According to Benami and Ofen (Citation1984), irrigation water needed prior to irrigation is the amount of water required during irrigation to replenish the soil moisture deficit, thereby taking the soil back to the field capacity. Based on the above method, the amounts of the used irrigation water were 2187, 1500 and 0 m3 ha−1 (for the first year), and 2625, 2250 and 0 m3 ha−1 (for the second year) for irrigation at 75% FC, 50% FC and rainfed condition (without irrigation), respectively. The lavender, rosemary and thyme plants were harvested on the 20 September.
2.5. Measurements
Nitrogen percentage was estimated using the semi-micro Kjeldahl method (Jackson, Miller, and Franklin Citation1973). To measure phosphorus of leaf, dried samples were milled, digested, and analyzed as described by Watanabe and Olsen (Citation1965), and Ohnishi, Gall, and Mayer (Citation1975). The flame photometric determination of potassium using ash extracts of plants has been studied based on Waling et al. (Citation1989).
The leaf relative water content (RWC) was determined by measuring the fresh weight (FW), rehydrated weight (SW) on distilled water, and dry weight (DW, 70°C for 48 h).
Total chlorophyll was estimated by extracting the leaves using 80% acetone as explained by Lichtenthaler and Buschmann (Citation2001). Leaf total soluble sugars and proline contents were measured by methods Dubois et al. (Citation1956), and Paquin and Lechasseur (Citation1979), respectively.
Total phenolic content (TPC) was determined with Folin–Ciocalteu reagent according to the method of Slinkard and Singleton (Citation1977) using gallic acid as a standard phenolic compound. The TPC was expressed as milligrams of gallic acid equivalents per gram fresh weight of sample (mg GAE g−1 FW). Absorbance was recorded at 760 nm using a U-2100 spectrophotometer.
Total flavonoid content (TFC) was tested according to Jia, Tan, and Wu (Citation1999) with minor modifications. The TFC was expressed as milligrams of quercetin equivalents per gram fresh weight of sample (mg QCE g−1 FW). The absorbance was measured at 510 nm.
Phenylalanine ammonia-lyase (PAL) activity was measured according to the method of D’Cunha, Satyanarayan, and Nair (Citation1996). The absorbance of reaction mixture was measured at 290 nm using an extinction coefficient of 9630 mM−1 cm−1. One unit of PAL activity is equal to 1 µmol of cinnamic acid produced per g fresh weight min−1.
The measurement of 2, 2-diphenyl-1-picrylhydrazyl radical scavenging activity (DPPH) was carried out according to the method of Hatano et al. (Citation1988). The reduction of DPPH radicals was determined by measuring the absorption at 517 nm. The radical scavenging activity was calculated as a percentage of DPPH discoloration using the following equation:
DPPH radical scavenging % = [(A0 – A1)/A0] × 100
Where A0 is the absorbance of the DPPH solution and A1 is the absorbance of the sample.
2.6. Statistical analyses
Analysis of variance for the two years data was performed using SAS 9.1 software as combined over the years. The means were compared by Duncan’s Multiple Range Test (DMRT) test (P ≤ 0.05).
3. Results
3.1. Lavandula officinalis
In irrigated lavender, mycorrhization had no significant effect on Nleaf. In non-AMF rainfed plants, leaf nitrogen decreased to the lowest possible, but it was even increased up to amounts more than that of irrigated plants by colonization (). In plants of I1, Pleaf was not affected by mycorrhizal inoculation. Leaf phosphorus was improved by ‘F. mosseae’ symbiosis in I2, and by ‘R. irregularis’ in I3 treatments (). Leaf potassium of AMF-inoculated plants was not affected by drought, so they were the identically highest Kleaf of mycorrhized lavenders in I2 and I3 treatments. Though, Kleaf of non-AMF inoculated plants was respectively decreased by 22 and 25% in irrigation at 50% FC and rainfed conditions ().
Table 1. Means comparison of nutrient element and osmolytes plants affected by ‘irrigation×mycorrhiza’
Increasing irrigation intervals led to significant reduction in total chlorophyll of non-inoculated plants; led to minimum concentration in rainfed plants. Colonization by ‘F. mosseae’ showed higher leaf chlorophyll in I1 and I3 treatments, whereas it was greater in ‘R. irregularis’ symbiosis in I2 treatment ().
Plants irrigated at 75% FC exhibited low proline, while stressed plants (50% FC and rainfed) showed the same increase of leaf proline ()). Mycorrhizal symbiosis caused lower concentrations of leaf proline in rainfed plants, which is identical to plant irrigated at 50% FC ()).
Figure 1. Means comparison of leaf proline in different levels of irrigation (a), and different species of mycorrhizae (b), and the means comparison of and 2, 2-diphenyl-1-picrylhydrazyl (c) in different levels of irrigation for lavender plants, and means comparison of proline (d) and total flavonoid content (e) in different levels of irrigation for thyme plants. The same letters show non-significant differences at P ≤ 0.05
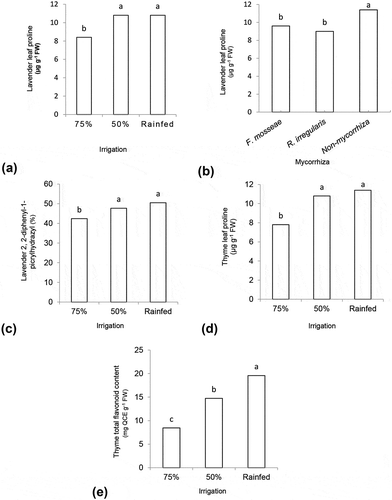
In non-AMF inoculated control plants, leaf RWC declined with intensification of stress level. In plants irrigated at 50% FC, AMF-inoculation improved leaf water content, so that the highest values belonged to irrigation at 50% FC. Plants were affected only by mycorrhization under stress conditions, positively ().
Table 2. Means comparison of some physiological traits of plants affected by ‘irrigation×mycorrhiza’
In non-AMF inoculated rainfed lavender plants, total flavonoid content markedly increased up to 34% compared to irrigated plants (50 and 75% FC). Despite the decrease in rainfed plants, mycorrhization did not affect the total flavonoid content of irrigated plants ().
The PAL activity of lavender plants increased in response to stress. Though, the PAL enzyme had a lower activity in AMF inoculated rainfed plants ().
A gradual increase of total phenolic content was observed in non-inoculated control plants expose to drought, but it was not increase in AMF-inoculated plants. The total phenolic content decreased by ‘F. mosseae’ in plants irrigated at 50% FC. TPC of rainfed lavender plants were identically diminished by application of two species of fungi ().
With increasing the severity of water stress, in rainfed condition, DPPH (50.50%) continued to be accumulated in the highest amounts ()).
3.2. Rosmarinus officinalis
In irrigated plants inoculation with ‘F. mosseae’ had only positive effect on leaf nitrogen, which was much more in plants irrigated at 75% FC. Though in rainfed plants, AMF inoculation showed a little enhancement in terms of Nleaf (). In all irrigation treatments, non-inoculated control plants exhibited the same lowest concentration of Pleaf. The excellence of Pleaf of plants inoculated with ‘F. mosseae’ at 50% FC was evident. There was no improvement of Pleaf in plants I1 and I3 by AMF inoculation. Though, higher Pleaf was observed in irrigated plants (). Stress reduced Kleaf, leading to the least concentration in rainfed plants. In spite of the superiority of water supply of I1, I2 and I3, respectively, colonization in the three irrigation regimes improved the Kleaf ().
A gradual decrease of total chlorophyll was observed in control plants exposed to drought. AMF-inoculation was enhanced by 2–4 fold in irrigated plants. This effect was more notable in the plants inoculated with ‘R. irregularis’ ().
Rosemary plants significantly demonstrated higher levels of leaf TSS in stress conditions, regardless of AMF inoculation. In the application of mycorrhiza, TSS was the same for plants irrigated at 75% FC and rainfed, and it was even higher than non-AMF inoculated plants for irrigation at 50% FC ().
With increase in the severity of water stress in rainfed condition, the leaf proline continued to be accumulated in the highest amounts, and mycorrhization diminished the leaf proline accumulation. Reduction of proline, which occurred by water supply in irrigated plants ().
In non-AMF inoculated plants, the relative water content was the highest identically in irrigated rosemary, but rainfed condition caused significant decrease of RWC. Mycorrhizal inoculations exhibited no benefit on leaf RWC for irrigated plants. Inoculation with ‘F. mosseae’ and ‘R. irregularis’ enhanced water status (RWC) in rainfed condition ().
In non-inoculated control plants, the total flavonoid content increased in water deficit condition either in I2 and I3 treatments, while inoculated plants reduced total flavonoid content in stress condition. Inoculation of drought-exposed rosemary plants with both fungi was confirmed to decrease tissue TFC accumulation ().
In non-AMF control plants, the significant increase of PAL was occurred by water shortage reached to the maximum in rainfed plants. In I3, the PAL activity in leaves of rosemary were positively influenced by two fungi species, while in I2 only plants inoculated with F. mosseae presented lower activity of PAL than that ones in the control untreated plants ().
In non-AMF inoculated plants, the total phenolic content was the highest in rainfed rosemary that decreased by mycorrhization with two species ().
There was a considerable reduction in the DPPH radical-scavenging activity of control plants in the order: rainfed >50% FC >75% FC. The application of mycorrhiza improved DPPH in stress condition, with the same advantage of two fungi species ().
3.3. Thymus vulgaris
In non-AMF inoculated control plants, leaf nitrogen did not change in irrigated and rainfed conditions. In AMF-inoculated plants, increasing trends of Nleaf were observed by irrigation water supply from I3 to I1 treatments. Fungus of ‘F. mosseae’ was more efficient on the leaf nitrogen. On the contrary, symbiosis with ‘F. mosseae’ obtained the highest values (up to 36 mg g−1) of Nleaf for all irrigation levels (). Leaf phosphorous had a notable decrease in non-AMF inoculated rainfed plants; and Pleaf was much higher in mycorrhizal plants (). The fungi increased Kleaf only in unstressed condition, with the same advantage ().
The leaf chlorophyll concentration of non-AMF control plants was decreased in response to water stress. Furthermore, mycorrhizal plants produced higher total chlorophyll in stressed plants ().
A gradual increase was observed in TSS concentration with water deficit in all colonized and/or control plants. This trend was steeper in the control plants ().
However, the leaf proline showed the highest value for rainfed plants, as much as irrigation at 50% FC ()).
With increasing stress, leaf RWC decrease in untreated control plants. The non-AMF thyme plants in I1 maintained the leaf RWC higher than 60%. In stressed (irrigation at 50% FC and rainfed) plants, mycorrhizal inoculation enhanced the RWC as well as plants irrigated at 75% FC ().
Deficit irrigation (I2) did not have significant effect on PAL activity, but it was markedly increased with extensive irrigation intervals. The effect of fungi in decreasing of PAL activity was only observed in the I3 with no superiority of mycorrhizal fungi inoculation ().
The total phenol content of non-inoculated thyme plants increased in exposure to stress, getting a maximum value in rainfed condition, which was 14.69% more than of the AMF-inoculated thyme plants ().
Exposure of plants to severe stress (I3) caused to increase the total flavonoid content (from 8.45 to 19.59 mg QCE g−1 FW), more than 2-fold of plants irrigated at 75% FC ()).
Control plants irrigated at 75% FC contained lower DPPH radical-scavenging activity than those with limited irrigation. AMF colonization reduced DPPH in leaves of rainfed plants ().
4. Discussion
Our findings show that stress reduced the rate of nutrient (NPKleaf) uptake in control plants, which was more noticeable in rainfed condition, even though mycorrhizal fungi could mitigate this reduction. New evidence provided new idea into the exchange of nutritional benefits between the symbiotic associates (Chen et al. Citation2018). The nutrition translocation to the leaf was increased much more in the mycorrhizal plants than in non-mycorrhizal. Nutritional unbalances caused by drought were previously reported on lavender (Armada, Roldan, and Azcon Citation2014). In drought soils, plants are more dependent on mycorrhizal activity which is able to increase nutrients and water uptake. The seedlings (lavender, rosemary and thyme) inoculated with fungi and subjected to water stress had more successful colonization (Pirzad and Mohammadzadeh Citation2018). Plant root colonization by AMF can range from highly positive to highly negative. Mycorrhizal associations could consider symbioses that functionally range along a continuum of parasitism to mutualism (Johnson, Graham, and Smith Citation1997), and that plant species, even from the same genus and/or family, determine the position of AMF (Matysiak and Falkowski Citation2010; Moora et al. Citation2004). In support of current investigation of our data, recent studies have shown that abscisic acid levels increased in response to water deficit more in control plants than in inoculated plants, suggesting that AMF plants experience less intense drought (Doubkova, Vlasakova, and Sudova Citation2013). In tomato mutant sitiens in which ABA levels are reduced, they were able to show that ABA is necessary for the proper formation of arbuscules and for a sustained colonization of the plant root. The plants with reduced AM formation can be restored by the application of ABA, correlating with increased mycorrhization by exogenous ABA (Herrera Medina et al. Citation2007). AMF regulation of plant aquaporin genes under drought generally improves plant water status and drought tolerance (Li et al. Citation2013). Our results showed that application of mycorrhizal fungi improved nitrogen, phosphorous and potassium uptake in three Lamiaceae plants. This effect varied depending on the plant and fungi species. So, the highest colonization was obtained from rosemary plants followed by thyme and lavender plants (Pirzad and Mohammadzadeh Citation2018). There is abundant evidence that AMF can acquire nitrogen (presumably also P and K) from decomposing organic and inorganic material and transfer it to the plant (Govindarajulu et al. Citation2005; Perez-Tienda et al. Citation2012). Higher nutrient uptake in mycorrhizal plants might be attributed to the wider absorption surface provided by the hyphae (smaller diameter than root) in the soil and thus absorb more nutrients (Smith et al. Citation2010, Citation2011; Rahimzadeh and Pirzad Citation2017a, Citation2017b). Another explanation for the improved nutrient status in mycorrhizal plants is utilization of more soluble phosphate from rock phosphate than non-inoculated plants (Gyaneshwar et al. Citation2002). In addition to P and N, other nutrients are also transferred from the fungus to the host (Cruz et al. Citation2007; Mensah et al. Citation2015). The maintenance of the AMF symbiosis is more determined by the sum of nutritional benefits that are provided through the mycorrhizal interface (Carbonnel and Gutjahr Citation2014). However, the symbiosis also provides a variety of non-nutritional benefits to the host plants. For example, the carbon supply of the host acts as an important trigger for P and N transport in the AMF symbiosis (Fellbaum et al. Citation2014). Interestingly, a strong correlation between potassium and phosphorous during AMF symbiosis was reported. Olsson et al. (Citation2011) highlighted a co-distribution and a linked ratio of potassium and phosphorous in ‘R. irregularis’ spores, hyphae and vesicles. When spores were enriched in P, an increase of K content was observed. From current study, it can be concluded that the use of AMF could reduce the amount of fertilizer needed to produce sustainable crops.
Chlorophyll content was reduced by severe water deficit stress (rainfed condition) as compared to plants irrigated at 75% FC. The differences of photosynthetic pigments were significant between AMF and non-AMF plants under drought conditions. Rahimzadeh and Pirzad (Citation2017a; Citation2017b) reported high total chlorophyll from mycorrhizal plants as well. The concentrations of total chlorophyll in mycorrhizal fungi inoculated plants were higher in order of lavender, thyme and rosemary under stress conditions.
Progressive water deficit stress increased the concentration of proline and soluble sugars in untreated control rosemary plants followed by lavender and thyme. Leaf proline in all plants was diminished by AMF-inoculation, but total soluble sugar in lavender and rosemary was not affected by AMF-inoculation, positively. Mycorrhizal fungi significantly decrease the harmful effect of drought stress by up-regulating the antioxidant defense system, synthesis of osmolytes, and maintaining phytohormone levels (Al-Arjani, Hashem, and Abd Allah Citation2020). The accumulation of the osmolytes in the cells as a result of water stress is often associated with a possible mechanism to tolerate the harmful effect of water shortage (Pirzad et al. Citation2011), which can help the plants to maintain cell turgor and structural integrity of membranes (Hayat et al. Citation2012). Proline accumulation is responsible for the hydration of biopolymers surviving as a readily utilizable energy source and serving as a nitrogen source compound during periods of inhibited growth (Sharma and Singh Citation2016), and is used as a biochemical marker of drought level in plants. In fact, proline as an effective scavenger of ROS, could also be considered as a marker of the potential injury caused by water deficit. Mycorrhizal plants characterized by lower proline accumulation show less stress than the non-mycorrhizal plants (Kishor et al. Citation2005).
In our study, leaf relative water content as an index of water status was decreased under water stress conditions, while it increased in plants inoculated with mycorrhizal fungi. This maximum increase for lavender and rosemary were in symbiosis with ‘F. mosseae’, while it had been observed in ‘R. irregularis’ inoculated thyme. Several studies have reported that the enhancement of leaf water potential, relative water content and stomatal conductance resulting in efficiency of CO2 assimilation in the host plants are often related to the effect of AMF symbiosis (Barzana et al. Citation2012; Ruiz-Sanchez et al. Citation2011).
Phenylalanine ammonia lyase leads to the biosynthesis of a wide array of secondary metabolites (Popovic et al. Citation2016). Plant phenols and flavonoids, as secondary natural metabolites, are considered to have a key role as defense compounds against higher production of free radicals and other oxidative species in plants (Ramawat and Merillon Citation2013). Significant positive correlations were obtained between phenylalanine ammonia lyase and antioxidant capacity as well as phenolic acids among themselves (Popovic et al. Citation2016). Increase the severity of water stress in rainfed condition, the phenol, flavonoid and PAL of plants (lavender, rosemary and thyme) continued to be accumulated in the highest amounts, and mycorrhization by fungi species identically diminished their accumulation. The association of AMF inoculation, to reduce the harmful effects of stress and thus reduce the production of secondary metabolites, was the most successful in rainfed condition.
5. Conclusion
Generally, Inoculation with two species of mycorrhizal fungi was able to compensate stress-induced reduction of nitrogen, phosphorus and potassium (NPKleaf). Drought also decreased total chlorophyll in non-inoculated plants. Inoculation with fungi improved chlorophyll content in stressed plants, identically by two species of fungi. Though, mycorrhization showed the higher leaf chlorophyll by ‘F. mosseae’ in thyme and lavender plants, and by ‘R. irregularis’ in rosemary. Increasing irrigation intervals reduced the relative water content in non-inoculated plants but it was improved in AMF-inoculated rainfed plants much more than irrigated plants. A gradual increase was observed in osmolytes concentrations with increase in severity of water stress in all three plants species. This trend was more severe in non-AMF inoculated control plants than mycorrhizal plants. Stress treatments stimulated the PAL, DPPH radical-scavenging activity and, phenolic and flavonoids content in non-inoculated. Inoculation with fungi significantly reduced their concentration in stressed plants.
Acknowledgments
We thank Professor Mohammad Moghaddam from University of Tabriz for contribution to statistical analysis.
Disclosure statement
The authors confirm that there are no known conflicts of interest associated with this publication and there has been no significant financial support for this work that could have influenced its outcome.
References
- Abdel Latef, A. A. H., and M. Miransari. 2014. Use of Microbes for the Alleviation of Soil Stresses. New York, USA: Springer.
- Al-Arjani, A. F., A. Hashem, and E. F. Abd Allah. 2020. “Arbuscular Mycorrhizal Fungi Modulates Dynamics Tolerance Expression to Mitigate Drought Stress in Ephedra Foliata Boiss.” Saudi Journal of Biological Sciences 27 (1): 380–394. doi:10.1016/j.sjbs.2019.10.008.
- Armada, E., A. Roldan, and R. Azcon. 2014. “Differential Activity of Autochthonous Bacteria in Controlling Drought Stress in Native Lavandula and Salvia Plants Species under Drought Conditions in Natural Arid Soil.” Microbial Ecology 67 (2): 410–420. doi:10.1007/s00248-013-0326-9.
- Bahreininejad, B., J. Razmjoo, and M. Mirza. 2013. “Influence of Water Stress on Morpho-physiological and Phytochemical Traits in Thymus Daenensis.” International Journal of Plant Production 7: 151–166. doi:10.22069/IJPP.2012.927.
- Barzana, G., R. Aroca, J. A. Paz, F. Chaumont, M. C. Martinez-Ballesta, M. Carvajal, and J. M. Ruiz-Lozano. 2012. “Arbuscular Mycorrhizal Symbiosis Increases Relative Apoplastic Water Flow in Roots of the Host Plant under Both Well-watered and Drought Stress Conditions.” Annals of Botany 109 (5): 1009–1017. doi:10.1093/aob/mcs007.
- Baslam, M., and N. Goicoechea. 2012. “Water Deficit Improved the Capacity of Arbuscular Mycorrhizal Fungi (AMF) for Inducing the Accumulation of Antioxidant Compounds in Lettuce Leaves.” Mycorrhiza 22 (5): 347–359. doi:10.1007/s00572-011-0408-9.
- Begum, N., C. Qin, M. A. Ahanger, S. Raza, M. I. Khan, M. Ashraf, N. Ahmed, and L. Zhang. 2019. “Role of Arbuscular Mycorrhizal Fungi in Plant Growth Regulation: Implications in Abiotic Stress Tolerance.” Frontiers in Plant Science 10: 1068. doi:10.3389/fpls.2019.01068.
- Benami, A., and A. Ofen. 1984. Irrigation Engineering-sprinkler, Trickle and Surface Irrigation: Principles, Design and Agricultural Practices. Bet Dagan: Irrigation Engineering Scientific Publications.
- Carbonnel, S., and C. Gutjahr. 2014. “Control of Arbuscular Mycorrhiza Development by Nutrient Signals.” Frontiers in Plant Science 5: 462. doi:10.3389/fpls.2014.00462.
- Chen, H., and J. G. Jiang. 2010. “Osmotic Adjustment and Plant Adaptation to Environmental Changes Related to Drought and Salinity.” Environmental Reviews 18: 309–319. doi:10.1139/A10-014.
- Chen, M., M. Arato, L. Borghi, E. Nouri, and D. Reinhardt. 2018. “Beneficial Services of Arbuscular Mycorrhizal Fungi - from Ecology to Application.” Frontiers in Plant Science 9: 1270. doi:10.3389/fpls.2018.01270.
- Chrysargyris, A., S. Laoutari, V. D. Litskas, M. C. Stavrinides, and N. Tzortzakis. 2016. “Effects of Water Stress on Lavender and Sage Biomass Production, Essential Oil Composition and Biocidal Properties against Tetranychus Urticae.” Scientia Horticulturae 213: 96–103. doi:10.1016/j.scienta.2016.10.024.
- Cordovilla, M. P., M. Bueno, C. Aparicio, and M. Urrestarazu. 2014. “Effects of Salinity and the Interaction between Thymus Vulgaris and Lavandula Angustifolia on Growth, Ethylene Production and Essential Oil Contents.” Journal of Plant Nutrition 37: 875–888. doi:10.1080/01904167.2013.873462.
- Cruz, C., H. Egsgaard, C. Trujillo, P. Ambus, N. Requena, M. A. Martins-Loucao, and I. Jakobsen. 2007. “Enzymatic Evidence for the Key Role of Arginine in Nitrogen Translocation by Arbuscular Mycorrhizal Fungi.” Plant Physiology 144 (2): 782–792. doi:10.1104/pp.106.090522.
- D’Cunha, G. B., V. Satyanarayan, and P. M. Nair. 1996. “Purification of Phenylalanine ammonia Lyase from Rhodotorula Glutinis.” Phytochemistry 42 (1): 17–20. doi:10.1016/0031-9422(95)00914-0.
- Diagne, N., M. Ngom, P. I. Djighaly, D. Fall, V. Hocher, and S. Svistoonoff. 2020. “Roles of Arbuscular Mycorrhizal Fungi on Plant Growth and Performance: Importance in Biotic and Abiotic Stressed Regulation.” Diversity 12 (10): 370. doi:10.3390/d12100370.
- Doubkova, P., E. Vlasakova, and R. Sudova. 2013. “Arbuscular Mycorrhizal Symbiosis Alleviates Drought Stress Imposed on Knautia Arvensis Plants in Serpentine Soil.” Plant and Soil 370 (1–2): 149–161. doi:10.1007/s11104-013-1610-7.
- Dubois, M., K. A. Gilles, J. K. Hamilton, P. A. Rebers, and F. Smith. 1956. “Colorimetric Method for Determination of Sugars and Related Substances.” Journal of Analytical Chemistry 28 (3): 350–356. doi:10.1021/ac60111a017.
- Fellbaum, C. R., J. A. Mensah, A. J. Cloos, G. E. Strahan, P. E. Pfeffer, E. T. Kiers, and H. Bucking. 2014. “Fungal Nutrient Allocation in Common Mycorrhizal Networks Is Regulated by the Carbon Source Strength of Individual Host Plants.” New Phytologist 203 (2): 646–656. doi:10.1111/nph.12827.
- Govindarajulu, M., P. E. Pfeffer, H. Jin, J. Abubaker, D. D. Douds, J. W. Allen, H. Bucking, P. J. Lammers, and Y. Shachar-Hill. 2005. “Nitrogen Transfer in the Arbuscular Mycorrhizal Symbiosis.” Nature 435 (7043): 819–823. doi:10.1038/nature03610.
- Gyaneshwar, P., G. Kumar, L. Parekh, and P. Poole. 2002. “Role of Soil Microorganisms in Improving P Nutrition of Plants.” Plant and Soil 245 (1): 133–143. doi:10.1023/A:1020663916259.
- Habeeb, T. H., M. Abdel-Mawgoud, R. S. Yehia, A. M. A. Khalil, A. M. Saleh, and H. Abd Elgawad. 2020. “Interactive Impact of Arbuscular Mycorrhizal Fungi and Elevated CO2 on Growth and Functional Food Value of Thymus Vulgare.” Thymus Vulgare. Journal of Fungi 6 (3): 168. doi:10.3390/jof6030168.
- Habibzadeh, Y., A. Pirzad, M. R. Zardashti, J. Jalilian, and O. Eini. 2013. “Effects of Arbuscular Mycorrhizal Fungi on Seed and Protein Yield under Water-deficit Stress in Mung Bean.” Agronomy Journal 105 (1): 79–84. doi:10.2134/agronj2012.0069.
- Hassan, F. A. S., S. Bazaid, and E. F. Ali. 2013. “Effect of Deficit Irrigation on Growth, Yield and Volatile Oil Content on Rosmarinus Officinalis L. Plant.” Journal of Medicinal Plants Studies 1: 12–21.
- Hatano, T., H. Kagawa, T. Yasuhara, and T. Okuda. 1988. “Two New Flavonoids and Other Constituents in Licorice Root: Their Relative Astringency and Radical Scavenging Effects.” Chemical and Pharmaceutical Bulletin 36 (6): 2090–2097. doi:10.1248/cpb.36.2090.
- Hayat, S., Q. Hayat, M. N. Alyemeni, A. S. Wani, J. Pichtel, and A. Ahmad. 2012. “Role of Proline under Changing Environments.” Plant Signaling and Behavior 7 (11): 1456–1466. doi:10.4161/psb.21949.
- Herrera Medina, M. J., S. S. Teinkellner, H. Vierheilig, J. A. Ocampo Bote, and J. M. García Garrido. 2007. “Abscisic Acid Determines Arbuscule Development and Functionality in the Tomato Arbuscular Mycorrhiza.” New Phytologist 175 (3): 554–564. doi:10.1111/j.1469-8137.2007.02107.x.
- Jackson, N. E., R. H. Miller, and R. E. Franklin. 1973. “The Influence of Vesicular-arbuscular Mycorrhizae on Uptake of 90Sr from Soil by Soybeans.” Soil Biology and Biochemistry 5 (2): 205–212. doi:10.1016/0038-0717(73)90003-5.
- Jia, Z., M. Tan, and J. Wu. 1999. “The Determination of Flavonoid Contents in Mulberry and Their Scavenging Effects on Superoxide Radicals.” Food Chemistry 64 (4): 555–559. doi:10.1016/S0308-8146(98)00102-2.
- Johnson, N. C., J. H. Graham, and F. A. Smith. 1997. “Functioning of Mycorrhizal Associations along the Mutualism Parasitism Continuum.” New Phytologist 135 (4): 575–586. doi:10.1046/j.1469-8137.1997.00729.x.
- Kishor, P. K. B., S. S. Angam, R. N. Amrutha, P. S. Laxmi, K. R. Naidu, K. R. S. S. Rao, R. Sreenath, K. J. Reddy, P. Theriappan, and N. Sreenivasulu. 2005. “Regulation of Proline Biosynthesis, Degradation, Uptake and Transport in Higher Plants: Its Implications in Plant Growth and Abiotic Stress Tolerance.” Current Science 88: 424–438.
- Li, T., Y. J. Hu, Z. P. Hao, H. Li, Y. S. Wang, and B. D. Chen. 2013. “First Cloning and Characterization of Two Functional Aquaporin Genes from an Arbuscular Mycorrhizal Fungus Glomus Intraradices.” New Phytologist 197 (2): 617–630. doi:10.1111/nph.12011.
- Lichtenthaler, H. K., and C. Buschmann. 2001. “Chlorophylls and Carotenoids: Measurement and Characterization by UV-VIS Spectroscopy.” In Current Protocols in Food Analytical Chemistry, edited by R. E. Wrolstad, T. E. Acree, H. An, E. A. Decker, M. H. Penner, D. S. Reid, S. J. Schwartz, C. F. Shoemaker, and P. Sporns, F4.3.1–F4.3.8. New York: Current Protocols.
- Liu, R. J., and X. S. Luo. 1994. “A New Method to Quantify the Inoculum Potential of Arbuscular Mycorrhizal Fungi.” New Phytologist 128 (1): 89–92. doi:10.1111/j.1469-8137.1994.tb03990.x.
- Matysiak, B., and G. Falkowski. 2010. “Response of Three Ornamental Plant Species to Inoculation with Arbuscular Mycorrhizal Fungi Depending on Compost Addition to Peat Substrate and the Rate of Controlled Release Fertilizer.” Journal of Fruit and Ornamental Plant Research 18: 321–333.
- Mensah, J. A., A. M. Koch, P. M. Antunes, E. T. Kiers, M. Hart, and H. Bucking. 2015. “High Functional Diversity within Species of Arbuscular Mycorrhizal Fungal Is Associated with Differences in Phosphate and Nitrogen Uptake and Fungal Phosphate Metabolism.” Mycorrhiza 25 (7): 533–546. doi:10.1007/s00572-015-0631-x.
- Moora, M., M. Opik, R. Sen, and M. Zobel. 2004. “Native Arbuscular Mycorrhizal Fungal Communities Differentially Influence the Seedling Performance of Rare and Common Pulsatilla Species.” Functional Ecology 18 (4): 554–562. doi:10.1111/j.0269-8463.2004.00876.x.
- Murphy, B. R., L. Martin-Nieto, F. Doohan, and T. Hodkinson. 2015. “Fungal Endophytes Enhance Agronomically Important Traits in Severely Drought‐stressed Barley.” Journal of Agronomy and Crop Science 201 (6): 419–427. doi:10.1111/jac.12139.
- Ohnishi, T., R. S. Gall, and M. L. Mayer. 1975. “An Improved Assay of Inorganic Phosphate in the Presence of Extralabile Phosphate Compounds: Application to the ATPase Assay in the Presence of Phosphocreatine.” Analytical Biochemistry 69 (1): 261–267. doi:10.1016/0003-2697(75)90585-0.
- Olsson, P. A., E. C. Hammer, J. Pallon, I. M. van Aarle, and H. Wallander. 2011. “Elemental Composition in Vesicles of an Arbuscular Mycorrhizal Fungus, as Revealed by PIXE Analysis.” Fungal Biology 115 (7): 643–648. doi:10.1016/j.funbio.2011.03.008.
- Paquin, R., and P. Lechasseur. 1979. “Observations sur une methode de dosage de la praline libre dans les extraits de plantes.” Canadian Journal of Botany 57 (18): 1851–1854. doi:10.1139/b79-233.
- Perez-Tienda, J., A. Valderas, G. Camanes, P. Garcia-Agustin, and N. Ferrol. 2012. “Kinetics of NH4+ Uptake by the Arbuscular Mycorrhizal Fungus Rhizophagus Irregularis.” Mycorrhiza 22 (6): 485–491. doi:10.1007/s00572-012-0452-0.
- Phillips, J. M., and D. S. Hayman. 1970. “Improved Procedures for Clearing Roots and Staining Parasitic and Vesicular Arbuscular Mycorrhizal Fungi for Rapid Assessment of Infection.” Transactions of the British Mycological Society 55 (1): 158–161. doi:10.1016/S0007-1536(70)80110-3.
- Pirzad, A., and S. Mohammadzadeh. 2018. “Water Use Efficiency of Three Mycorrhizal Lamiaceae Species (Lavandula Officinalis, Rosmarinus Officinalis and Thymus Vulgaris).” Agricultural Water Management 204: 1–10. doi:10.1016/j.agwat.2018.03.020.
- Pirzad, A., M. R. Shakiba, S. Zehtab-Salmasi, S. A. Mohammadi, R. Darvishzadeh, and A. Samadi. 2011. “Effect of Water Stress on Leaf Relative Water Content, Chlorophyll, Proline and Soluble Carbohydrates in Matricaria Chamomilla L.” Journal of Medicinal Plants Research 5: 2483–2488.
- Popovic, B. M., D. Stajner, R. Zdero-Pavlovic, V. Tumbas-Saponjac, J. Canadanovic-Brunet, and S. Orlovic. 2016. “Water Stress Induces Changes in Polyphenol Profile and Antioxidant Capacity in Poplar Plants (Populus Spp.).” Plant Physiology and Biochemistry 105: 242–250. doi:10.1016/j.plaphy.2016.04.036.
- Rahimzadeh, S., and A. Pirzad. 2017a. “Arbuscular Mycorrhizal Fungi and Pseudomonas in Reduce Drought Stress Damage in Flax (Linum Usitatissimum L.): A Field Study.” Mycorrhiza 27 (6): 537–552. doi:10.1007/s00572-017-0775-y.
- Rahimzadeh, S., and A. Pirzad. 2017b. “Microorganisms (AMF and PSB) Interaction on Linseed Productivity under Water-deficit Condition.” International Journal of Plant Production 11: 259–274. doi:10.22069/ijpp.2017.3423.
- Ramawat, K. G., and J. M. Merillon. 2013. Natural Products: Phytochemistry, Botany and Metabolism of Alkaloids, Phenolics and Terpenes. Berlin Heidelberg: Springer.
- Ruiz-Sanchez, M., E. Armada, Y. Munoz, I. E. Garcia de Salamone, R. Aroca, J. M. Ruiz-Lozano, and R. Azcon. 2011. “Azospirillum and Arbuscular Mycorrhizal Colonization enhance Rice Growth and Physiological Traits under Well-watered and Drought Conditions.” Journal of Plant Physiology 168 (10): 1031–1037. doi:10.1016/j.jplph.2010.12.019.
- Sharma, A. K., and S. P. Singh. 2016. “Effect of Amino Acids on the Repression of Alkaline Protease Synthesis in Haloalkaliphilic Nocardiopsis Dassonvillei.” Biotechnology Reports 12: 40–51. doi:10.1016/j.btre.2016.10.004.
- Singh, M., and S. Ramesh. 2000. “Effect of Irrigation and Nitrogen on Herbage, Oil Yield and Water-use Efficiency in Rosemary Grown under Semi-arid Tropical Conditions.” Journal of Medicinal and Aromatic Plant Sciences 22: 659–662.
- Slinkard, K., and V. L. Singleton. 1977. “Total Phenol Analysis Automation and Comparison with Manual Methods.” American Journal of Enology and Viticulture 28: 49–55.
- Smith, S. E., E. Facelli, S. Pope, and F. A. Smith. 2010. “Plant Performance in Stressful Environments: Interpreting New and Established Knowledge of the Roles of Arbuscular Mycorrhizas.” Plant and Soil 326 (1–2): 3–20. doi:10.1007/s11104-009-9981-5.
- Smith, S. E., I. Jakobsen, M. Grønlund, and F. Smith. 2011. “Roles of Arbuscular Mycorrhizas in Plant Phosphorous Nutrition: Interactions between Pathways of Phosphorous Uptake in Arbuscular Mycorrhizal Roots Have Important Implications for Understating and Manipulating Plant Phosphorous Acquisition.” Plant Physiology 156 (3): 1050–1057. doi:10.1104/pp.111.174581.
- Tatrai, Z. A., R. Sanoubar, Z. Pluhar, S. Mancarella, F. Orsini, and G. Gianquinto. 2016. “Morphological and Physiological Plant Responses to Drought Stress in Thymus Citriodorus.” International Journal of Agronomy 2016: 1–8. Article ID 4165750. doi:10.1155/2016/4165750.
- Waling, I., W. V. Vark, V. J. G. Houba, and J. J. Van der Lee. 1989. Soil and Plant Analysis, a Series of Syllabi, Part 7, Plant Analysis Procedures. Wageningen, The Netherlands: Wageningen Agricultural University.
- Watanabe, F. S., and S. R. Olsen. 1965. “Test of an Ascorbic Acid Method for Determining Phosphorus in Water and NaHCO3 Extracts from Soil.” Soil Science Society of America, Proceedings 29 (6): 677–678. doi:10.2136/sssaj1965.03615995002900060025x.
- Wink, M. 2015. “Modes of Action of Herbal Medicines and Plant Secondary Metabolites.” Medicines 2 (3): 251–286. doi:10.3390/medicines2030251.
- Yang, L., K. S. Wen, X. Ruan, Y. X. Zhao, F. Wei, and Q. Wang. 2018. “Response of Plant Secondary Metabolites to Environmental Factors.” Molecules 23: 762. doi:10.3390/molecules23040762.
- Zhang, B. B., W. Z. Liu, S. X. Chang, and A. O. Anyia. 2013. “Phosphorus Fertilization and Fungal Inoculations Affected the Physiology, Phosphorus Uptake and Growth of Spring Wheat under Rainfed Conditions on the Canadian Prairies.” Journal of Agronomy and Crop Science 199 (2): 85–93. doi:10.1111/jac.12001.
- Zhu, X. C., F. B. Song, S. Q. Liu, T. D. Liu, and X. K. Zhou. 2012. “Arbuscular Mycorrhizae improves Photosynthesis and Water Status of Zea Mays L. Under Drought Stress.” Plant, Soil and Environment 58 (No. 4): 186–191. doi:10.17221/23/2011-PSE.