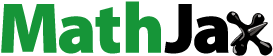
ABSTRACT
This study aimed to explore the effect of Chinese milk vetch (CMV) on soil fertility and rice production in double rice cropping systems. A field experiment was conducted including the following six treatments: Ck (no NPK mineral fertilizer and no CMV), CMV0 (NPK mineral fertilizer and no CMV), and CMV1–4 (NPK mineral fertilizer and 7500 kg ha−1 CMV, 15,000 kg ha−1 CMV, 22,500 kg ha−1 CMV, 30,000 kg ha−1 CMV) from 2008 to 2018. The results showed that the application of CMV enhanced the organic matter, the total nitrogen, the available phosphorus, pH, and enzyme activities (except for the cellulase) in paddy soils. The organic matter and total nitrogen of CMV3 were increased by 15.99% and 8.07% compared with CMV0 and the increase rate of the organic matter and total nitrogen was by an average of 0.413 and 0.029 g kg−1 per year compared with the initial soil, respectively. The CMV return also improved the enzyme activities (except for the cellulase). Meanwhile, the CMV returned to soil increased dramatically the yields of early rice, late rice, and double rice by 13.46%-26.23%, 13.63%-20.84%, and 13.55%-25.76% compared with CMV0, respectively. The yields of early rice, late rice, and double rice of the CMV3 were highest among all the treatments. The application of CMV improved the agronomic efficiency (AE) and the partial factor productivity (PFP), especially for CMV3. The AE and PFP values of double rice for CMV3 increased by 64.54% and 25.76%, respectively. The application of CMV also increased the sustainability index and reduced the coefficient of variation of the rice yields. In addition, there was negative linear relationship between the amount of CMV needed to generate higher yields of double rice and the years of CMV application. In conclusion, CMV addition at 15,000–22,500 kg ha−1 combined with mineral fertilizer had beneficial effects on the productivity, the stability of rice yield as well as the fertilizer efficiency in double rice cropping system.
1. Introduction
Rice is a staple food for more than half of the global population, and its paddies cover 11% of the planet’s arable land (Qian et al. Citation2020). The main focus of the current agricultural approach in China is on improving the yield per hectare and increasing comprehensive productivity to maintain the stable and sustainable growth of rice crops (Xie et al. Citation2017). Fertilization is an important method in improving soil fertility and crop yields; however, traditional fertilization causes potential environmental threats, such as soil degradation and freshwater eutrophication (Zhou et al. Citation2020; Xie et al. Citation2016). Therefore, many new approaches have been explored to achieve the ideal outcome of ensuring food security and shrinking agriculture’s environmental footprint.
One such strategy to reduce mineral fertilizer inputs, while stabilizing or increasing crop yield, is the use of natural resources as a substitute. Green manure is an important natural resource. Previous studies have shown that the incorporation of green manure increased the yields of double rice and the sustainable yield index (Xie et al. Citation2016; Wang et al. Citation2018). Green manure improved the soil's physical, biological properties, and soil fertility and influenced the soil carbon and nitrogen cycling (Yang et al. Citation2014; Yu et al. Citation2020; Zhou et al. Citation2020). And the planting of green manure was helpful to inhibit the density of the paddy weed seed bank and main weed seed species (Norsworthy et al. Citation2005; Samarajeewa, Horiuchi, and Oba Citation2005). In addition, the application of green manure may enhance the nitrogen use efficiency by improving the N supplying capacity and reducing the N fertilizer input (Xie et al. Citation2016; Yang et al. Citation2019).
Other previous studies have confirmed that green manure application plays an important role in ameliorating the paddy soil physical and chemical properties, leading to improvements in the soil quality and soil nutrient capacity (Martínez-Mena et al. Citation2020; Adekiya et al. Citation2019; Ghosh et al. Citation2020; Yadav, Dwivedi, and Pandey Citation2000; Yadav Citation1995; Pypers et al. Citation2005). In addition, green manure from legumes provides N to soils through biological N fixation and increase the soil N supply to subsequent crops (Thorup-Kristensen, Magid, and Jensen Citation2003). Other studies indicated that green manure is an efficient way to promote C sequestration and distribution (Yao et al. Citation2019; Yang et al. Citation2014). Moreover, the application of green manure transferred nutrients, which were then stored in soils (e.g., N, P, K, Ca, Mg, and Si) and improved the decomposition rate of the indigenous soil organic nitrogen and carbon, which are all critical factors for the growth of succeeding crops (Yao et al. Citation2019; Pypers et al. Citation2005; Zhou et al. Citation2019). Soil enzyme activities reflect the intensity and direction of nutrient cycling and are reactive to minute variations (Ghosh et al. Citation2020), therefore they are used as a key indicator of soil fertility and soil quality (Dai et al. Citation2019). It has been reported that green manure application can also improve the soil enzymatic activities by affecting the soil microbial biomass, soil microbial activity as well as the community composition (Zhou et al. Citation2020).
Chinese milk vetch (CMV, Astragalus sinicus L.) is a common winter green manure in the southern rice region of China and has been widely applied for subsequent crops as a nutrient source in rice cropping systems using the crops for rotation (Zhu et al. Citation2014; Yang et al. Citation2014). However, there is little knowledge about the optimum amount of CMV incorporated with chemical fertilizer in the double cropping rice system in East China by long-term CMV application. Especially, it has not been evaluated fully that how the demand of CMV applied had changed with the increase of the years of CMV application in double rice system.
So the present study analyzed the effects of different amounts of CMV combined with chemical fertilizer on the yield of rice, fertilizer efficiency, and the fertility, the enzymatic activities of paddy soil after 11 years of continuous CMV application. And then the optimum amount of CMV was explored at higher rice yield and soil fertility and the change of the demand of CMV applied was also evaluated with the increase in the years of continuous CMV application. These results would be helpful to the reasonable utilization of CMV in the lower and middle Yangtze, East China.
2. Materials and methods
2.1. Site and soil conditions
The long-term field experiment was conducted in Anqing city, Anhui province, China (31º06′26.46″N, 117º04′43.62″E) and began in 2008. This location has a humid subtropical monsoon climate with mean annual air temperature of 16.0°C and average annual precipitation of 1250 mm. The mean duration of annual sunshine is 1903 h and the average frost-free period is 121 days. The annual temperature, the accumulative temperature (≥10°C), the average precipitation, and the average sunshine time in the period of early rice were 23.5°C, 2140°C, 518 mm, and 466.1 h, respectively. And the annual temperature, the accumulative temperature (≥10°C), the average precipitation, and the average sunshine time in the period of late rice were 17.3°C, 2422°C, 467 mm, and 721.5 h, respectively. The soil is a weakly acidic (pH 5.52) paddy soil developed from river alluvium. Prior to the experiment, the chemical properties of surface layer soil (0–20 cm) were as follows: organic matter (SOM), 27.09 g kg−1; total nitrogen (TN), 1.72 g kg−1; available phosphorus (AP), 8.87 mg kg−1; and available potassium (AK), 79.49 mg kg−1.
2.2. Experimental design
Each plot was 2 m × 10 m. Experimental plots were separated by a 30 cm × 20 cm ridge aboveground, which was covered with plastic film to prevent the movement of water and nutrients between plots. The following six experimental treatments were randomly assigned to the plots with four field replications: Ck (no NPK mineral fertilizer and no CMV); CMV0 (NPK mineral fertilizers and no CMV); CMV1 (NPK mineral fertilizers and 7500 kg ha−1 CMV); CMV2 (NPK mineral fertilizers and 15,000 kg ha−1 CMV); CMV3 (NPK mineral fertilizers and 22,500 kg ha−1 CMV); and CMV4 (NPK mineral fertilizers and 30,000 kg ha−1 CMV).
The amount of applied chemical fertilizers to rice were: 105 kg ha−1 N (urea, 464 g kg−1 N), 52.5 kg ha−1 P2O5 (superphosphate, 120 g kg−1 P2O5), and 63 kg ha−1 K2O (potassium chloride, 600 g kg−1 K2O) for early rice; and 180 kg ha−1 N (urea, 464 g kg−1 N), 75 kg ha−1 P2O5 (superphosphate, 120 g kg−1 P2O5) and 120 kg ha−1 K2O (potassium chloride, 600 g kg−1 K2O) for late rice. For urea application, 50% of N was broadcasted as basal application, while 30% of N was top-dressed at the tillering stage, and another 20% of N was top-dressed at the panicle initiation stage. All P and K fertilizers were consistently applied as basal fertilizers. Rice seedlings were transplanted at the spacing of 18.3 cm×20.3 cm (early rice) and 16.5 cm ×20.0 cm (late rice). Early rice was transplanted in early May and harvested in the mid-July each year. Late rice was transplanted in the last week of July and harvested in the first week of December.
CMV (Astragalus sinicus L. cv. Yijiangzi, Anhui) was directly seeded without tillage 20 days earlier than the date of the harvest of late rice in the winter season (except Ck and CMV0). Fresh CMV was harvested and measured sequentially every year at the full-bloom stage in the middle of April. The fresh whole plants of CMV were mixed mechanically within 15–20 cm soil depth, and was flooded up to 5–10 cm depth for 15 days before the early rice transplanting. In the CMV1–4, the whole crop of CMV was applied for the following early rice season at the rates of 7500, 15,000, 22,500, and 30,000 kg ha−1, respectively. The dry green manure contained 467.10 g kg−1 total organic carbon, 21.10 g kg−1 total N, 2.05 g kg−1 total P, and 13.24 g kg−1 total K (multi-year average values). The rice straw was removed from every experiment plot after harvesting the rice field.
2.3. Rice grain yield measurement and soil analysis
At the maturity of early and late rice, grains were separated from the straw. Subsequently, the grains were sun-dried and weighed separately to obtain the annual yield every year. The yield was calibrated according to the entire plot area for both early and late rice from 2008 to 2018. The soil samples located at 0–20 cm depth were collected in December 2018 after harvesting late rice by using a 10 cm diameter soil auger. A total of five soil cores were collected per plot. Each sample was then divided into two parts: one part was frozen for enzyme analysis and the other was air-dried and ground for the determination of soil chemical properties. The contents of SOM, TN, AP, and AK were determined according to the methods described by Bao (Citation2000). Soil pH was measured with a compound electrode (FE20, Mettler-Toledo Instruments, Shanghai, China) at a soil-to-water ratio of 1:2.5. The activities of invertase, urease, catalase, cellulose, and acid phosphatase were measured according to the methods described by Guan (Citation1986). Soil invertase, urease, catalase, cellulose, and acid phosphatase were expressed as mg glucose g−1 24 h−1, mg NH4+-N g−1 24 h−1, μmol H2O2 g−1 24 h−1, mg glucose g−1 24 h−1, and μmol phenol g−1 24 h−1.
2.4. Statistical analysis
The unary quadratic yield response models were used to describe the relationship between the amount of CMV applied and rice yield responses (Valkama, Uusitalo, and Turtola Citation2011). The unary quadratic model was applied as follows:
where Y (kg ha−1) was the yield of double rice every year, c is the yield of rice at CMV = 0, a and b were fitted constants, and x (kg ha−1) was the amount of CMV applied every year.
The sustainable yield index (SYI) were obtained relative to the maximum observed yield over the years under the double rice cropping system (Yadav, Dwivedi, and Pandey Citation2000). The yield stability was evaluated with the coefficient of variation (CV). The higher the SYI value, the more sustainable the system, and the lower the CV value, the more stable the system. The SYI and CV were calculated as follows.
where (kg ha−1) is the average yield for 11 years,
(kg ha−1) is the maximum yield obtained under that treatment in any year and
is the standard deviation of yield for specific treatment across years.
The agronomic efficiency (AE) and the partial factor productivity (PFP) of applied fertilizer were calculated as follows.
where (kg ha−1) is the rice grain average yield of the fertilized treatment,
(kg ha−1) is the rice grain yield (kg ha−1) without fertilizer application and F (kg ha−1) is the amount of chemical fertilizer applied.
Values obtained from different treatments were subjected to ANOVA tests. Separation of means was performed on significant ANOVA tests by Duncan’s multiple range test (p < 0.05) using SPSS 20.0. Data followed by different letters in columns are significant among treatments at the 5% level. For the quadratic model, the data were analyzed by SigmaPlot 10.0 program. The graphs and tables in this study were generated by Origin 8.5 and Excel 2010, respectively.
3. Results
3.1. Soil chemical properties
After the 11 years, the SOM in CMV1–4 were significantly higher than those of Ck (24.39 g kg−1) (). When the amount of CMV applied exceeded 15,000 kg ha−1, all the SOM in CMV2–4 were also notably higher than that of CMV0 by 11.33%-15.99%. Moreover, compared with the initial soil (27.09 g kg−1), the SOM by application of CMV (CMV1–4) were increased by 10.41%-16.76% after 11 years, and were increased by an average of 0.256–0.413 g kg−1 per year. The TN of soil in CMV0 was 1.61 ± 0.03 g kg−1 and the TN of CMV2-4 were significantly higher than CMV0 by 16.15%, 26.71%, and 24.22%, respectively. The TN was also increased with the increase of the addition amount of CMV at the same nitrogen fertilizer applied. Compared with the TN of the initial soil (1.72 g kg−1), the TN in both Ck and CMV0 were reduced and the TN in Ck was lower than that of the initial soil by 0.25 g kg−1. In CMV1–4, the TN was increased by 1.16%-18.60% compared with that of the initial soil. The TN in CMV3 was increased by an average of 0.03 g kg−1 per year.
Table 1. Mean soil organic matter (SOM), the total nitrogen (TN), the available phosphorus (AP), the available potassium (AK) and pH in the 0-20 cm soil layer among all the treatments after harvesting of late rice in 2018 (n=4)
The AP in Ck was not only the lowest among all the treatments, but was also lower than the initial soil. The AP was decreased by 0.60 mg kg−1 per year when there was no nutrient input during the 11-year period. Although the chemical fertilizer level was the same among CMV0–4, the AP values in CMV1–4 were significantly higher than that of CMV0. The AK in Ck was also lowest among all treatments. Compared with CMV0, the application of CMV had no significant effects on the AK and chemical fertilizer application might play a more important role in the content of AK in paddy soils. Furthermore, the pH value in Ck was the highest among all treatments and the input of chemical fertilizer or CMV decreased the pH values. Moreover, all the pH values in CMV1–4 were higher than that of CMV0. It implied that the application of CMV at least partially contributed to preventing the reduction of pH caused by the chemical fertilizer in the paddy soil.
3.2. Soil enzyme activity
The soil invertase activities in CMV1–4 were higher than those of CMV0 and there were significant differences detected at CMV2–4 compared with CMV0 (). The invertase activities of CMV3 was higher significantly than that of CMV0 by 23.52%. The soil urease activity showed a similar trend to the soil invertase activities among all treatments (). The urease activity in Ck and CMV3 was the lowest and the highest among all the treatments, respectively. The addition of CMV promoted the activity of urease when compared with CMV0. Similarly, the lowest soil catalase activity was found in Ck and the soil catalase activities in CMV1–4 were higher than those in CMV0 by 5.17%-22.20% (). The soil catalase activity was associated with the amount of CMV and the highest value was found in CMV4. The acid phosphatase activities in Ck were lowest among all treatments, and the addition of chemical fertilizer only (CMV0) and CMV application (CMV1–4) both increased the phosphatase activities (). And the acid phosphatase activities in CMV1–4 were higher than those in CMV0 (chemical fertilizer only) by 3.41%-18.49%. However, there were no significant differences of the activities of cellulase among the treatments (p > 0.05) ().
3.3. Rice grain yield
The 11 years of yield data were collected from 2008 to 2018. As shown in , the annual rice yields of all fertilizer treatments were higher than those of Ck. The annual rice yields of Ck were significantly lower than those of all the fertilized treatments, indicating that fertilization management is beneficial to enhance the yields of rice. Specifically, the average annual yields of early rice decreased in the order CMV3 > CMV2 > CMV4 > CMV1 > CMV0 > Ck, whereas the yields of late rice decreased in the order CMV3 > CMV4 > CMV2 > CMV1 > CMV0 > Ck. The average grain yields ranged from 2887 to 6020 kg ha−1 for early rice (), from 3003 to 6307 kg ha−1 for late rice () and from 5890 to 12,327 kg ha−1 for double rice (). The yields of CMV1–4 were all higher than the yield of CMV0, and the application of CMV improved the yields of early rice, late rice, and double rice. The yields of early rice, late rice, and double rice were highest in CMV3, though there was no significant difference among CMV2–4. In addition, a reduction in rice yields was exhibited in CMV4 when compared with CMV3, and there was no significant difference between CMV3 and CMV4.
Figure 2. The rice yields for early rice (a), late rice (b) and double rice (early rice and late rice, c) of these treatments as influenced by various amount of CMV applied from 2008 to 2018 (Different letters in a figure mean significance among treatments at the 5% level, n=11)
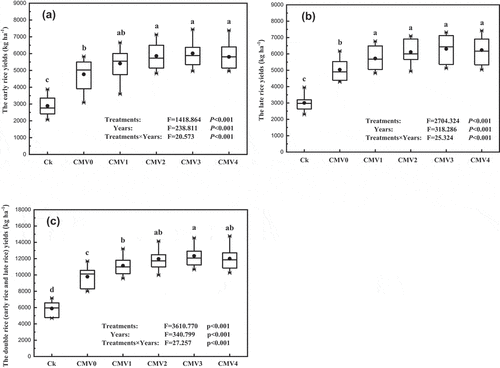
The trends of annual rice yield in the double rice cropping system generally reflected changeable climate conditions, different rice varieties, and variable pest and weed control practices. An ANOVA for the yields indicated significant treatment × year interaction in early rice, late rice, and double rice.
The relationship between the amount of CMV applied and the yield of double rice was analyzed by the unary quadratic equation well (Y = c+ ax+bx2, r2 > 0.95, p < 0.05) and the association was at a significant level in the regression analysis (). The rational amount of CMV applied was estimated according to the unary quadratic equation at the highest yield of double rice achieved each year (). When combined with the years of CMV application, there was a significant linear negative correlation (r2 = 0.331, p = 0.037*) between the years of CMV applied and the rational amount of CMV (). It was exhibited that the required amount of CMV to improve rice yield was reduced with the year of CMV applied in the double rice system.
Table 2. CMV application rate at the maximum rice yield of double rice of each year calculated by unary quadratic equation with the coefficients of determination and the p values
3.4. The SYI and CV values of early and late rice as well as the double rice yields
For the yield stability, the highest CV value occurred in Ck in all early rice, late rice, and double rice (). In early rice, the CV values decreased with an increase of CMV, and the addition of CMV improved the stability of the early rice yield. The presence of CMV increased the SYI values in CMV1–4 compared with CMV0 in early rice. In late rice, all CV values of CMV1–4 were lower, and all the SYI values of CMV1–4 were higher compared with CMV0. For double rice, the trends of the CV values and the SYI values were similar to those in late rice. Therefore, the application of CMV was also beneficial to improve the stability of double rice. The CV and SYI data revealed that the amount of CMV at 15,000–22,500 kg ha−1 combined with chemical fertilizer provided optimal stability of the yields in early rice, late rice, and double rice. In addition, the SYI values in late rice were higher than those in early rice and the CV in late rice was lower than those in early rice. It indicated that late rice yields were more sustainable and stable than those of early rice. It may be due to that the weather conditions of the vegetative growth stage in early rice which were worse than that in late rice. And the instability of extreme weather condition occurred in the period of early rice was also higher than that in the period of late rice. These may not be conducive to the yield and the stability, especially to the returning green stage and the tillering stage of the early rice planting.
Table 3. The coefficient of yield variation (CV %) and the sustainable yield index (SYI %)of early and late rice as well as double rice in the double rice system (2008-2018, n=11)
3.5. The efficiency of fertilizer in the early, late rice and double rice
The AE and PFP in all treatments are shown in . For early rice, all the AE and PFP values of CMV1–4 were significantly higher than those of CMV0, within the ranges 34.15%-66.57% and 13.44%-26.23%, respectively. Similar results were also found in late rice and double rice. It indicated that the application of CMV enhanced the utilization rate of chemical fertilizer. Interestingly, the AE and PFP values of all treatments in late rice were lower than those of the corresponding treatments in early rice ().
Table 4. Fertilizer use efficiency for early rice, late rice and double rice, as influenced by various amount of CMV application in the double rice system (2008-2018, n=11)
4. Discussion
Soil fertility and rice production are two major indicators used for the sustainability measurement of rice systems (Xie et al. Citation2016). In our study, the input of CMV enhanced the concentration of SOM, TN, AP, and AK after cultivation (). The continuous application of CMV improved SOM compared with CMV0. The CMV returned not only increased the carbon input, but also influenced rice crop stubbles, which brought the benefits of agricultural organic matter sequestration. According to previous research, the green manure application improved the porosity and soil bulk density, which favored the accumulation of organic carbon (Zhou et al. Citation2019). For CMV0, NPK fertilizer did not increase SOM since it did not contain organic matter or decaying matter, which are necessary for soil structure improvement. The cultivation of CMV significantly enhanced the soil TN, especially for CMV3 and CMV4. It has been shown that CMV can fix atmospheric nitrogen to return it to paddy soil, which could cause a net gain of N and 62.62 kg ha−1 N at the amount of 22,500 kg ha−1 CMV (containing 21.1 g kg−1 of N in dry matter of CMV) (). In our case, when the amount of CMV returned reached 22,500 kg ha−1, an input of 62.62 kg ha−1 N would be also returned to the paddy soil, which could remarkably improve annual N conservation and recovery and increase the N content. In addition, it has been shown that CMV decomposition also improved the NH4+-N content and released more N on its return to paddy soil to feed the growth of rice and assist in N absorption and accumulation (Xie et al. Citation2017; Zhou et al. Citation2019). Therefore, the return of CMV introduced extra N to the soil after being plowed in. The AP was also increased with CMV input and the return of CMV enhanced the AP in paddy soil by 21.13%-28.68% (). Incorporated CMV in soil went through the decomposition process and produced organic acid, which can promote P availability by competing with P via anion exchange, blocking P adsorption sites (Pypers et al. Citation2005). Moreover, the organic acids can also solubilize certain P-containing primary minerals to improve the P availability overall (Mitran et al. Citation2016; Pypers et al. Citation2005). Moreover, the degradation of CMV can increase the activities of the P-cycling enzymes such as acid phosphatase as shown in our study () and others (e.g., alkaline monophosphoesterase and phosphodiesterase) (Nannipieri et al. Citation2012) to facilitate the availability of P in paddy soil. Although there was a slightly lower soil pH after CMV application compared with Ck because of the production of CO2, acidic deposition, and organic acids during the decomposition process (Adekiya et al. Citation2019), the continuous returns of CMV diminished the trend of the pH reduction resulting from chemical fertilizer application (CMV0) (). This may be because the decomposition of CMV releases macronutrients (e.g., Ca, Mg and K) that neutralize soil acidity (Yadav, Dwivedi, and Pandey Citation2000; Zhou et al. Citation2019). The soil enzymes reflect the nutrient cycling activities and act as critical indicators of soil fertility levels in multiple agricultural systems (Zhou et al. Citation2020). In our study, the continuous application of CMV significantly improved the activities of invertase, catalase, and urease (). This may be due to that the application of CMV can simulate the amounts and activities of soil microbiological biomass (increase microbial proliferation) and enzymatic activity through the modification of soil physical properties and the addition of C and N sources (Fraser et al. Citation1988), as well as an increase in activity of plant-origin enzymes (Piotrowska and Wilczewski Citation2012). Moreover, acid phosphatase plays an important role in the mineralization of organic phosphorous and the release of inorganic phosphorous (Mndzebele et al. Citation2020), our experiment showed that the application of CMV enhanced the activity of acid phosphatase, which could influence the P availability.
Our results also showed that CMV could dramatically increase the yields by 13.46%-26.23%, 13.63%-25.31%, and 13.55%-25.76% in early, late and double rice in the double rice system, respectively, compared with CMV0 (). Our results were consistent with those of previous studies (Xie et al. Citation2016; Zhou et al. Citation2019; Liu et al. Citation2019) and confirmed that CMV application had beneficial effects on the yields of early, late and double rice. Our data supported that the CMV combined with nitrogen fertilizer might provide timely available nutrients for rice growth. Moreover, the cooperation between chemical fertilizer and CMV can synergize the yields of rice by promoting yield components such as effective panicle number, gain number per spikelet, and 1000-grain weight (Liu et al. Citation2019).
In addition to the fertilization and yield, we also checked SYI and CV for the sustainability and stability of crop production (Chaudhury et al. Citation2005). In our experiment, the chemical fertilizer with CMV at more than 15,000 kg ha−1 improved the SYI values and the CV values compared with CMV0, especially for early rice (). This implies that CMV application might have more positive effects on the sustainability and stability of early rice than in late rice. These findings might be related to the rapid decomposition of CMV with a low C/N ratio, and it could afford more available nutrient for early rice. For late rice, the continuous positive effect of CMV decomposition was mainly revealed by improving the fertility and quality of paddy soil. And there may be the higher dosage of chemical fertilizer on late rice than that on early rice which may mask the positive effect of organic materials partly on late rice. Zhou et al. (Citation2020) had also shown that the beneficial effects of green manure in early rice yield (the loading score 0.72) were greater than those in late rice yield (the loading score 0.22). We found that CMV improved the efficiency of chemical fertilizers (AE and PFP) (). This was because that the CMV contained N, P2O5 and K2O, which were the same nutrients as chemical fertilizer for rice growth, especially in terms of N fertilizer.
Based on the average yield of 11 years, the yields of early rice, late rice and double rice all increased with the application of CMV from 0 to 22500 kg ha−1. However, the reduction of the yields of early, late rice and double rice were found at 30000 kg ha−1 CMV application, though there was no statistical difference among CMV2, CMV3 and CMV4. The similar trends were also found in the values of CV, SYI and the efficiency of fertilizers (AE and PFP). This could be due to that the degradation of excessive green manure might induce the generation of active organic reductants, and produce too much organic acid, H2S, which compromised the growth of rice (Li and Han Citation1966; Liu et al. Citation2012).
Furthermore, there was a significant linear negative correlation between the years of CMV application and the rational amount of CMV at the maximum yield of double rice. These findings suggested the amount of CMV required was reduced with the year of CMV applied when the high yield of double rice reached. There were two reasons as follow. On the one hand, it was due to that the application of CMV can improve the soil fertility () and soil enzymes activities () and enhance soil C, N and P cycling with the increase of the CMV applied. And the CMV applied can also provide N to soils through biological N2 fixation and increase the soil N supply to rice (Zhou et al. Citation2020). On the other hand, with the long-term CMV application, there may be enough nutrients from paddy soil for rice growth. And if more than the demand of the application of CMV was incorporated into the paddy soil continuously, there may be excessive active organic reductants, such as organic acid, H2S from paddy soil with easily decomposable organic nutrient accumulated (Li and Han Citation1966). There would be the negative effect of large amount of CMV application on the rice production.
After 11 years of continuous CMV application in our field experiment, the optimal amount of CMV was 15,000–22,500 kg ha−1 based on the yields of early and late rice, the efficiency of fertilizers, and the soil biochemical properties. We found that CMV application generated beneficial effects in terms of improving soil fertility and the amount of CMV needed to be decreased with the years of CMV application. Since CMV is a natural resource and is not associated with contamination, it can be applied continuously to enhance the soil fertility and the crop yields over a wide area. However, when CMV was applied at 30,000 kg ha−1, there were reductions in rice yield, rice yield stability and sustainability, and the efficiency of fertilizers. Therefore, we recommend that 15,000–22,500 kg ha−1 CMV application combined with chemical fertilizer can be an effective fertilization management in the lower and middle Yangtze region, East China.
5. Conclusion
After 11 years of continuous CMV application, CMV combined with chemical fertilizer demonstrated improvement of TN, SOM, AP and reduced the soil pH decline, which otherwise could be caused by chemical fertilizer application alone. The cultivation of CMV also improved the activities of soil enzymes (except for soil cellulase) in the paddy soil. Meanwhile, the CMV input increased the yields of early, late, and double rice in the double rice system as well as the sustainability and stability of rice production, especially at 15,000–22,500 kg ha−1 CMV return. The CMV application also enhanced the efficiency of chemical fertilizer. In addition, the amount of CMV required for maximum double rice yield could be reduced with the years of CMV application and thus the long-term application of CMV had brought positive effects on the productivity of the paddy soil. In conclusion, CMV application at 15,000–22,500 kg ha−1 combined with chemical fertilizer is an effective management approach to improve productivity, sustainability, and stability of rice yield as well as the fertilizer efficiency in early, late, and double rice in the double rice system in the lower and middle Yangtze region, East China.
Acknowledgments
This work was supported by National Natural Science Foundation of China (Grant No. 41807106) and China Agriculture Research System Green Manure (CARS-22). We thank Alex Boon, PhD, from Liwen Bianji, Edanz Editing China (www.liwenbianji.cn/ac), for editing the English text of a draft of this manuscript.
Disclosure statement
No potential conflict of interest was reported by the authors.
Additional information
Funding
References
- Adekiya, A. O., T. M. Agbede, C. M. Aboyeji, O. Dunsin, and J. O. Ugbe. 2019. “Green Manures and NPK Fertilizer Effects on Soil Properties, Growth, Yield, Mineral and Vitamin C Composition of Okra (Abelmoschus Esculentus (L.) Moench).” J Saudi Soc Agr Sci. 18: 218–223. doi:10.1016/j.jssas.2017.05.005.
- Bao, S.D. 2000. Soil and Agricultural Chemistry Analysis. Beijng: China Agricultural Press. (in Chinese)
- Chaudhury, J., U. K. Mandal, K. L. Sharma, H. Ghosh, and B. Mandal. 2005. “Assessing Soil Quality under Long-term Rice-based Cropping System.” Commun Soil Sci Plant 36 (9–10): 1141–1161. doi:10.1081/CSS-200056885.
- Dai, X. L., W. Zhou, G. R. Liu, G. Q. Liang, P. He, and Z. B. Liu. 2019. “Soil C/N and pH Together as a Comprehensive Indicator for Evaluating the Effects of Organic Substitution Management in Subtropical Paddy Fields after Application of High-quality Amendments.” Geoderma 337: 1116–1125. doi:10.1016/j.geoderma.2018.11.023.
- Fraser, D. G., J. W. Doran, W. W. Sahs, and G. W. Lesoing. 1988. “Soil Microbial Populations and Activities under Conventional and Organic Management.” J Environ Qual. 17 (4): 585–590. doi:10.2134/jep1988.00472425001700040011x.
- Ghosh, A., A. B. Singh, R. V. Kumar, M. C. Manna, R. Bhattacharyya, M. M. Rahman, P. Sharma, P. S. Rajput, and S. Misra. 2020. “Soil Enzymes and Microbial Elemental Stoichiometry as Bio-indicators of Soil Quality in Diverse Cropping Systems and Nutrient Management Practices of Indian Vertisols.” Appl Soil Ecol. 145: 103304–103316. doi:10.1016/j.apsoil.2019.06.007.
- Guan, S. Y. 1986. Soil Enzyme and Its Research Method. Beijing: Agricultural Press. (in Chinese)
- Li, X. Y., and D. Q. Han. 1966. “The Dynamical Equilibrium of Soil Reductive Substances after Green-manuring for Rice Culture.” Acta Pedol Sin. 14 (1): 59–64. in Chinese.
- Liu, X. C., H. Y. Zhao, B. Y. Li, Z. C. Lu, D. Q. Feng, and L. Qiao. 2012. “Effects of the Chinese Milk Vetch’s Amount and Retting Time in Rice Regreening Plant by Covered with Plastic Film”. Plant Nutr Fert Sci. (3):90–93. in Chinese.
- Liu, Y., H. Y. Tang, A. Muhammad, and G. Q. Huang. 2019. “The Effects of Chinese Milk Vetch Returning with Nitrogen Fertilizer on Rice Yield and Greenhouse Gas Emissions.” Green Gases. 9 (4): 743–753. doi:10.1002/ghg.1892.
- Martínez-Mena, M., E. Carrillo-López, C. Boix-Fayos, M. Almagro, N. García Franco, E. Díaz-Pereira, I. Montoya, and J. de Vente. 2020. “Long-term Effectiveness of Sustainable Land Management Practices to Control Runoff, Soil Erosion, and Nutrient Loss and the Role of Rainfall Intensity in Mediterranean Rainfed Agroecosystems.” Catena 187: 104352–104364. doi:10.1016/j.catena.2019.104352.
- Mitran, T., P. K. Mani, N. Basak, D. Mazumder, and M. Roy. 2016. “Long-term Manuring and Fertilization Influence Soil Inorganic Phosphorus Transformation Vis-a-vis Rice Yield in a Rice-wheat Cropping System.” Arch Agron Soil Sci. 62 (1): 1–18. doi:10.1080/03650340.2015.1036747.
- Mndzebele, B., B. Ncube, M. Fessehazion, T. Mabhaudhi, S. Amoo, C. Du Plooy, S. Venter, and A. Modi. 2020. “Effects of Cowpea-amaranth Intercropping and Fertiliser Application on Soil Phosphatase Activities, Available Soil Phosphorus, and Crop Growth Response.” Agronomy 10 (1): 79–98. doi:10.3390/agronomy10010079.
- Nannipieri, P., L. Giagnoni, G. Renella, E. Poglisi, B. Ceccanti, G. Masciandaro, F. Fornasier, M. C. Moscatelli, and S. Marinari. 2012. “Soil Enzymology: Classical and Molecular Approaches.” Biol Fert Soils 48 (7): 743–762. doi:10.1007/s00374-012-0723-0.
- Norsworthy, J. K., L. Brandenberger, N. R. Burgos, and M. Riley. 2005. “Weed Suppression in Vigna Unguiculata with a Spring-seeded Brassicaceae Green Manure.” Crop Protection (Guildford, Surrey) 24 (5): 441–447. doi:10.1016/j.cropro.2004.09.015.
- Piotrowska, A., and E. Wilczewski. 2012. “Effects of Catch Crops Cultivated for Green Manure and Mineral Nitrogen Fertilization on Soil Enzyme Activities and Chemical Properties” Geoderma 189–190. 72-80. doi: 10.1016/j.geoderma.2012.04.018
- Pypers, P., S. Verstraete, C. P. Thi, and R. Merckx. 2005. “Changes in Mineral Nitrogen, Phosphorus Availability and Salt-extractable Aluminium following the Application of Green Manure Residues in Two Weathered Soils of South Vietnam.” Soil Biol Biochem. 37 (1): 163–172. doi:10.1016/j.soilbio.2004.06.018.
- Qian, H. Y., S. Huang, J. Chen, L. Wang, B. A. Hungate, C. van Kessel, J. Zhang, A. X. Deng, Y. Jiang, and V. G. K J. 2020. “Lower-than-expected CH4 Emissions from Rice Paddies with Rising CO2 Concentrations.” Global Change Biol. 26 (4): 2368–2376. doi:10.1111/gcb.14984.
- Samarajeewa, K. B. D. P., T. Horiuchi, and S. Oba. 2005. “Weed Population Dynamics in Wheat as Affected by Astragalus Sinicus L. (Chinese Milk Vetch) under Reduced Tillage.” Crop Protection (Guildford, Surrey) 24 (10): 864–869. doi:10.1016/j.cropro.2005.01.018.
- Thorup-Kristensen, K., J. Magid, and L. S. Jensen. 2003. “Catch Crops and Green Manures as Biological Tools in Nitrogen Management in Temperate Zones.” Adv Agron. 79: 227–302. doi:10.1016/s0065-2113(02)79005-6.
- Valkama, E., R. Uusitalo, and E. Turtola. 2011. “Yield Response Models to Phosphorus Application: A Research Synthesis of Finnish Field Trials to Optimize Fertilizer P Use of Cereals.” Nutr Cycl Agroecosyst 91 (1): 1–15. doi:10.1007/s10705-011-9434-4.
- Wang, H., S. Tang, J. Wu, R. Hu, S. Han, Y. Liu, Q. Zhu, M. Li, Y. Q. Wang, and Z. L. Shi. 2018. “Effects of Nitrogen Managements with Incorporation of Chinese Milk Vetch on the Yield of Double Cropping Rice and Fertilizer Use Efficiency.” J Agr Resour Environ 35 (4): 327–333. in Chinese. doi10.13254/j.jare.2017.0321.
- Xie, Z. J., S. X. Tu, S. Farooq, C. X. Xu, J. R. Chen, D. Han, G. R. Liu, H. L. Li, M. Imtiaz, and W. D. Cao. 2016. “Substitution of fertilizer-N by Green Manure Improves the Sustainability of Yield in Double-rice Cropping System in South China.” Field Crop Res. 188: 142–149. doi:10.1016/j.fcr.2016.01.006.
- Xie, Z. J., Y. Q. He, S. X. Tu, C. X. Xu, G. R. Liu, H. M. Wang, W. D. Cao, and H. Liu. 2017. “Chinese Milk Vetch Improves Plant Growth, Development and 15N Recovery in the Rice-based Rotation System of South China.” Sci Rep-UK 7 (1): 3577–3587. doi:10.1038/s41598-017-03919-y.
- Yadav, R. L. 1995. “Soil Organic Matter and NPK Status as Influenced by Integrated Use of Green Manure, Crop Residues, Cane Trash and Urea N in Sugarcane-based Crop Sequences.” Bioresource Technology 54 (2): 93–98. doi:10.1016/0960-8524(95)-00084-4.
- Yadav, R. L., B. S. Dwivedi, and P. S. Pandey. 2000. “Rice-wheat Cropping System: Assessment of Sustainability under Green Manuring and Chemical Fertilizer Inputs.” Field Crop Res 65 (1): 15–30. doi:10.1016/S0378-4290(99)00066-0.
- Yang, L., X. Zhou, Y. L. Liao, Y. H. Lu, J. Nie, and W. D. Cao. 2019. “Co-incorporation of Rice Straw and Green Manure Benefits Rice Yield and Nutrient Uptake.” Crop Science 59(2): 1–11. doi:10.2135/cropsci2018.07.0427.
- Yang, Z. P., S. X. Zheng, J. Nie, Y. L. Liao, and J. Xie. 2014. “Effects of Long-term Winter Planted Green Manure on Distribution and Storage of Organic Carbon and Nitrogen in Water-stable Aggregates of Reddish Paddy Soil under a Double-rice Cropping System.” J Integ Agr. 13 (8): 1772–1781. doi:10.1016/S2095-3119(13)60565-1.
- Yao, Z. Y., D. B. Zhang, N. Liu, P. W. Yao, N. Zhao, Y. Y. Li, S. Q. Zhang, et al. 2019. “Dynamics and Sequestration Potential of Soil Organic Carbon and Total Nitrogen Stocks of Leguminous Green Manure-based Cropping Systems.” Soil Till Res. 191: 108–116. doi:10.1016/j.still.2019.03.022.
- Yu, Q. G., X. Hu, J. W. Ma, J. Ye, W. C. Sun, Q. Wang, and H. Lin. 2020. “Effects of Long-term Organic Material Applications on Soil Carbon and Nitrogen Fractions in Paddy Fields.” Soil Till Res. 196: 104483–104489. doi:10.1016/j.still.2019.104483.
- Zhou, G. P., S. J. Gao, Y. H. Lu, Y. L. Liao, J. Nie, and W. D. Cao. 2020. “Co-incorporation of Green Manure and Rice Straw Improves Rice Production, Soil Chemical, Biochemical and Microbiological Properties in a Typical Paddy Field in Southern China.” Soil Till Res. 197: 104499–104507. doi:10.1016/j.still.2019.104499.
- Zhou, X., Y. H. Lu, Y. L. Liao, Q. D. Zhu, H. D. Cheng, X. Nie, W. D. Cao, and J. Nie. 2019. “Substitution of Chemical Fertilizer by Chinese Milk Vetch Improves the Sustainability of Yield and Accumulation of Soil Organic Carbon in a Double-rice Cropping System.” J Integ Agr. 18 (10): 2381–2392. doi:10.1016/S2095-3119(18)62096-9.
- Zhu, B., L. X. Yi, Y. G. Hu, Z. H. Zeng, C. W. Lin, H. M. Tang, G. L. Yang, and X. P. Xiao. 2014. “Nitrogen Release from Incorporated 15N-labelled Chinese Milk Vetch (Astragalus Sinicus L.) Residue and Its Dynamics in a Double Rice Cropping System.” Plant Soil. 374: 331–344. doi:10.1007/s11104-013-1808-8.