ABSTRACT
Nitrogen (N) fixation by rhizobia plays an important role in N assimilation of soybean (Glycine max). Nitrogenase, the enzyme responsible for N fixation in rhizobia, contains a large amount of sulfur (S) molecules and requires phosphorus (P) in adenosine triphosphate. Thus, S and P are essential for plant growth and N fixation by rhizobia. However, P in soils forms insoluble PO43 – salts with metals. Plant roots solubilize these PO43- salts by secreting organic acids and absorb solubilized PO43-. In this study, the effects of S application on P solubilization by soybean roots and the involvement of rhizobia were evaluated. Soybean plants inoculated with Bradyrhizobium diazoefficiens USDA110 were grown in vermiculite, and Ca3(PO4)2 was added as the sole P source. A hydroponic solution supplemented with three S concentrations (0, 20, and 1000 µM) was applied, and plants were grown for 5 weeks. Root fresh weight, the soil-plant analysis development (SPAD) values, and shoot and root S concentrations increased significantly with S application, but root nodule numbers and nitrogenase activity were not significantly affected. Organic acid secretion by roots was drastically increased by S application, and shoot P concentration and content also increased significantly. These results indicated that S application can promote P solubilization by increasing the secretion of organic acids by roots and increase in N fixation is not involved in this promotion at least in early vegetative growth period.
1. Introduction
Phosphorus (P) is an essential macronutrient in plants and forms part of important compounds in plant cells, such as nucleic acids, phospholipids, sugar phosphates, and coenzymes. Particularly in legumes, P, in the form of adenosine triphosphate, is needed for nodule formation and N fixation (Ljones Citation1979; Pang et al. Citation2018; Raven Citation2012; Sulieman and Tran Citation2015). However, most P in soils exists in insoluble forms that plants cannot absorb, e.g., P bonded with metals such as aluminum (Al) and iron (Fe) in acid soils or calcium (Ca) in alkaline soils (Sanyal and De Datta Citation1991). Additionally, 20–80% of soil P exists as organic P such as phytic acid, sugar phosphates, nucleic acids, and phospholipids, which are also poorly soluble when bonded with metal ions (Iyamuremye, Dick, and Baham Citation1996). Therefore, the efficiency of P fertilizer use by crops is less than 20%, and farmers tend to apply more P fertilizer to fields than is required by crops. In addition, countries having PO43–rich rocks, which is the raw material of P fertilizer, are unevenly distributed, and P resources are limited globally (Cordell, Drangert, and White Citation2009; Gilbert Citation2009). Therefore, efficient P management is necessary to achieve sustainable agriculture.
Plants have strategies to acquire P under P-deficient conditions such as modifying root architecture to increase root surface area, secretion of organic acids and phosphatases to solubilize poorly soluble P under P-deficient conditions (Hallama et al. Citation2019; Otani, Ae, and Tanaka Citation1996; Zhao and Wu Citation2014; Zhang, Ma, and Cao Citation1997). In the rhizosphere, organic acids release inorganic PO43- from poorly soluble PO43- salts, and phosphatases release inorganic PO43- via hydrolyzation of organic P compounds, then the solubilized PO43- can be absorbed by plants. It is known that plant genotypes or species with efficient P content have high ability to secrete organic acids or phosphatases. For example, white lupine (Lupinus albus) plants show preferable root architecture called as ‘cluster roots’ with high activity to secrete acid phosphatase under conditions of low available P (Wasaki et al. Citation2003). As for pigeon pea (Cajanus cajan), specific organic acids are secreted to solubilize PO43- bond with Fe (Ae et al. Citation1990).
In legumes, rhizobium inoculation can promote the secretion of organic acids by roots and P solubilization in soils. Rhizobium inoculation promoted the exudation of organic acids under P-deficient conditions in common bean (Phaseolus vulgaris; Maureen et al. Citation2011) and enhanced P solubilization in soybean (Glycine max; Qin et al. Citation2011). In addition, rhizobium inoculation in soybean increased the solubilization of Ca–P, after which plants could effectively absorb P (Qin et al. Citation2011).
The application of S promotes symbiosis between legumes and rhizobia. For example, in white clover (Trifolium repens), S application improved plant growth because of increased production of nitrogenase and leghemoglobin and the promotion of nodulation (Varin et al. Citation2010). In alfalfa (Medicago sativa), S application increased the number of nodules and improved nitrogenase activity (Scherer and Lange Citation1996). S application in pea (Pisum sativum) increased the N content, SPAD values, and plant dry weight. Therefore, growth suppression due to S deficiency was caused by N deficiency resulting from decreased N fixation (Zhao, Wood, and McGrath Citation1999). In Lotus japonicus, nodule-specific sulfate transporter was required for proper nodule formation and N fixation (Krusell et al. Citation2005). Furthermore, the structure and activity of nitrogenase protein complex requires S atoms, which is why S is required for N fixation.
As described above, nodulation and N fixation activity could be improved by S application, whereas rhizobium inoculation can promote organic acid exudation and P solubilization, suggesting a relationship between N fixation by rhizobia, S application, and P solubilization. In particular, S application might affect P solubilization by promoting the exudation of organic acids through the improvement of N fixation activity. However, these relationships have not been confirmed. Understanding of the mechanisms of the plant P cycle after rhizobium inoculation and/or S application is necessary for improving P use efficiency in leguminous crops. Thus, the objective of this study was to investigate the effects of S application on organic acid secretion from soybean roots for solubilizing Ca–P in the rhizosphere and to analyze the involvement of soybean–rhizobia symbiosis in Ca–P solubilization.
2. Materials and methods
2.1. Experimental design and plant growth conditions
In our experimental design, three different concentrations of sulfur, 0 µM (S0), 20 µM (S20) or 1000 µM (S1000) were used in the nutrient solutions for soybean. Ca-P was used as a sole phosphorus source, and a rhizobium was inoculated to all plants. A preliminary experiment has been conducted in advance and similar results were observed with the main experiment shown in this manuscript.
Seeds soybean (Glycine max (L.) Merr cv ‘Enrei’) and the rhizobium strain Bradyrhizobium diazoefficiens USDA110 were used in this study. As an insoluble P source, Ca3(PO4)2 was mixed with vermiculite (Yoshino Gypsum Co., Ltd., Tokyo, Japan) at 100 mg P per kilogram vermiculite (Qin et al. Citation2011). An N-free nutrient solution, slightly modified from Tejima et al. (Citation2003), was used: 5 mM CaCl2, 5 mM KCl, 2 mM MgCl2, 34 µM EDTA–FeNa, 5 µM MnCl2, 2 µM ZnCl2, 0.5 µM CuCl2, 0.1 µM NaMoO4, and 25 µM H3BO3. The S concentrations were adjusted by adding Na2SO4 to the N-free nutrient solution at 0, 20, or 1000 µM and applied to plants once every 2–3 days, maintaining the moisture at 0.6 mL/g vermiculite. Plants were cultivated at 10 replicates in each treatment.
Bradyrhizobium diazoefficiens USDA110 was cultured in YMB medium (Jordan Citation1984) on a shaker at 100 rpm and 28°C for 1 week, after which it was washed and diluted with the N-free nutrient solution (S0) which is described above. The seeds were sterilized with NaClO and sown in vermiculite moistured with the N-free solution at 0.6 mL/g vermiculite. Subsequently, diluted B. diazoefficiens USDA110 was dropped on the sown seeds at 1 mL per seed for inoculation. One week after sowing, the seedlings were thinned out so that only one seedling per a pot remained. The plants were grown at 25 ℃ under a 12 h light–dark cycle for 5 weeks. To prevent spindly growth, LED panels were positioned in every direction. Five weeks after sowing, plant samples were harvested to measure the fresh weight of nodules. The samples were oven-dried at 80 ℃ for 48 hours to determine the plant dry weight and other parameters.
2.2. SPAD value measurements
The soil-plant analysis development (SPAD) values were measured using a SPAD meter (SPAD 502 Plus, Konica Minolta, Inc., Tokyo, Japan) at 3, 4, and 5 weeks after sowing. These values were determined at the center of the trifoliate leaves at the third node from the top, and the values were averaged. Measurements were performed with 10 biological replicates in each treatment.
2.3. Nitrogenase activity
Nitrogenase activity was evaluated via acetylene reductase activity (ARA) at 5 weeks after sowing. ARA was measured using a Shimadzu GC-2014 gas chromatograph (Shimadzu Corporation, Kyoto, Japan) equipped with a Porapak N column (Agilent Technologies, Santa Clara, USA). The whole root system of each soybean plant was placed in an incubation bottle (300 mL) with a sealed cap, and 10% (v/v) of the air was replaced with acetylene gas. Samples were incubated at 28°C for 60 min. After incubation, 1 mL of the gas sample was injected into the gas chromatograph (Artigas et al. Citation2020). Measurements were performed with 5 biological replicates in each treatment.
2.4. Plant N content
Shoots and roots were sampled separately at 5 weeks after sowing and oven-dried at 80 ℃ for 48 hours, after which they were powdered using a blender. Twenty milligrams of the dried powders were subjected to measurement of total N and C by NC analyzer (SUMIGRAPH NCTR22, Sumika Chemical Analysis Service Ltd., Tokyo, Japan) according to Miyatake et al. (Citation2019). Measurements were performed with 5 biological replicates in each treatment.
2.5. Plant S content
The dried shoots and roots sampled at 5 weeks after sowing were powdered as described above and digested with HNO3. The total S content was determined via inductively coupled plasma mass spectrometry (Agilent 7800) using 2 ppb In as internal standard (Miyatake et al. Citation2019). Measurements were performed with 5 biological replicates in each treatment.
2.6. Organic acid extraction and analysis
Soybean plants were removed from the vermiculite at 5 weeks after sowing, and their roots were gently washed with tap water and then rinsed with milli-Q water. The roots were soaked in 100 mL milli-Q water for 2 h so that root exudates could be collected from the water, and the extracted solutions were freeze-dried. The freeze-dried extracts were suspended in 2 mL milli-Q water and filtered with a 0.22 µm sterile filter unit (Millex®-GV, MILLIPORE, Cork, Ireland). Organic acids were analyzed using a high-performance liquid chromatography system equipped with a Shimadzu Shim-pack column SCR101N at a column temperature of 40°C. Furthermore, 0.1% (v/v) of H3PO4 was used as the mobile phase in isocratic mode at 0.5 mL/min. The analysis time was 50 min. Standards of organic acids, such as oxalic, citric, tartaric, malonic and succinic acid, were used to determine the concentration of each organic acid in the extracts. Measurement were performed with 4 biological replicates in each treatment.
2.7. Plant P content
Of the dried powder, 100 mg was digested with 2 mL HNO3 and 0.2 mL H2SO4 at 150 ℃ according to Kuboi (Citation1990). The digested samples were mixed with 0.1 N HCl up to 50 mL and subjected to colorimetric analysis via the MoO12S3 method (Murphy and Reley Citation1962). Measurement were performed with 5 biological replicates in each treatment.
2.8. The pH of the vermiculite
The pH of the vermiculite before and after soybean cultivation for 5 weeks was measured. Reverse osmosis (RO) water was applied to the vermiculite at the ratio of water to the vermiculite as 2: 5 and shaken for 10 minutes. The pH of the supernatant was measured with three biological replicates.
2.9. Statistical analysis
All the data were statistically analyzed using IBM SPSS (v. 23.0.0; SPSS, IBM, NY, USA).
3. Results
3.1. Plant growth
SPAD values with treatment S1000 were the highest among S treatments at 3, 4, and 5 weeks after sowing (). These values were significantly higher than those with S20 at 3 weeks as well as those with S0 and S20 at 4 and 5 weeks. From 3 to 4 weeks after sowing, the SPAD values in all S treatments increased, especially with S1000, whereas the values did not increase with all S treatments from 4 to 5 weeks after sowing (). This suggests that plant growth stabilized at 4 to 5 weeks after sowing. Dry weights of shoots and roots at 5 weeks showed no significant differences between the S treatments ().
Figure 1. Changes in SPAD values from 3 to 5 weeks after sowing with 0, 20, or 1000 µM S. Each value was mean of 10 biological replicates with standard error. Different letters on bars indicate significant differences in SPAD value between S treatments according to Tukey test (P < 0.05)
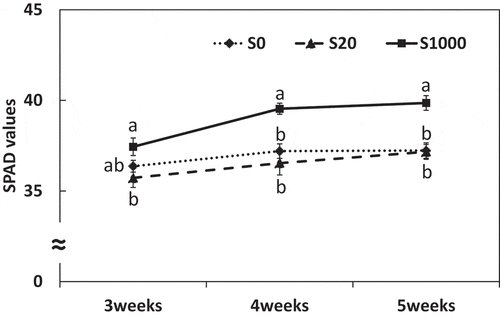
Figure 2. Shoot and root dry weight at 5 weeks after sowing with 0, 20, or 1000 µM S. Each value was mean of 10 biological replicates with standard error. Different letters on bars indicate significant differences between S treatments according to Tukey test (P < 0.05)
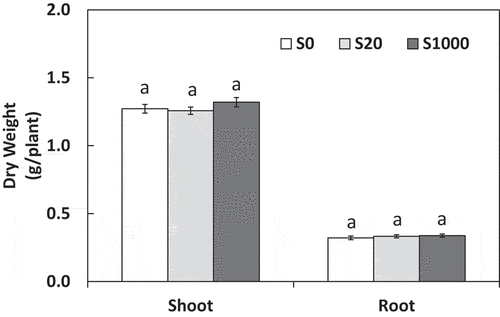
Both root and shoot S concentrations increased significantly with S application, and those with treatment S1000 were significantly higher than those with S0 and S20 ()). Therefore, applied S was assumed to be absorbed and assimilated by plants in this study. Shoot S content increased by 18.7% with S20 and S1000 compared with S0, but this increase was not significant (P = 0.07; ). Root S content significantly increased with S1000 compared with S0 ()).
Figure 3. Shoot and root S concentrations (a) and content (b) at 5 weeks after sowing with 0, 20, or 1000 µM S. Each value was mean of 5 biological replicates with standard error. Different letters on bars indicate significant differences between S treatments according to Tukey test (P < 0.05)
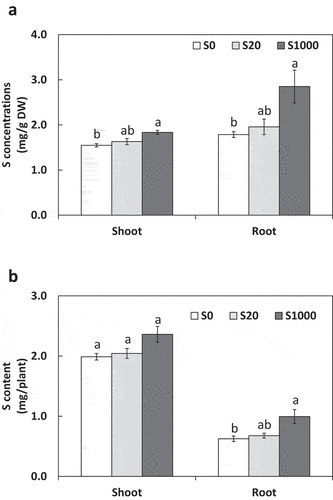
The number of nodules were not significant, likely because the number of nodules varied within the same treatment ()). Nodule weights were not significantly differencnt between S treatments ()).
3.2. Plant nitrogenase activity and N concentrations
ARA was used to evaluate nitrogenase activity via the amount of ethylene produced by acetylene reduction. Neither ARA per nodule fresh nor ARA per plant were significantly different between S treatments (). Shoot and root N concentrations were similar among the S treatments ()). Trends in N content were similar to those in N concentrations ()).
Figure 5. Acetylene reductase activity (ARA) per nodules fresh weight (a) and per plant (b) at 5 weeks after sowing with 0, 20, or 1000 µM S. Each value was mean of 5 biological replicates with standard error. Different letters on bars indicate significant differences between S treatments according to Tukey test (P < 0.05)
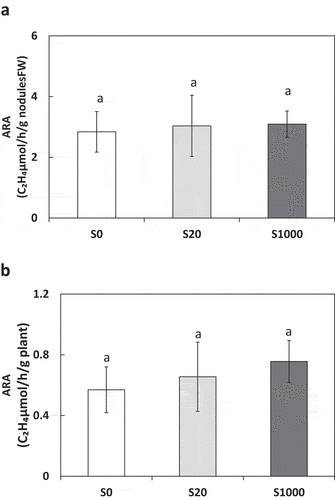
3.3. Effects of S application on rhizosphere P solubilization
Organic acids can improve the solubilization of insoluble soil P. The effects of S on organic acids secreted from soybean roots were examined. All organic acids measured increased with increasing S application (). Oxalic, citric, and malonic acid increased drastically with increasing S application. With treatments S20 and S1000 compared to S0, oxalic acid increased by 397 and 474%, citric acid by 206 and 491%, tartaric acid by 533 and 1019%, malonic acid by 161 and 221%, and succinic acid by 65 and 122%, respectively.
Figure 7. Organic acid concentrations (a) and content (b) secreted from roots 5 weeks after sowing in treatments with 0, 20, or 1000 µM S. Each value was mean of 4 biological replicates with standard error. Different letters on bars indicate significant differences in organic acid content between S treatments according to Tukey test (P < 0.05)
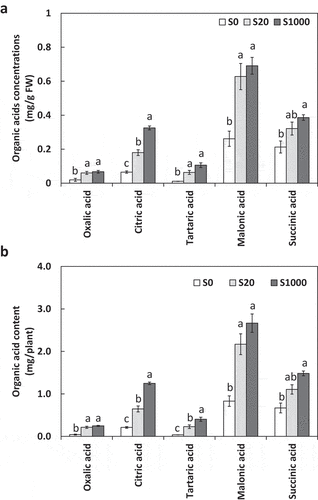
Shoot P concentration increased significantly by 17.3% with S20 and S1000 compared to that with S0 (). In contrast, root P concentrations showed no significant differences between S treatments. Shoot P content per plant with S20 and S1000 increased significantly by 14.7 and 17.6%, respectively, compared with that with S0 (). In contrast, no significant differences were observed in root P content among the S treatments.
3.4. Effects of S application on the pH of the vermiculite before and after cultivation
Before cultivation, the pH of the vermiculite with S0, S20 and S1000 were 7.28, 7.24 and 7.29, respectively. After cultivation, the pH of the vermiculite with S0, S20 and S1000 were 6.44, 6.45 and 6.44, respectively.
4. Discussion
4.1. Effects of S application on plant growth and P solubilization
SPAD values with treatment S1000 were significantly higher than those with S0 and S20, and the differences increased with increasing number of cultivation days (). The SPAD value is a parameter of N content, but since N concentrations were similar in the different S treatments ()), factors other than N likely contributed to increased SPAD values with S1000. For example, shoot S content was higher with S1000 (), and S application is known to increase chlorophyll content (Hawkesford et al. Citation2012).
In our study, organic acids secreted by the roots increased with the addition of S. In particular, citric, tartaric, and malonic acid content increased significantly even with S20 compared to that with S0 (). According to Bolan et al. (Citation1994), the efficiency of P solubilization is in the order of tricarboxylic acid > dicarboxylic acid > monocarboxylic acid. In our study, it is considered that citric acid secreted from roots solubilized P with high efficiency as a tricarboxylic acid, and tartaric and malonic acid also contributed as dicarboxylic acids.
This suggests that the organic acids secreted by roots increased drastically with S addition. Since no previous studies have been conducted on S nutrition affecting organic acid secretion to our knowledge, our findings in this regard are novel. In our study, shoot P concentrations and content were significantly increased by S addition at S20 and S1000 compared to S10, but those in roots were not significantly affected (). This suggested that increased organic acid secretion from roots with S application promoted PO43- solubilization from Ca3(PO4)2, and solubilized PO43- was absorbed by roots and transferred to shoots, where it accumulated. For all organic acids except succinic acid, concentrations and contents were significantly increased with S20 than those with S0, however differences between S20 and S1000 were significant only for citric acid concentration and citric and tartaric acids contents (), which may explain the reason of the significant increase in P concentrations and contents from S0 to S20 with no difference between S20 and S1000 ().
Although increases in shoot P concentration and content by S addition were significant, they were not so remarkable compared to the increase in organic acid secretion. This may be because most of P in shoots at 5 weeks after sowing was covered by supply from seeds. Calculating from P contents as 5.7 g/kg seeds in soybean ‘Enrei’ (Ishimoto et al. Citation2016), the same cultivar with which used in this study, P content in a seed used in this study (weight of one seed was 0.302 g in average) is 1.7 mg/seed. As P content per plant is about 2 mg in shoot and 0.5 mg in root (), more than half of total P contents in plants are covered by supply from seeds, which may have covered the effects of P solubilization by organic acid. Further sduty is necessary to evaluate if plants will be grown for longer than 5 weeks to obtain larger plant biomass, the effects of S addition on P contents in plants will be seen more clearly.
In the present study, S was supplemented as Na2SO4. Thus Na+ ion was also increased accordingly. It is reported that organic acids were decreased by 100 or 150 mM of NaCl in Alfalfa (Fougère,et al. Citation1991), suggesting that organic acids rather decrease under salinity stress. But these concentrations are much higher compared to those used in our study. The Na+ concentrations use in our study were 40 μM with S20 and 2 mM with S1000 treatments, which should not cause salinity stress in plants. Increase in organic acid secretions are also reported by other cations for Al3+, Cu2+ and Zn2+ treatments at μM orders (Li, Ma, and Matsumoto Citation2000; Qin, Hirano, and Brunner Citation2007; Wu et al. Citation2018), and this is for chelating these metals as they are toxic even at low concentrations. There was no report for the effect of Na+ on organic acid secretion at μM order and this level of Na+ is not at al toxic for plants. Thus it is considered that effects on organic acid secretion were caused by the essential element, S, in our study.
4.2. Influence on N fixation and organic acid secretion by S application
Previous studies have reported that S is important for nodulation and N fixation of plants (Krusell et al. Citation2005; Scherer and Lange Citation1996; Varin et al. Citation2010; Zhao, Wood, and McGrath Citation1999). Our results suggested that the number and weight of nodules tended to increase with S application, but no significant effect was observed (). The reason for this may be that seed S content supported physiological function for symbiosis for 5 weeks after sowing. Calculating from seed S contents as 3.2 g/kg seeds in soybean ‘Enrei’ (Ishimoto et al. Citation2016), S content in a seed used in this study is about 0.97 mg/seed. This covers about 40% of the total S contents in plants at 5 weeks after sowing (), which may have been enough for supporting symbiosis. Furthermore, calculating from seed N contents as about 64.4 g/kg seeds in soybean ‘Enrei’ (Ishimoto et al. Citation2016), N content in a seed used in this study is about 19.4 mg/seed. This covers about half or the total N contents in plants at 5 weeks after sowing (), which might have also covered the effects of S application on N contents. In contrast, organic acid secretion increased significantly with S addition and was thus sensitive to S nutrition. Root S concentration with S1000 was also higher than that with the other treatments (), which may have increased organic acid secretion by roots.
S application had little effect on growth, N content, and N fixation activity. Therefore, the secretion of organic acids by soybean was concluded to be suppressed by S deficiency in this study. Furthermore, it is considered that S deficiency causes plants to use carbon within the plant body to maintain their physiological processes rather than secrete carbon compounds. Since organic acids were released with S application in this study, soybean likely only secretes organic acids under suitable S levels.
4.3. Relationship between P solubilization and soil pH
Kaplan and Orman S (Citation2008) suggested that elemental S application decreased the soil pH of calcareous alkaline soil, and, consequently, the dry weight and P absorption increased in bean (Phaseolus Vulgaris). Similarly, in sorghum (Sorghum bicolor), the absorption of P, Fe, Zn, Mn, and Cu increased with increasing elemental S application in calcareous alkaline soils (Kaplan and Orman Citation1998). Kaya et al. (Citation2020) showed that the growth of maize in calcareous soil was improved by S-enriched soil amendments under P-deficient conditions, and this improvement was concomitant with increased plant antioxidative activity. These reports suggested that pH improvement via elemental S application or S-enriched amendments increased the micronutrient and P solubility, which promoted plant growth. In contrast, the present study did not use alkaline soils, and the pH of the vermiculite was almost neutral with or without S application. Actually, the pH of the vermiculite determined were similar between S treatment both at before and after cultivation. This is considered to be because the hydroponic solutions with the same pH was given once every 2 to 3 days during cultivation. Therefore, increased P absorption with S application in this study was likely caused by a different mechanism than pH changes.
5. Conclusion
Organic acid secretion is important for plants to solubilize P in soils. The synthesis and secretion of organic acids are known to be promoted under P- or Fe-deficient conditions, but the relationship between S and organic acids has hitherto been unknown. The present study revealed that S nutrition affected the solubilization of Ca–P by drastically affecting the secretion of organic acids, especially citric and malonic acid. To our knowledge, this is the first study reporting that S application affect organic acid secretion which may be able to solubilize phosphorus in the rhizosphere. Our study concluded that, under S-deficient conditions, attention should be paid not only to S application for plant growth but also the acquisition of P, and S application has a potential to improve solubilization P fixed in rhizosphere soils for its acquisition by plants. In vermiculite, S application did not significantly increase rhizobium symbiosis in early vegetative growth period. Symbiosis with rhizobia may be involved in P solubilization with S application depending on soil conditions or soybean growth periods.
Disclosure statement
The authors declare that they have no known competing financial interests or personal relationships that could have appeared to influence the work reported in this paper.
Additional information
Funding
References
- Ae, N., J. Arihara, K. Okada, T. Yoshihara, and C. Johansen. 1990. “Phosphorus Uptake by Pigeon Pea and Its Role in Cropping Systems of the Indian Subcontinent.” Science 248 (4954): 477–480. doi:10.1126/science.248.4954.477.
- Artigas, R. M. D., M. Espana, S. Lewandowska, K. Yuan, S. Okazaki, N. Ohkama-Ohtsu, and T. Yokoyama. 2020. “Phylogenetic Analysis of Symbiotic Bacteria Associated with Two Vigna Species under Different Agro-Ecological Conditions in Venezuela.” Microbes and Environments 35: 1. doi:10.1264/jsme2.ME19120.
- Bolan, N. S., R. Naidu, S. Mahimairaja, and S. Baskaran. 1994. “Influence of Low-molecular-weight organic Acids on the Solubilization of Phosphates.” Biology and Fertility of Soils 18 (4): 311–319. doi:10.1007/BF00570634.
- Cordell, D., J.-O. Drangert, and S. White. 2009. “The Story of Phosphorus: Global Food Security and Food for Thought.” Global Environmental Change-Human and Policy Dimensions 19 (2): 292–305. doi:10.1016/j.gloenvcha.2008.10.009.
- Fougere, F., D. Lerudulier, and J. G. Streeter. 1991. “Effects of Salt Stress on Amino-Acid, Organic-Acid, and Carbohydrate-Composition of Roots, Bacteroids, and Cytosol of Alfalfa (Medicago-Sativa L).„ Plant Physiology 96 (4): 1228–36. doi:10.1104/Pp.96.4.1228.
- Gilbert, N. 2009. “Environment: The Disappearing Nutrient.” Nature 461 (7267): 1041. doi:10.1038/461716a.
- Hallama, M., C. Pekrun, H. Lambers, and E. Kandeler. 2019. “Hidden Miners - the Roles of Cover Crops and Soil Microorganisms in Phosphorus Cycling through Agroecosystems.” Plant and Soil 434 (1–2): 7–45. doi:10.1007/s11104-018-3810-7.
- Hawkesford, M., W. Horst, T. Kichey, H. Lambers, J. Schjoerring, I. S. Møller, and P. White. 2012. “Functions of Macronutrients.” In Marschner’s Mineral Nutrition of Higher Plants3rd, P. Marschneredited by, Pergamon, UK:135–189, Academic press. doi:10.1016/B978-0-12-384905-2.00006-6
- Ishimoto, M., Y. Yokota, K. Takagi, and A. Kaga. 2016. “Analysis of Genetic Factors Associated with the Contents of Soybean Seed Components in a Controlled Environment.” (in Japanese with English Summary). Soy Protein Research, Japan 19: 24–29. https://www.fujifoundation.or.jp/search/pdf/037/37_05.pdf
- Iyamuremye, F., R. P. Dick, and J. Baham. 1996. “ORGANIC AMENDMENTS AND PHOSPHORUS DYNAMICS: I. PHOSPHORUS CHEMISTRY AND SORPTION.” Soil Science 161 (7): 426–435. doi:10.1097/00010694-199607000-00002.
- Jordan, D. C. 1984. “Rhizobiaceae.” In Bergey’s Manual of Systematic Bacteriology, Vol. 1, edited by N. R. Krieg and J. G. Holt, 234–244. Baltimore: Williams and Wilkins.
- Kaplan, M., and Ş. Orman. 1998. “Effect of Elemental Sulphur and Sulphur Containing Waste in a Calcareous Soil in Turkey.” Journal of Plant Nutrition 21 (8): 1655–1665. doi:10.1080/01904169809365511.
- Kaplan, M. and S, Orman. 2008. “Effect of Elemental sulphur and sulphur Containing Waste in a Calcareous Soil in Turkey. Journal of plant nutrition 21, 1655-1665. doi:10.1080/01904169809365511
- Kaya, C., M. Senbayram, N. A. Akram, M. Ashraf, M. N. Alyemeni, and P. Ahmad. 2020. „Sulfur-enriched Leonardite and Humic Acid Soil Amendments Enhance Tolerance to Drought and Phosphorus Deficiency Stress in maize (Zea mays L.).“ Scientific Reports 10 (1). doi:10.1038/S41598-020-62669-6
- Krusell, L., K. Krause, T. Ott, G. Desbrosses, U. Kramer, S. Sato, Y. Nakamura, et al. 2005. “The Sulfate Transporter SST1 Is Crucial for Symbiotic Nitrogen Fixation in Lotus Japonicus Root Nodules.” The Plant Cell 17 (5): 1625–1636. doi:10.1105/tpc.104.030106.
- Kuboi, T. 1990. “Ashing: 4. Digestion by Nitric Acid - Sulfuric Acid.” In Japanese Society of Soil Science and Plant Nutrition (Ed) Experiment Methods for Plant Nutrition, 126. Hakuyusha, Tokyo.
- Li, X. F., J. F. Ma, and H. Matsumoto. 2000. “Pattern of Aluminum-induced Secretion of Organic Acids Differs between Rye and Wheat.” Plant Physiology 123 (4): 1537–1544. doi:10.1104/pp.123.4.1537.
- Ljones, T. 1979. “Nitrogen Fixation and Bioenergetics: The Role of Atp in Nitrogenase Catalysis.” FEBS Letters 17 (5): 1–8. doi:10.1016/0014-5793(79)80138-6
- Maureen, F., Roseline, R., Jans, M., Appolinaire, T., and L. Eddy. 2011. “Inoculation with Rhizobium etli enhances organic acid exudation in common bean (Phaseolus vulgaris L.) subjected to phosphorus deficiency.„ African Journal of Agricultural Research 6: 2235-2242. doi:10.1093/jxb/erp303.
- Miyatake, M., T. Ohyama, T. Yokoyama, S. Sugihara, T. Motobayashi, T. Kamiya, T. Fujiwara, K. Yuan, S. D. Bellingrath-Kimura, and N. Ohkama-Ohtsu. 2019. “Effects of Deep Placement of Controlled-Release Nitrogen Fertilizer on Soybean Growth and Yield under Sulfur Deficiency.” Soil Science and Plant Nutrition 65 (3): 259–266. doi:10.1080/00380768.2019.1615827.
- Murphy, J. and J. Reley. 1962 “A Modified Single Solution Method for the Determination of Phosphate in Natural Waters.„Anal. Chimica Chim Acta 27: 31–36. doi:10.1016/S0003–2670(00)88444–5
- Murphy, J., and J. P. Riley. 1986. “Citation-Classic - a Modified Single Solution Method for the Determination of Phosphate in Natural-Waters.” Current Contents/Agriculture Biology & Environmental Sciences 12: 16-16.
- Otani, T., N. Ae, and H. Tanaka. 1996. “Phosphorus (P) Uptake Mechanisms of Crops Grown in Soils with Low P Status .2. Significance of Organic Acids in Root Exudates of Pigeonpea.” Soil Science and Plant Nutrition 42 (3): 553–560. doi:10.1080/00380768.1996.10416324.
- Pang, J., M. H. Ryan, H. Lambers, and K. H. Siddique. 2018. “Phosphorus Acquisition and Utilisation in Crop Legumes under Global Change.” Current Opinion in Plant Biology 45 (Pt B): 248–254. doi:10.1016/j.pbi.2018.05.012.
- Qin, L., H. Jiang, J. Tian, J. Zhao, and H. Liao. 2011. “Rhizobia Enhance Acquisition of Phosphorus from Different Sources by Soybean Plants.” Plant and Soil 349 (1–2): 25–36. doi:10.1007/s11104-011-0947-z.
- Qin, R. J., Y. Hirano, and I. Brunner. 2007. “Exudation of Organic Acid Anions from Poplar Roots after Exposure to Al, Cu and Zn.” Tree Physiology 27 (2): 313–320. doi:10.1093/treephys/27.2.313.
- Raven, J. A. 2012. “Protein Turnover and Plant RNA and Phosphorus Requirements in Relation to Nitrogen Fixation.” Plant Science 188-189: 25–35. doi:10.1016/j.plantsci.2012.02.010.
- Sanyal, S. K., and S. K. De Datta. 1991. “Chemistry of Phosphorus Transformations in Soil.” In Advances in Soil Science, edited by B. A. Stewart, 1–120. New York: Springer.
- Scherer, H. W., and A. Lange. 1996. “N2 Fixation and Growth of Legumes as Affected by Sulphur Fertilization.” Biology and Fertility of Soils 23 (4): 449–453. doi:10.1007/BF00335921.
- Sulieman, S., and L.-S. P. Tran. 2015. “Phosphorus Homeostasis in Legume Nodules as an Adaptive Strategy to Phosphorus Deficiency.” Plant Science 239: 36–43. doi:10.1016/j.plantsci.2015.06.018.
- Tejima, K., Y. Arima, T. Yokoyama, and H. Sekimoto. 2003. “Composition of Amino Acids, Organic Acids, and Sugars in the Peribacteroid Space of Soybean Root Nodules.” Soil Science and Plant Nutrition 49 (2): 239–247. doi:10.1080/00380768.2003.10410003.
- Varin, S., J.-B. Cliquet, E. Personeni, J.-C. Avice, and S. Lemauviel-Lavenant. 2010. “How Does Sulphur Availability Modify N Acquisition of White Clover (Trifolium Repens L.)?” Journal of Experimental Botany 61 (1): 225–234. doi:10.1093/jxb/erp303.
- Wasaki, J., T. Yamamura, T. Shinano, and M. Osaki. 2003. “Secreted Acid Phosphatase Is Expressed in Cluster Roots of Lupin in Response to Phosphorus Deficiency.” Plant and Soil 248 (1–2): 129–136. doi:10.1023/A1022332320384.
- Wu, L. J., Y. Kobayashi, J. Wasaki, and H. Koyama. 2018. “Organic Acid Excretion from Roots: A Plant Mechanism for Enhancing Phosphorus Acquisition, Enhancing Aluminum Tolerance, and Recruiting Beneficial Rhizobacteria.” Soil Science and Plant Nutrition 64 (6): 697–704. doi:10.1080/00380768.2018.1537093.
- Zhang, F. S., J. Ma, and Y. P. Cao. 1997. “Phosphorus Deficiency Enhances Root Exudation of Low-Molecular Weight Organic Acids and Utilization of Sparingly Soluble Inorganic Phosphates by Radish (Raghanus Sativus L.) And Rape (Brassica Napus L.) Plants.” Plant and Soil 196 (2): 261–264. doi:10.1023/A:1004214410785.
- Zhao, F. J., A. P. Wood, and S. P. McGrath. 1999. “Effects of Sulphur Nutrition on Growth and Nitrogen Fixation of Pea (Pisum Sativum L.).” Plant and Soil 212 (2): 207–217. doi:10.1023/A:1004618303445.
- Zhao, K., and Y. Y. Wu. 2014. “Rhizosphere Calcareous Soil P-extraction at the Expense of Organic Carbon from Root-exuded Organic Acids Induced by Phosphorus Deficiency in Several Plant Species.” Soil Science and Plant Nutrition 60 (5): 640–650. doi:10.1080/00380768.2014.934191.